Fig. 8.1
a CCA illustrating MAPCAs in situ. b Axial CT image illustrating overriding aorta, hypertrophied right ventricle, and MAPCAs. c 2D echo illustrating overriding aorta. d 3D reconstruction of CT dataset from a posterior angle. MAPCAs are colored various shades of green; see Table 8.1 for full color explanation. Cardiac catheterization angiography (CCA), major aortopulmonary collateral arteries (MAPCAs), computed tomography (CT), three-dimensional (3D)
We will not review the various methods (and controversies) of unifocalization, one-stage or multistage approach, or the benefits/deficits of a transatrial or transventricular approach as a better option for repair. This chapter will investigate the role of 3D modeling and 3D printing as a means to support an institutions’ approach to TOF/MAPCA repair.
Current Imaging Modalities
Overview
For diagnostic evaluation, 3D modeling, and presurgical planning, accurate clinical images are important to understanding the cardiac morphology of patients with TOF and MAPCAs. Here, we discuss the different common imaging modalities as they relate to 3D modeling and 3D printing of TOF/MAPCA anatomy.
2D and 3D Echocardiography
Two-dimensional (2D) echocardiogram (echo) is typically sufficient in defining most structures important for surgical repair of TOF. Echo is particularly well suited to noninvasively locate and define the shape of the anterior malalignment VSD and any additional VSDs. Noting the size and location of these VSDs can be important to surgical success [11]. It is also useful to note the presence of an anomalous left anterior descending from the right coronary artery across the infundibulum of the right ventricle for preoperative planning of a ventriculotomy. Due to the relatively small diameter of pulmonary vessels and MAPCAs, 2D echo may not be an ideal tool for observing the size, course, and tortuosity of the collateral vessels, especially when they course through the parenchyma.
With regard to 3D printing, 2D echo is largely insufficient and lacks significant image processing algorithms needed for anatomical reconstruction. 3D echo provides opportunities for reconstructing TOF anatomy, although it too has its limitations. Similar to 2D echo, 3D echo can accurately capture large defects such as the malaligned VSD and may illustrate eccentricity of pulmonary stenosis, but discontinuous vessels in the lung’s parenchyma may be lost. In addition, the field of view for 3D echo is insufficient in capturing the entire cardiac anatomy, and its low signal-to-noise ratio for small structures, pulmonary vessels, and MAPCAs make it less appealing for anatomical reconstruction and 3D printing. However, a multimodal approach where 3D echo data is coregistered with computed tomography (CT) or magnetic resonance (MR) angiography dataset holds some promise of bringing 3D echo’s strengths to clinical 3D printing [12]. In fact, the shape and location of the malaligned VSD may best be described by this multimodality approach to 3D printing. Additional image processing technology will need to be developed to improve the signal-to-noise ratio of 3D echo in addition to improve coregistering techniques with other image modalities.
Cardiac Catheterization Angiography
Traditionally, cardiac catheterization angiography (CCA) is considered the gold standard for the identification of muscular VSDs, true pulmonary arteries, and MAPCAs in TOF/MAPCA patients. CCA procedures are well suited for identifying MAPCAs and their paths, especially in hemodynamically complex cases such as in the presence of aortopulmonary shunts [13]. This is in part due to the interventionalists’ control of where and how much contrast is deployed during the procedure.
Although certainly useful for defining the morphology of a TOF lesion or identifying MAPCAs, CCA has drawbacks in regard to patient safety. While minimally invasive, CCA does have inherent risks associated with any catheterization procedure including bleeding, arrhythmia, vessel damage, and infections [14]. In addition, CCA procedures involve radiation exposure and serial investigations can exacerbate the radiation dose [13, 15, 16]. Median effective radiation doses in comparative studies showed an increase of 1400% from CCA as compared to CT with some simulations putting potential dose increases as high as 7100% greater than minimal radiation CT [13, 17–19]. Further, compounding the issue of radiation exposure is the lowered rates of mortality for the TOF population [20, 21]. While surgical and interventional procedures are enabling longer lives for patients with TOF, higher accumulative radiation exposure may lead to secondary complications.
Direct 3D modeling or 3D printing solely from CCA images is a challenge due to the 2D image acquisition. Multiple perspectives or rotational techniques in conjunction with image processing would be needed to create a 3D print. However, these techniques are seldom used due to lack of spatial (volumetric) resolution, that is, resolution defining a volumetric feature. CCA images can be used to compliment the segmentation and anatomical reconstruction of CT or MR images as they provide supplementary information regarding the morphology and course of the MAPCAs and other key TOF structures.
Computed Tomography
CT has traditionally been used as a support tool for CCA as it can help to identify the number and location of MAPCAs [22]. The intended utility is to reduce the amount of time under CCA, thereby decreasing radiation exposure. CT also allows visualization of the pulmonary parenchymal anatomy. Emerging CT technologies have enhanced the acquisition of images so that they offer clarity comparable to CCA. In fact, studies support that CT is as accurate for MAPCA measurement as traditional CCA-based measuring schemes [13]. In the past, the decision of whether to perform a complete, two-ventricle repair with closure of the VSD was based on linear measurements of the pulmonary arteries from CCA. These metrics are known as the Nakata (pulmonary artery) index and the McGoon ratio [23, 24]. CT and MR imaging afford the capabilities to reassess these criteria with volumetric measurements of the pulmonary arteries. The volumetric nature of CT images yields well to the creation of 3D models (Fig. 8.1). These CT-based models are well described in the literature [25, 26].
Magnetic Resonance Imaging
Magnetic resonance imaging (MRI) is presently in a state of rapid advancement and evolution. Current generation technologies can provide an enormous amount of both structural and functional information. Volumetric 3D MR angiography can be used to identify key structures such as the pulmonary arteries and MAPCAs as accurately as CT methods [15, 27]. However, current MR technologies do not have the spatial resolution offered by CT, resulting in a loss of fidelity of minute structures. MR angiography has made great strides in the recent past and may soon overcome CT technologies with regard to volumetric datasets. In addition, less contraindications for MR angiography and the lack of radiation make MR angiography an appealing alternative for TOF/MAPCA diagnosis and surgical planning.
3D Modeling and Printing
Overview
3D visualization and 3D printing are well suited to aid in the understanding of morphologically complex CHD lesions. Anatomic reconstruction and visualization begin with image acquisition. For the remainder of the chapter, we will focus on current and emerging technologies and how they impact TOF/MAPCA evaluation and surgical/interventional planning.
3D Reconstruction
Following image acquisition, medical images are brought into a medical image software suite featuring segmentation tools. Segmentation is the image processing technique where an image, or image dataset in the case of volumetric patient data, is separated into discrete parts (Fig. 8.2). Segmentation is performed largely based on two concepts: discontinuity and/or similarity of intensity values. In discontinuity segmentation, an image is partitioned based on abrupt changes in intensity, such as edges in an image [28]. In similarity segmentation, an image is partitioned based on intensity criteria such as thresholding, region growing, and Boolean operations [28].


Fig. 8.2
a Segmented mask (multicolor) of the cardiac blood volume. b Polygonal reconstruction from the blood volume mask. c, d Full colored 3D reconstruction from CT dataset. Computed tomography (CT)
Specific challenges to TOF reconstruction include segmentation of all distal pulmonary vessels and MAPCAs. As MAPCAs typically travel through the lung parenchyma, CT or MR identification can be difficult. Even in cases where MAPCAs are identifiable, pixel intensity value for these vessels may be effectively similar to the intensity value of soft tissue. Global thresholding techniques are not as useful as local thresholding techniques or more advanced image processing techniques such as marching boxes.
Care should be taken in the reconstruction of the pulmonary arteries, pulmonary veins, MAPCAs, right ventricular outflow tract (RVOT), and the coronary arteries. An aberrant origin of the left anterior descending coronary artery from the right coronary sinus in TOF is ideal to model in cases for surgical preparation. Surgical interventions such as the creation of a right ventricle to pulmonary artery conduit may compress the aberrant coronary artery that crosses the RVOT. A 3D model can accurately display the course of these coronaries and their relationships to surrounding, critical structures.
Fused Filament Modeling
Fused filament modeling (FFF), also commonly known as the trademarked fused deposition modeling (FDM, Stratasys), is currently the most cost effective form of 3D printing. FFF 3D printers can currently be purchased under $300 at consumer electronic stores. This 3D printing technology entails the use of a plastic filament that is heated past its glass transition temperature and extruded onto a build platform. The 3D printer extrudes each layer of the intended model with the near-molten plastic. Common materials for this process include acrylonitrile butadiene styrene (ABS), polylactic acid (PLA), and nylon [29, 30]. Material properties for this 3D printing technology include high durability and low elasticity. Advantages in using this technique include low cost for printer and materials, durability, and post processing applications. Limitations of this technology include comparatively low-resolution capabilities, limited color palette, and limited non-rigid options.
While FFF technology may be more affordable than other printing methods, its printing resolution limitations and support generation make the technology less than ideal when 3D modeling TOF/MAPCA and its small, delicate features. Due to these limitations, we recommend utilizing FFF technology for macrostructures such as the shape of the overriding aorta or the hypertrophied right ventricle.
Powder Bed/Inkjet Printing
Inkjet printing technology entails the use of a binding agent ejected through a printhead onto a bed of powder media such as gypsum or acrylic. Depending on the technology, the binding agent acts either as an adhesive, forming a resin, or as a plastic binding agent, fusing the plastic powder media. A 3D object is printed through the many depositions of this binding agent onto the powder bed until the object is complete [29, 30]. Until recently, inkjet printing was the only method of achieving full color 3D prints.
The role of color in informing clinicians and the patient’s family about a patient’s CHD cannot be understated. In an educational environment, the utility of color-coded 3D-printed heart models led to statistically significant higher responses to questions probing CHD lesions and morphology (Table 8.1) [26]. The application of color can also assist clinicians in evaluating and differentiating the heart’s true pulmonary arteries from MAPCAs. In addition, serial CT or MR studies in conjunction with color 3D prints can enable a clinician to designate pulmonary vessels with unique colors (Fig. 8.3). The unique colors of an earlier time point when compared to a later time point may aid in understanding the development of the patient’s true pulmonary arteries.
Table 8.1
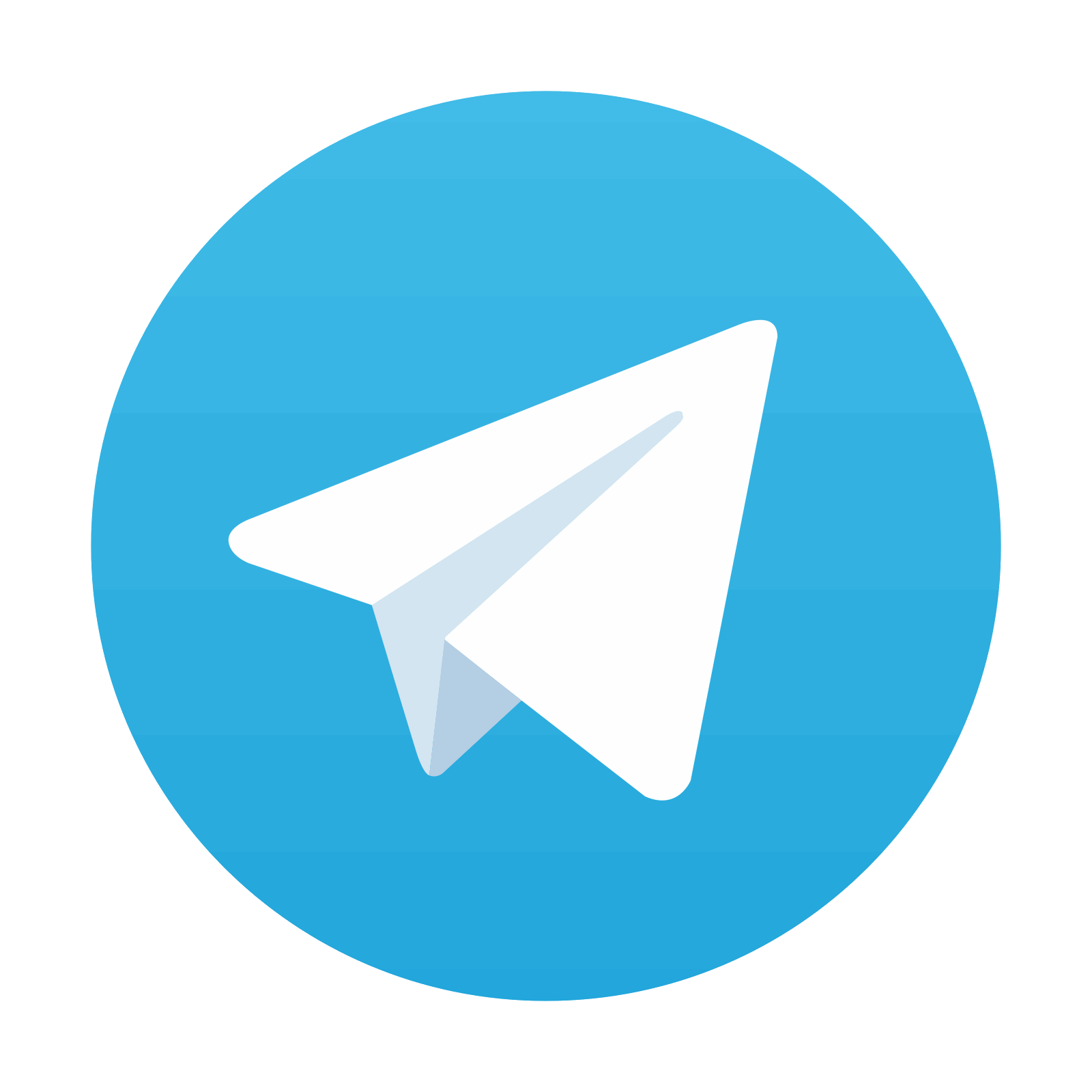
Color-coding scheme for 3D-printed blood volumes as developed by Richardson et al. [26]
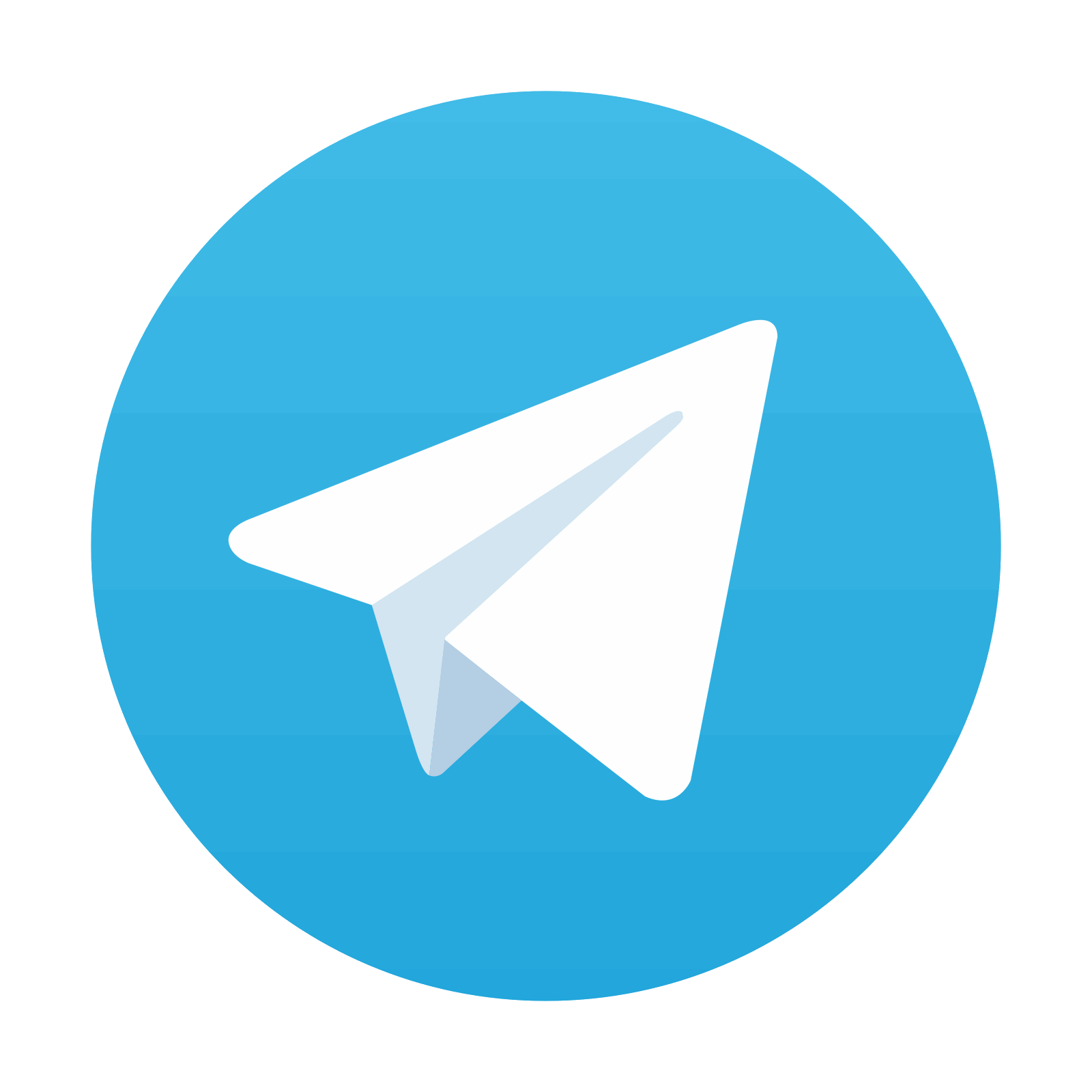
Stay updated, free articles. Join our Telegram channel

Full access? Get Clinical Tree
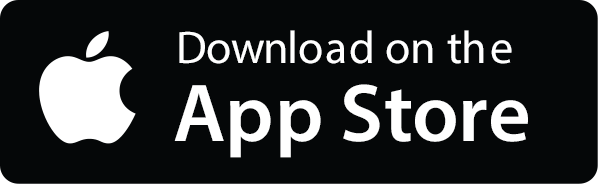
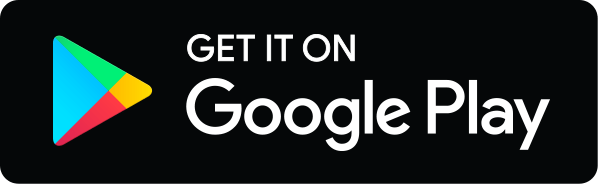
