TEVAR for Thoracoabdominal Aneurysm Repair
Gustavo S. Oderich
Tiziano Tallarita
Introduction
Endovascular aortic aneurysm repair (EVAR) has gained widespread acceptance. Three years after the pioneer work by Juan Parodi and colleagues in 1991, Michael Dake and associates expanded the applications of endovascular stent grafts to treat thoracic aortic aneurysms. Prospective randomized studies have shown several short-term advantages for EVAR, including less blood loss and transfusion requirements, shorter hospital stay, and lower morbidity and mortality. The potential benefit of a minimally invasive approach is greater when this technique is applied to the thoracic or thoracoabdominal aorta.
Open repair of thoracic and thoracoabdominal aortic aneurysms (TAAAs) remains a formidable challenge. Exposure requires thoracotomy or thoracoabdominal incision, single lung ventilation, and several adjuncts are used to protect the spinal cord, kidneys, and gastrointestinal organs. Despite refinements in organ protection using combinations of cardiopulmonary or left heart bypass, deep hypothermia, cerebrospinal fluid drainage, and selective visceral artery perfusion, spinal cord injury, and dialysis remain vexing complications of these procedures. Contemporary series have shown that open repair of thoracic and TAAAs can be performed with acceptable results in centers of excellence. Coselli and associates reported 2,286 patients treated by open TAAA repair, with an operative mortality of 6.6% and spinal cord injury in 4%. Other reports from large-volume aortic centers have shown mortality rates in the range of 4.6% to 14.6%. However, “real-world” data using national and regional datasets have demonstrated more ominous results. In the study by Rigberg and associates on 797 Medicare beneficiaries who underwent elective open TAAA repair in the State of California, the mortality was 19% at 30 day and 31% in 1 year.
Since the original report by Michael Dake and associates, thoracic endovascular repair evolved from homemade to manufactured stent grafts. The GORE TAG endoprosthesis (WL Gore & Associates, Inc., Flagstaff, AZ) became the first thoracic stent graft approved in the United States in 2005. Several multicenter prospective nonrandomized pivotal trials have
shown the safety and efficacy of these devices, establishing TEVAR as the first line of treatment for thoracic aneurysms.
shown the safety and efficacy of these devices, establishing TEVAR as the first line of treatment for thoracic aneurysms.
Anatomical factors have limited the application of endovascular approaches beyond the abdominal and thoracic aorta. In addition to the limitation of inadequete landing zones because of side branch involvement, other challenges included difficult access, angulation, tortuous anatomy, and discrepancy in diameter. For TAAAs, involvement of the visceral segment of the aorta posed a major challenge. In 2001, Timothy Chuter and associates introduced the concept of multiple directional branches to treat TAAAs. This was followed by significant progress in fenestrated stent-graft techniques by the group from Western Australia and Roy Greenberg at the Clevelend Clinic. During the last decade, fenestrated and branched stent grafts have been widely applied to treat arch, thoracoabdominal, pararenal, or aortoiliac aneurysms. Although these devices have been widely utilizied worldwide with >9,000 implants (Cook Medical, personal communication), its access has been limited in the United States to a few centers who have physician-sponsored Investigational Device Exemption (IDE) protocols. This chapter summarizes basic concepts of endovascular treatment of thoracic and TAAAs.

The indication for repair should take into consideration a risk–benefit analysis. The risk of rupture should be analyzed in contrast to the risk of perioperative death or major disability, including paraplegia, stroke, or dialysis. Treatment is recommended in the symptomatic patient independent of size criteria. In general, for thoracic and TAAAs a minimum size of 5.5 to 6 cm is recommended to indicate repair. Other well-accepted indication is rapid aneurysm enlargement, defined as >5 mm growth in 6 months. Saccular aneurysms are more unpredictable and warrant repair at smaller size diameter.
The threshold to recommend repair may be increased in the elderly patient with comorbidities, or lowered in the younger patient or in those with rapid aneurysm enlargement or connective tissue disorders. The annual risk of rupture for a >6-cm thoracic aortic aneuryms is approximately 10% to 15%, increasing to 20% to 30% for aneurysms >7 cm. Spinal cord ischemia remains the most dreaded complication. Rates are dependent upon extent of aortic coverage, spinal cord collaterals, status of the vertebral and internal iliac arteries, and prior aortic repair. In general, rates of spinal cord injury average from <1% for coverage of the proximal thoracic aorta to 3% to 8% for coverage of the entire thoracic aorta. The highest rate occurs for type II TAAA, ranging from 5% to 20%. Recent reports indicate that staging the procedure and preservation of collateral networks reduce rates of permanent spine cord injury.
Although the most common etiology remains degenerative aneurysms, endovascular repair has been increasingly utilized to treat acute and chronic dissections, intramural hematomas (IMHs), penetrating aortic ulcers (PAU), blunt aortic injuries, and aortic coarctation. For chronic dissections, aortic diameter >5.5 to 6 cm remains the most common indication. The same recommendations for degenerative aneurysms are used for chronic dissections. Acute type B dissections have been traditionally treated medically; endovascular repair was reserved for complicated dissection, rupture, or failed medical therapy. Recent evidence from the INSTEAD XL trial and the IRAD registry has challenged this recommendation. In the INSTEAD XL trial, early endovascular repair combined with medical therapy was associated with favorable aortic remodeling, lower rates of aneurysm degeneration, and lower mortality from aortic-related causes. These have led several centers to broaden the indications for endovascular repair in asymptomatic or uncomplicated cases. The author continues to recommend selective treatment in these patients, but has broadened the indications to include patients with refractory pain, difficult to control hypertension, severely collapsed true lumen with preferential false lumen perfusion, aortic enlargement to >4 cm, and false lumen expansion to >2 cm. Timing of TEVAR is also a topic of controversy; the authors’ preference
is for early observation and repair >4 weeks after the event in selected as an uncomplicated case.
is for early observation and repair >4 weeks after the event in selected as an uncomplicated case.
Table 29.1 Genetic Testing in Patients with Aortic Dissection and Aneurysms | ||||||||||||||||||||||||||||||||||||
---|---|---|---|---|---|---|---|---|---|---|---|---|---|---|---|---|---|---|---|---|---|---|---|---|---|---|---|---|---|---|---|---|---|---|---|---|
|
Aortic coarctation in the adult has also been treated with stent grafts in patients who have claudication, difficult to control hypertension, or evidence of end-organ damage, such as left heart strain. For blunt aortic injury, TEVAR is widely accepted in patients with Grades 2 to 4 injury, while a conservative approach is recommended for Grade 1 injury. The use of stent grafts in infected fields remains highly controversial, but TEVAR has been used in patients with aortoesophageal fistula as a bridge to definitive open repair. Because the infection needs to be eradicated, late results with stent grafts placed in the infected field are poor and this cannot be recommended as definitive treatment.
Indications for treatment of uncomplicated IMH remain somewhat controversial. Most experts recommend repair for acute IMH of the ascending aorta (type A), and aggressive medical therapy for IMH in the descending aorta (type B), similar to type B aortic dissection. Indications for repair are complicated, progressive IMH, intractable symptoms, recurrent IMH, and diameter >1 cm. PAU can occur in association with IMH or as isolated incidental finding on imaging studies. Indications for repair are symptomatic ulcers or signs of deep erosion, which predisposes to dissection or rupture.
Endovascular treatment has not been accepted as first-line treatment in patients with connective tissue disorders because of concerns relative to higher rates of procedural complications (e.g., retrograde dissection, endoleak, disruption) and long-term durability. Because the aortic wall is inherently diseased, it is logical to expect progression of aortic disease and loss of seal. One exception is placement of the stent grafts into prior surgical grafts, which provide excellent landing zone. The most frequent genetic disorders associated with aortic-related complications are Marfans syndrome, Loyes–Dietz syndrome, Vascular Ehlers–Danlos syndrome, and familial thoracic aneurysm and dissection syndromes (Table 29.1). A medical genetics evaluation is recommended in patients with clinical features suggestive of these disorders, in younger patients (<50 years old) who present with aortic aneurysm or dissection, and in those who have first-degree relatives with aortic pathology.
Anatomical Considerations
Preoperative imaging is a critical component to plan these procedures. In most centers, computed tomography angiography (CTA) is the preferred imaging modality to plan the operation; less frequently, magnetic resonance angiography (MRA) can also be used. Several of the important anatomical details are outlined below.
Iliac Access
Endovascular thoracic and TAAA repair is most often performed using access via one or both iliofemoral arteries. For complex procedures involving fenestrated and branched
endografts, brachial access is also needed. Difficulties in gaining access remain an obstacle to TEVAR. Most problems can be anticipated based on review of preoperative imaging. An adequate CTA study should evaluate the abdominal aorta, iliac arteries, and common femoral arteries distal to the bifurcation. Presence of small iliac artery diameter (<8 mm), severe calcification, stenosis or occlusion, excessive tortuosity, or dissection may preclude safe introduction of large delivery systems (>20 Fr), which is needed for most commercially available thoracic and TAAA endoprosthesis using conventional femoral approach.
endografts, brachial access is also needed. Difficulties in gaining access remain an obstacle to TEVAR. Most problems can be anticipated based on review of preoperative imaging. An adequate CTA study should evaluate the abdominal aorta, iliac arteries, and common femoral arteries distal to the bifurcation. Presence of small iliac artery diameter (<8 mm), severe calcification, stenosis or occlusion, excessive tortuosity, or dissection may preclude safe introduction of large delivery systems (>20 Fr), which is needed for most commercially available thoracic and TAAA endoprosthesis using conventional femoral approach.
Selection of the ideal access is individualized and takes into consideration iliac artery diameter, occlusive disease, tortuosity, prior stents, or surgical grafts. For most operators, right iliac artery is favored, but these procedures can be safely performed through either approach. The contralateral femoral is accessed using a small 5- or 6-Fr sheath for introduction of angiography catheters for diagnostic purposes. TAAA repair requires bilateral femoral access for placement of renal fenestrated-branches and a distal bifurcated device.
If difficult access is encountered or anticipated, several maneuvers are recommended to avoid iliac artery disruption. Open surgical or endovascular conduits are required in approximately 10% to 15% of patients. Open surgical conduits are performed either prior to the procedure (permanent iliofemoral conduit) or in conjunction with the endovascular repair. A staged permanent conduit should be considered in patients requiring TAAA repair with fenestrated and branched endografts because of the coagulopathy that frequently occurs following these procedures; it should also be considered in patients who have aortic disease affecting multiple segments, where multiple repairs are anticipated. These procedures are performed using a lower abdominal curvilinear incision to enter the retroperitoneum. Blunt dissection is carried over the psoas muscle and iliac crest, exposing the common, internal, and external iliac arteries. After these vessels are controlled with sylastic vessel loopes and the patient is heparinized, a longitudinal arteriotomy is made from the distal common iliac artery to the external iliac artery. The authors’ preference is to use a 12-mm polyester graft, anastomosed in an end-to-side fashion using 4-0 prolene suture with “parachute technique.” This graft diameter allows adequate room for introduction of 24-Fr delivery system with no difficulty. If a permanent conduit is planed, the authors’ preference is to bevel the external iliac artery and anastomose the graft end-to-end carrying the anastomosis to the distal common iliac artery. The distal anastomosis is done to the proximal common femoral artery under the inguinal ligament via a separate longitudinal groin incision.
The endoconduit technique is also an excellent alternative in patients with occlusion of the ipisilateral internal iliac artery. Because this technique requires coverage of the internal iliac artery, we do not recommend its use in patients with patent internal iliac arteries who need coverage of extensive thoracic or TAAA segments. The endoconduit or “paving and crack” technique applies use of small profile peripheral stent grafts or covered stents from the common iliac artery to the distal external iliac artery, with subsequent forceful dilatation to enlarge the vessel to 10 or 12 mm. It is critical that adequate proximal and distal seal is achieved to avoid uncontrolled arterial bleeding.
Brachial access is needed routinely for most TAAAs using a 10- or 12-Fr sheath (Fig. 29.1). The authors’ preference is to perform ultrasound of the proximal brachial artery just distal to the axillary hairline. If the brachial artery measures >4 mm in diameter, the vessel is surgically exposed for direct access. If smaller, the axillary artery is exposed in the delto-pectoral groove or via infraclavicular incision. Branch incorporation with directional branches is typically done via the brachial artery using a coaxial system with 12-Fr sheath and either a 9-Fr sheath (Fluency stent graft) or 7-Fr sheath (Viabahn or iCAST stent graft). For these cases the patient is positioned with the left arm abducted (Fig. 29.1), which facilitates access and minimizes radiation exposure to the operator, who can work away from the imaging intensifier. Alternatively, in patients where only one branch is performed via brachial access using a 7 or 8 Fr sheath, the distal brachial artery can be accessed at the antecubital crease. The authors’ preference is to routinely surgically expose the brachial artery for repair, eliminating risk of puncture hematoma, and its associated nerve injury.
Selection of Sealing Zones
The presence of a healthy proximal and distal sealing zone is of paramount importance to achieve successful and durable aneurysm exclusion. The proximal and distal landing zones must be selected in “normal aortic segments,” defined by parallel aortic wall, with minimal or no thrombus or calcification. The minimum length is >2 cm. Longer lengths are needed in tortuous segments such as in the aortic arch, or in patients with familial history of aortic disease, ectasia or thrombus laden aorta. Because it is critical to assure 360-degree apposition between the stent-graft fabric and aortic wall, this requirement is not compromised to achieve a durable result. One should not be tempted by acute successes, which are more often tempered by late endoleaks in patients with difficult anatomy who do not meet basic anatomical requirements. For this reason, if there is not an adequate sealing zone in the distal thoracic aorta or arch, other techniques of branch vessel incorporation (debranching, branched, or parallel grafts) should be contemplated.
Aortic Side Branch Involvement
The most common challenges include involvement of the distal aortic arch or visceral arteries. Options for extension of repair include surgical debranching, fenestrated and branched endografts, and parallel stent-graft techniques.
Arch Involvement
The aortic arch has been divided into four zones proposed by Ishimaru, based on the location of the landing zone in relation to supra-aortic trunks. One should not underestimate length of sealing zone if the intended stent grafts will be placed in zones 0 to 3. In these cases, one should plan for additional length for optimal apposition of the stent graft in the innercurvature of the aortic arch. Although recent technologic improvements in device conformability have facilitated these repairs, there are many challenges in the arch; to name a few, proximity to the aortic valve, acute angulation, supra-aortic trunks, hemodynamic forces, and respiratory motion.
Open surgical debranching has been widely applied in patients with aneurysms encroaching the carotid or subclavian arteries. Although coverage of the subclavian artery has been advocated by some, most agree that patients with extensive thoracic and thoracoabdominal aortic aneurysms need subclavian artery revascularization to minimize rate of spinal cord injury. The authors’ preference is to use a carotid–subclavian bypass or transposition done in single stage combined with stent-graft placement. If the intended
landing zone is in zone 1, a carotid–carotid bypass and carotid–subclavian bypass can be done. Description of arch branch devices is beyond the scope of this chapter, but these devices are already available and will limit the need for debranching procedures. Finally, for proximal arch involvement requiring placement of stent graft in zone 0, a decision is made between the options of aortic-based arch debranching or conventional arch replacement with frozen elephant trunk technique. The later provides an excellent landing zone for endograft placement.
landing zone is in zone 1, a carotid–carotid bypass and carotid–subclavian bypass can be done. Description of arch branch devices is beyond the scope of this chapter, but these devices are already available and will limit the need for debranching procedures. Finally, for proximal arch involvement requiring placement of stent graft in zone 0, a decision is made between the options of aortic-based arch debranching or conventional arch replacement with frozen elephant trunk technique. The later provides an excellent landing zone for endograft placement.
Visceral Artery Involvement
Extension of the aneurysm into the visceral segment defines TAAA pathology and therefore requires incorporation of the celiac axis, superior mesenteric artery (SMA) and renal arteries. A more detailed analysis with centerline of flow and axial measurements is used for accurate sizing. Although limited repairs with one or two vessels (e.g., celiac and SMA) can be planned, more often a four-vessel repair is recommended with extension of the landing zone to the infrarenal segment. Aortic side branches requiring incorporation should also be analyzed for presence of occlusive disease, excessive calcification or thrombus, unusual or aberrant anatomy. Small sized, calcified, or multiple renal arteries pose a challenge. If an endovascular technique is selected to incorporate a side branch, special attention should be taken to side branch anatomy, vessel tortuosity, adjacent thrombus, or occlusive disease. Independent of which technique is utilized to treat the visceral artery, anatomical factors may significantly impact technical difficulties and method of reconstruction.
Clinical Risk Evaluation
A comprehensive evaluation of cardiac, pulmonary, and renal performance is crucial to optimize patient selection. These operations are often indicated in the sickest patient, but clinical data suggests that prohibitively high-risk patients and those with limited life expectancy are not ideal candidates for these procedures. The evaluation should include noninvasive cardiac stress test (Dobutamine Stress Echocardiogram or Sestamibi study), pulmonary function tests, and carotid ultrasound. Factors associated with increased risk include unstable angina, symptomatic or poorly controlled ectopy, recurrent CHF, ejection fraction <25%, myocardial infarction <6 months, vital capacity <1.8 L, FEV1 <800 mL, DLCO <30%, resting pO2 <60 mm Hg and pCO2 >50 mm Hg, and serum creatinine >2.5 mg/dL.
Medical Genetics Evaluation
Medical genetic evaluation should be strongly considered in the younger patient or in those with phenotypic features suggestive of a disorder or familial history of aortic disease (Table 29.1). These disorders are autosomal dominant and are strongest in the younger patient population affected by aortic dissection or aneurysm. Approximately 20% of patients have a genetic disorder or altered connective tissue. Clinically relevant disorders include Marfans syndrome, Turner syndrome, Vascular Ehlers–Danlos syndrome, and Loeys–Dietz syndrome. The most common mutations are the fibrillin gene (FBN1) or the TGF-receptor 2 gene (TGFBR2) in Marfan and Loyes–Dietz syndromes, respectively. The most common nonsyndromic mutation associated with thoracic aneurysms and dissections is in the SMC actin gene (ACTA2).
Available Devices
Thoracic Aneurysms
Significant improvements in thoracic stent-graft technology have been made in the last decade with respect to delivery system, profile, diameter range, and conformability. In the United States, there are currently five first-generation and one second-generation device available for commercial use. Each endograft has completed a prospective multicenter
nonrandomized regulatory trial. Devices include WL Gore (Flagstaff, AZ) TAG and C-TAG, Cook (Bloomington, IN) TX2, Medtronic (Santa Rosa, CA) Talent and Valiant and Bolton (Sunrise, FL) Relay. The recommended instructions for use (IFU) are fairly similar for these devices, and include suitable access and anatomy with minimum proximal and distal landing zones >2 cm. Table 29.2 summarizes the main device characteristics.
nonrandomized regulatory trial. Devices include WL Gore (Flagstaff, AZ) TAG and C-TAG, Cook (Bloomington, IN) TX2, Medtronic (Santa Rosa, CA) Talent and Valiant and Bolton (Sunrise, FL) Relay. The recommended instructions for use (IFU) are fairly similar for these devices, and include suitable access and anatomy with minimum proximal and distal landing zones >2 cm. Table 29.2 summarizes the main device characteristics.
Table 29.2 Thoracic Aortic Stent Grafts | |||||||||||||||||||||||||||||||||||||||||||||
---|---|---|---|---|---|---|---|---|---|---|---|---|---|---|---|---|---|---|---|---|---|---|---|---|---|---|---|---|---|---|---|---|---|---|---|---|---|---|---|---|---|---|---|---|---|
|
Thoracoabdominal Aneurysms
Visceral Debranching
Hybrid approach or surgical debranching was introduced as a less-invasive alternative to open conventional repair. The first report was by Quinones–Baldrich and associates from UCLA in 1999. The operation aimed to reduce the anatomic and physiologic stress to the patient by avoiding several shortcomings of open surgery: Thoracotomy, single lung ventilation, aortic cross clamping, and prolonged end-organ ischemia. The early enthusiasm with surgical debranching has been tempered by several reports, which demonstrated significant risk of mortality and morbidity, particular when hybrid procedures are indicated in elderly patients with high surgical risk. Therefore, its current role in the treatment of patients with complex aortic aneurysms has evolved, and most centers with easy access to fenestrated and branched endografts have relegated hybrid procedures to high-risk patients who are neither candidates for total endovascular repair or open surgery. Patient selection, case planning, and technical aspects of the procedure are key for successful outcomes. If debranching is considered, the quality of the inflow site, which is typically located in the distal common and proximal external iliac artery, should be reviewed for presence of occlusive disease. It is critical to assure optimal inflow to the visceral grafts and enough length within the common iliac artery for attachment of the endografts. The presence of any abnormal venous anatomy (e.g., left-sided vena cava, retroaortic renal vein, etc.) should be noted to avoid inadvertent injury. For debranching procedures, thoracic and abdominal stent grafts are utilized for the endovascular portion of the procedure.
Parallel Stent Grafts
Total endovascular incorporation can be done using either parallel stent grafts or fenestrated and branched stent grafts. Snorkel and chimney grafts have been used in select centers, but endoleak rates are higher in systematic reviews, approaching 5% to 10%. The parallel stent graft is constructed using either a balloon-expandable or self-expandable stent, preferentially
a covered stent, placed between the aortic wall and the main aortic stent graft to preserve flow into the target vessel. Variations of this technique depending on stent configuration have been named periscope, sandwich, snorkel, and chimney grafts. Long-term result is still lacking and concern remains about the fate of “gutter” endoleaks and the durability of longer branches (e.g., “sandwich grafts”), which are often required in patients with TAAAs. These techniques have a role in the emergency setting or as a bail out in the event of inadvertent side branch coverage during conventional endovascular repair.
a covered stent, placed between the aortic wall and the main aortic stent graft to preserve flow into the target vessel. Variations of this technique depending on stent configuration have been named periscope, sandwich, snorkel, and chimney grafts. Long-term result is still lacking and concern remains about the fate of “gutter” endoleaks and the durability of longer branches (e.g., “sandwich grafts”), which are often required in patients with TAAAs. These techniques have a role in the emergency setting or as a bail out in the event of inadvertent side branch coverage during conventional endovascular repair.
Fenestrated and Branched Stent Grafts
The use of fenestrations and directional branches makes logical sense, given that these devices have been specifically designed to incorporate side branches and follow basic engineering principles to assure adequate seal. The current worldwide experience with manufactured devices uses the Cook Z stent platform. TAAA stent-graft design includes one of two options. An off-the-shelf multibranch stent graft (t-Branch, Cook Australia) pioneered by Tim Chuter from UCSF includes four downgoing directional branches (Fig. 29.2) intended to be accessed via brachial approach and bridged to the target vessel by self-expandable stent grafts. Directional branches are optimal for downgoing vessels which originate from a large aneurysm sac, where there is significant gap between the aortic stent graft and the aortic wall. Alternatively, a custom-made option (Fig. 29.2) is also available which requires a time delay of 6 to 8 weeks for manufacturing in Brisbane, Australia. These devices can have any combination of branches and fenestrations to fit the patient’s anatomical needs. Because the renal arteries are often transversely oriented or upgoing and have smaller size diameter, the use of downgoing branches is often not ideal. In these cases, a fenestrated device or combination of branches for the celiac axis and SMA with two fenestrations for the renal arteries is utilized. Fenestrations are the preferred method of incorporation for vessels that originate from narrow aortic segments, such as frequently observed with chronic dissections and type IV TAAAs.

Aortic Imaging
A computed tomographic angiography (CTA) is the primary imaging modality used to plan these procedures. Several anatomical considerations have been previously discussed. Patients with severe allergic reactions to iodinated contrast may need noncontrast CT or
gated MRA. Direct intra-arterial catheter injection can be used during CTA to minimize contrast dose. Analysis of digital datasets is done using one of several available softwares, such as M2S (Medical Metrx Software, West Lebanon, NH), Vitrea (Vital Images, Minnetonka, MN), and Aquarius (TeraRecon Inc., San Mateo, CA), to reconstruct imaging by tracting an orthogonal centerline of flow line and virtual grafts to aid in measurements and procedure planning.
gated MRA. Direct intra-arterial catheter injection can be used during CTA to minimize contrast dose. Analysis of digital datasets is done using one of several available softwares, such as M2S (Medical Metrx Software, West Lebanon, NH), Vitrea (Vital Images, Minnetonka, MN), and Aquarius (TeraRecon Inc., San Mateo, CA), to reconstruct imaging by tracting an orthogonal centerline of flow line and virtual grafts to aid in measurements and procedure planning.
The preoperative CTA should include chest, abdomen, and pelvis with and without contrast enhancement. Qualitative evaluation of arch disease for atheromatous burden, as well as the vertebral arteries, is critical to minimize the risk for cerebral ischemic complications when planning procedures in the distal arch or TAAA repair. For arch debranching, dedicated imaging of the extra and intracranial circulation may be needed. At a minimum, the carotid bifurcation should be interrogated using duplex ultrasound.
Pelvic flow via the internal iliac arteries is an important collateral network to the spinal cord, and should be preserved whenever possible in patients undergoing repair of thoracic or TAAAs. Similarly, the vertebral arteries are equally important and should be preserved via carotid–subclavian revascularization if needed.
Accurate measurements of aortic, target and access vessel diameter, and sealing sites determine the feasibility of the procedure and selection appropriate graft sizes. The stent graft is typically oversized by 10% to 20% in relation to the sealing zone diameter, which exception of acute dissections where lesser degree of oversizing (5% to 10%) is accepted. Diameters and orientation of the target vessels in relation to clock positions are best measured on orthogonal views; lengths are accurately measured using centerline of flow. When there is significant discrepancy in diameter between the proximal and distal landing zones, a tapered graft is selected.
Neuroprotection
Spinal cord injury remains a devastating complication after repair of thoracic and TAAAs. The main determinants are extent of aortic coverage, adequacy of collateral networks, prior aortic repair, and perioperative hypotension. The highest rate of spinal cord injury is observed with type II TAAAs. Although the frequency and type of spinal cord injury has changed in recent years, the threat of paraplegia continues to deter patients from undergoing elective operations to prevent rupture of extensive TAAA.
Significant progress has been made in the understanding of the anatomy and physiology of the spinal cord circulation in recent years. This allowed development of strategies to reduce risk of spinal cord injury (Fig. 29.3). The classic description that spinal
cord perfusion is dependent upon a single prominent branch from the descending aorta with a distinctive hairpin configuration, the so-called artery of Adamkiewicz, usually located between T8 and L2, has been challenged by evidence of a significant collateral network. Recent studies demonstrate that the spinal collateral network adapts quickly to ischemia, including change in vessel diameter and orientation of capillaries. Collateral flow is provided by a robust paraspinal muscle arterial network, segmental parietal arteries (SAs), both intercostal and lumbar, internal iliac, and vertebral artery branches. These vessels communicate via arcuate branches to the anterior spinal artery (ASA). In addition to multiple SA inputs, the ASA is also fed by an extensive epidural arterial network, and by a dense array of small vessels which supply the paraspinal musculature. All these vessels are interconnected, and have major anastomoses with the subclavian arteries cranially, and the hypogastric arteries caudally. This extensive collateral network allows compensatory flow to the spinal cord when some of the direct inputs to the ASA are compromised during repair of TAAAs.
cord perfusion is dependent upon a single prominent branch from the descending aorta with a distinctive hairpin configuration, the so-called artery of Adamkiewicz, usually located between T8 and L2, has been challenged by evidence of a significant collateral network. Recent studies demonstrate that the spinal collateral network adapts quickly to ischemia, including change in vessel diameter and orientation of capillaries. Collateral flow is provided by a robust paraspinal muscle arterial network, segmental parietal arteries (SAs), both intercostal and lumbar, internal iliac, and vertebral artery branches. These vessels communicate via arcuate branches to the anterior spinal artery (ASA). In addition to multiple SA inputs, the ASA is also fed by an extensive epidural arterial network, and by a dense array of small vessels which supply the paraspinal musculature. All these vessels are interconnected, and have major anastomoses with the subclavian arteries cranially, and the hypogastric arteries caudally. This extensive collateral network allows compensatory flow to the spinal cord when some of the direct inputs to the ASA are compromised during repair of TAAAs.
The concept of collateral spinal network led to development of staged endovascular strategies (Fig. 29.3) to minimize risk of ischemic injury while optimizing development of larger collaterals. This has been based on experimental evidence that a staged repair allows not only the development of larger collaterals with maintenance of collateral perfusion pressure, but also faster recovery to normal spinal perfusion pressures and reduction on risk of paraplegia. The Cleveland Clinic group demonstrated that a staged approach for type II TAAAs reduced risk of paraplegia and operative mortality as compared to a single-stage coverage of the entire aorta.
Hemodynamic instability because of bleeding or systemic complications can be deleterious, even in the presence of large anastomotic channels and has been associated with increased risk of SCI. The ideal mean arterial blood pressure should be maintained at >80 mm Hg. Increase in cerebral spinal fluid pressure has also been referred as potential cause of spinal ischemia. Based on the Monroe–Kellie hypothesis, perfusion pressure of the brain and spinal cord is directly influenced by the volume of cerebrospinal fluid as well as intracranial pressure. Drainage of cerebrospinal fluid is recommended in all patients undergoing coverage of the mid or distal thirds of the thoracic aorta, and in all patients with type I to III TAAAs. The authors’ preference is to liberally use spinal drainage also for type IV TAAAs.
A cerebrospinal drain is typically inserted immediately prior to the procedure at the level of L3-L4 and maintained for 48 to 72 hours, while keeping pressure less than 10 cm H2O. The incidence of hemorrhagic complications from catheter placement is also not negligible, and such complications are reported in 0% to 3% of patients. Other intraoperative adjunct that has been used is motor-evoked potential (MEP) and somatosentory evoked potential (SSEP) monitoring. The authors use this routinely in all TAAAs. It can help change the strategy of repair during the procedure, by allowing immediate identification of ischemia and further reduction of CSF pressure to 0 or 5 cm H2O, in addition to further increase in MAPs. Furthermore, the sequence of stent-graft placement may change, with immediate restoration of flow into the lower extremities, followed by placement of the side branch stents as the last part of the procedure. Delayed paraplegia after TEVAR has to be kept in account; specifically for this complication, CSF drainage has been shown to be effective in reversal of delayed-onset paraplegia after TEVAR and should be used whenever SCI is suspected.
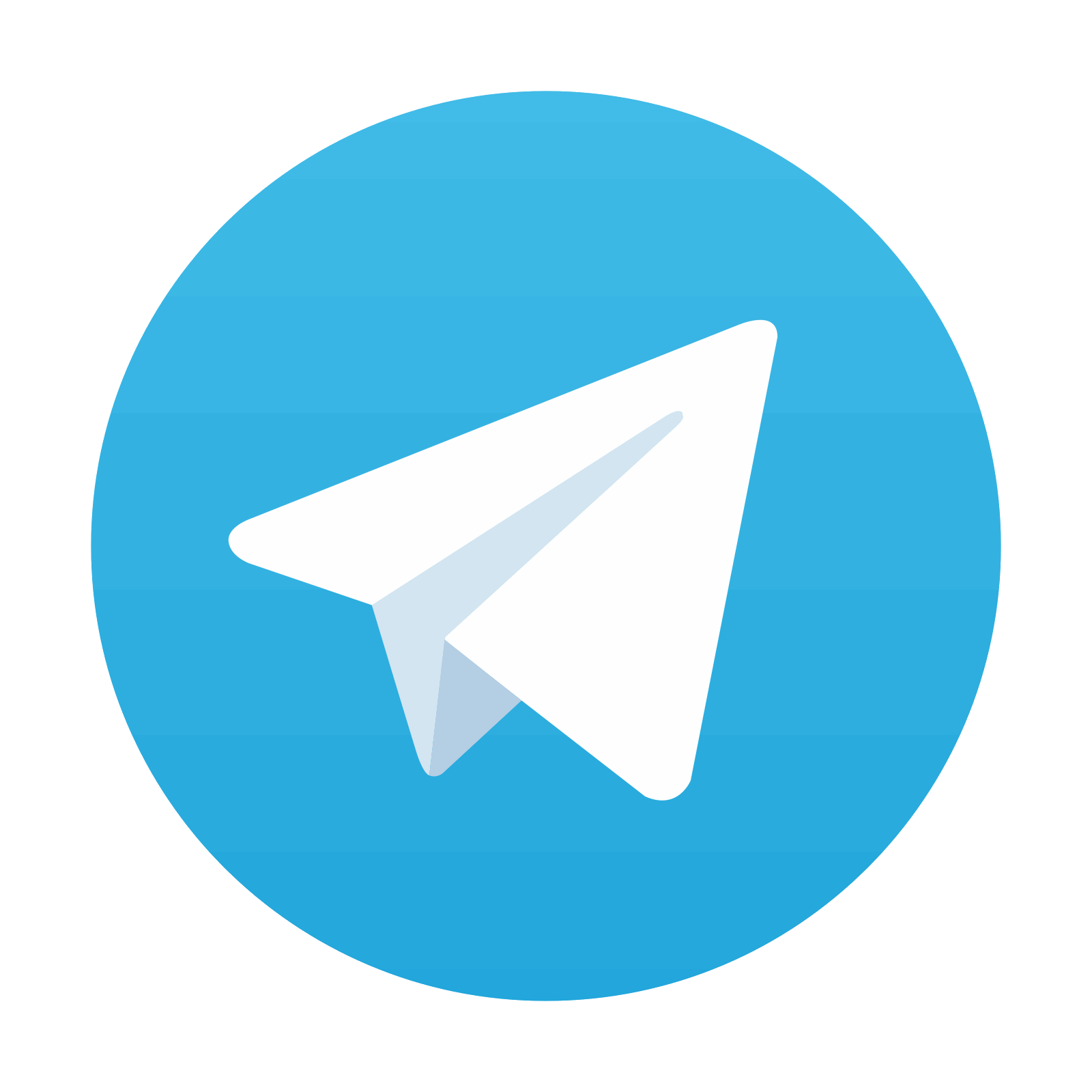
Stay updated, free articles. Join our Telegram channel

Full access? Get Clinical Tree
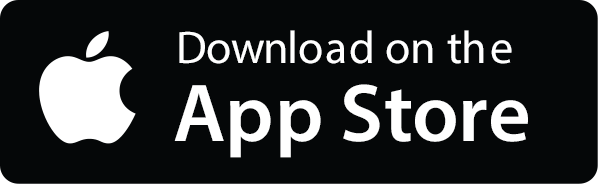
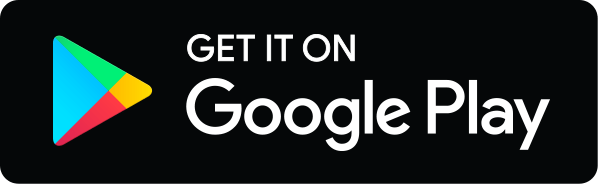