Abstract
This chapter provides an overview of the topic of tetralogy of Fallot with and without pulmonary atresia, covering areas from history and genetics to current management issues and methods, to long-term outcomes. It specifically addresses areas of relevance to the clinician in practice. While providing a reasonably balanced perspective of controversial issues, it emphasizes the general consensus (where it exists) and shares the personal perspective of the authors for limited topics.
Key Words
tetralogy, ventricular septal defect, pulmonary stenosis, pulmonary atresia, JET
The treatment of tetralogy of Fallot (TOF) exemplifies both the remarkable success and the questions and challenges associated with “successful repair” and long-term survival. Although TOF was once uniformly lethal, current operative mortality is less 2%, and it is estimated that more than 90% of infants with TOF repaired in the current era will survive to the fifth and sixth decades of life. Improvements in perioperative care and longer-term management enabled this success, as well as an appreciation of the fact that the postrepair right ventricle (RV) remains a potential source of morbidity throughout life.
TOF occurs without gender predilection in approximately 0.3 of every 1000 live births and accounts for 3% to 4 % of all infants with congenital heart disease. The cause of TOF is unknown. Neils Stenson first described this defect nearly 350 years ago (1671); however, it was two decades later (1888) that Etienne-Louis Fallot formalized the defining features of TOF: (1) ventricular septal defect (VSD), (2) RV hypertrophy, (3) overriding aorta, and (4) right ventricular outflow tract obstruction (RVOTO). These features were later termed tetralogy of Fallot by Maude Abbott (1924).
Surgical intervention began with the systemic to pulmonary artery shunt (SPAS) by Blalock, Taussig, and Thomas in 1944. Lillehei et al. reported the first repair in 1954. By the early 1980s, surgeons were introducing primary repair in infancy. a
a References .
In the past decade most major centers have converged to a practice of repair in the first 3 to 6 months of life with greatly diminished use of palliative shunting.Anatomy
The segmental anatomy in patients with TOF is typically normal, with normal abdominal and atrial situs and concordant atrioventricular and ventriculoarterial connections. The right ventricular outflow tract (RVOT), pulmonary valve, and main/branch pulmonary arteries (PAs) constitute the most variable anatomic feature of TOF. Stenosis can occur at one or more levels and can range from mild to complete atresia of the pulmonary valve, dependent on the degree of anterior and cephalad deviation of the infundibular septum.
Tetralogy of Fallot With Pulmonary Stenosis
The more common variety of TOF with pulmonary stenosis (TOF/PS) consists of moderate stenosis of the RVOT, pulmonary valve, and main PA with branch PAs of adequate caliber ( Fig. 59.1 ). The pulmonary valve is bicuspid in approximately 75% of patients, unicuspid in less than 10%, and tricuspid in the remainder. Valve dysplasia occurs in 50% of bicuspid valves. The VSD is characterized by a large, nonrestrictive interventricular communication in the membranous septum created by the anterior and cephalad deviation of the infundibular (conal or outlet) septum relative to the crest of the ventricular septum. Additional muscular VSDs can occur but are infrequent. The malalignment of the infundibular and ventricular septa creates the aortic override, whereby a portion of the aortic valve exists on the right side of the projected septal plane and the aortic valve forms the “roof” of the interventricular communication. The aortic valve and root demonstrate variable degrees of clockwise rotation such that the right coronary sinus is more leftward. A right aortic arch is common (20% to 25%) as are an aberrant subclavian artery (10%) and persistence of a left superior vena cava (SVC) (7% to 10%).

Coronary artery abnormalities include an origin of the left anterior descending coronary artery (LAD) from the proximal right coronary or a duplicated LAD (approximately 5% to 7% cases) with the aberrant or additional vessel crossing the RVOT at variable distances from the pulmonary valve annulus. Less common is a single coronary artery from the left sinus giving rise to all major branches with the right coronary artery crossing the RVOT. A patent foramen ovale (PFO) or atrial septal defect is routinely present. Other concomitant anomalies include atrioventricular septal defect (5% of cases) or absent pulmonary valve (approximately 3% of cases).
Tetralogy of Fallot With Pulmonary Atresia
In addition to the usual anatomic features of TOF/PS, TOF with pulmonary atresia (TOF/PA) is defined by atresia of the pulmonary valve with variable hypoplasia of the main PA, ranging from a cord-like structure to a patent vessel ( Fig. 59.2 ). There is a higher likelihood of (1) additional muscular VSDs, (2) right aortic arch (25% to 50%), and (3) aberrant subclavian artery (15% to 20%). To avoid confusion with nomenclature and the terms PA/VSD versus TOF/PA, we use the classification of TOF/PA in reference to all forms of PA/VSD with concordant segmental anatomy in which the RV is developed and the goal is a two-ventricle circulation.

The hallmark and complicating feature of this diagnosis is the variable anatomy of the native central PAs and the presence of major aortopulmonary collateral arteries (MAPCAs) providing variable segmental pulmonary blood flow (see Fig. 59.2 ). In more than half of cases, native central PAs are well developed, supplied by a large ductus arteriosus, and provide flow to all bronchopulmonary segments. However, one-third of patients with TOF/PA do not have a ductus arteriosus. Patients with confluent native PAs may have MAPCAs providing either single or dual supply to respective segments. If central PAs are nonconfluent, MAPCAs will invariably be present, with the number of MAPCAs having an inverse relationship to the distribution of blood flow from the native central PAs. MAPCAs typically arise from the descending thoracic aorta in the region of the left bronchus or carina but can arise from the subclavian arteries, ascending aorta or the aorta below the diaphragm, or even the coronary arteries.
Genetics
Approximately 25% of patients with TOF have chromosomal abnormalities. Trisomy 21 and microdeletions of chromosome 22q11.2 constitute the majority, with trisomy 13 and 18 being much less common. Chromosome 22q11.2 microdeletions occur in approximately 20% of patients with TOF/PS and 40% of patients with TOF/PA. DiGeorge syndrome, a result of a more significant deletion, is associated with craniofacial abnormalities, immune deficiencies, hypocalcemia, and neurocognitive delay. Velocardiofacial syndrome is a less extensive deletion without the immune deficiencies or hypocalcemia. Chromosome 22q11 deletions have been reported to be associated with more severe abnormalities of the pulmonary vasculature in TOF/PA, longer duration of intensive care unit (ICU) stay, airway malacia, and an alarmingly high association with long-term neuropsychiatric disorders. In TOF/PS there has not been a uniform relationship with mortality.
Other associative or causative mutations include those of the jagged 1 gene (Alagille syndrome), NK2 homeobox 5 gene, GATA4, GATA6, forkhead box transcription factor 1, nodal, teratocarcinoma-derived growth factor 1, and growth differentiation factor 1. TOF-associated copy number variants have been linked to chromosomes 22q11.2, 3p25.1, 1q21.1, and 7p21.3 and the plexin A2 gene.
Overall, the risk for TOF recurrence in a family is approximately 3%. Screening may include fluorescence in situ hybridization or microarray with more specific or extensive analysis pending preliminary results. Programs with dedicated cardiac geneticists are currently developing testing panels to optimize utility and minimize cost. Results have implications for not only prognosis but also screening related family members.
Physiology
Tetralogy of Fallot With Pulmonary Stenosis
Because the VSD is nonrestrictive, the relative resistances to systemic and pulmonary blood flow dictate the amount and direction of flow across the VSD. With severe RVOTO, flow will be right to left, resulting in cyanosis. With mild RVOTO, flow will be left to right, resulting in pulmonary overcirculation. The more typical situation is somewhere between these two extremes. As the intrinsic pulmonary vascular resistance decreases in the first 1 to 2 weeks of life, and with dynamic narrowing of the RVOT, the physiology may change over time.
Hypercyanotic episodes or “Tet” spells occur because of a marked decrease in the ratio of pulmonary to systemic blood flow (Q p :Q s ) and concomitant increase in right-to-left shunting across the VSD. Episodes can be provoked by a decrease in systemic vascular resistance or an increase in resistance to pulmonary blood flow. The desaturation and resulting metabolic acidosis can increase the pulmonary vascular resistance, thereby worsening the right-to-left shunting. Thresholds for defining hypercyanosis vary (arterial oxygen saturation [SaO 2 ] <70% to <80%), and a severe episode can be associated with irritability, hyperpnea, marked cyanosis, pallor, and lethargy or loss of consciousness. Fortunately, the classic Tet spell is uncommon in developed health care settings where patients with severe RVOTO at risk for hypercyanotic episodes undergo surgical correction at a young age.
Tetralogy of Fallot With Pulmonary Atresia
In contrast to TOF/PS, in which a portion of the systemic venous blood passes antegrade through the RV and into the pulmonary bed, in TOF/PA all pulmonary blood flow is from the aorta—either from a patent ductus arteriosus (PDA) supplying central PAs, MAPCAs, or a combination of the two. Systemic venous blood passes through the VSD from the RV to the left ventricle (LV) to mix with pulmonary venous blood before ejection into the aorta. As such, pulmonary arterial and systemic arterial oxygen saturations will be equal. Oxygen saturation levels greater than 85% indicate a Q p :Q s of 2 : 1 or greater, whereas saturations in the 70% range indicate a Q p :Q s of 1 or less. As in TOF/PS, patients with TOF/PA may have evidence of inadequate, adequate, or excessive net pulmonary blood flow depending on the size of the PDA and/or size and number of MAPCAs.
In the presence of MAPCAs, systemic oxygen saturations may not reveal the variable local physiology occurring in various bronchopulmonary segments. Certain segments may receive high-flow, high-pressure blood from nonstenotic MAPCAs, whereas other segments may receive substantially lower flow due to various degrees of MAPCA origin stenosis or intrinsic limitations of the native PAs. The net oxygen saturation may be “acceptable,” despite the deleterious physiology occurring in local segments.
Diagnosis
Clinical Manifestations
If TOF/PS is left unrepaired, mortality rates for patients with TOF/PS reach 30% by 6 months and 50% by 2 years with fewer than 10% expected to reach age 21 years. The rare adult patient who survives with unrepaired TOF is at high risk for morbidity and mortality due to complications related to severe cyanosis, exercise intolerance, arrhythmias, cerebral abscess, and congestive heart failure due to long-standing RV hypertension. Patients with unrepaired TOF/PA have a worse prognosis, with a mortality rate of 50% by 1 year and 85% by 5 years. Fortunately, it is now rare for children to go unrepaired beyond infancy, and due to advances in fetal echocardiography, a greater percentage of patients with TOF/PS or TOF/PA are diagnosed prenatally.
In patients with TOF/PS and mild RVOTO the presentation is similar to that of a VSD with symptoms dependent on the degree of pulmonary overcirculation. Patients with an intermediate degree of RVOTO may not be symptomatic with a relatively balanced Q p :Q s and no significant right-to-left shunting at baseline to cause cyanosis. If RVOTO is more significant, right-to-left shunting across the VSD will result in cyanosis. In the most severe form of RVOTO, antegrade blood flow across the pulmonary valve is not sufficient, and pulmonary blood flow is dependent on a PDA supplying the central PAs.
For TOF/PA most neonates with MAPCAs are asymptomatic, with adequate pulmonary blood flow resulting in acceptable oxygen saturations without congestive heart failure. Over time the MAPCAs tend to develop stenoses, which may limit pulmonary blood flow and result in cyanosis. In addition, if untreated for years, pulmonary vascular disease may develop in segments supplied by unobstructed MAPCAs, leading to a net decrease in pulmonary blood flow. More rarely, MAPCAs result in excessive pulmonary blood flow over the first few weeks of life as the pulmonary vascular resistance drops, resulting in congestive heart failure symptoms of tachypnea and failure to thrive.
Physical Examination
Infants with TOF/PS and TOF/PA are generally full sized and normal in appearance in the absence of an associated genetic anomaly. Growth failure or progressive cyanosis may develop over time if there is congestive heart failure or inadequate pulmonary blood flow. Hypertrophic osteoarthropathy may be present in the unrepaired older child with long-standing cyanosis. Auscultation of the chest typically reveals a harsh systolic ejection murmur along the left upper sternal border, reflecting flow across the stenotic outflow tract. Absence of a systolic ejection murmur should raise suspicion of pulmonary atresia, and the murmur may decrease in intensity or become absent if there is little to no flow across the RVOT (such as during a hypercyanotic spell). The second heart sound is usually single and of normal intensity and may be accompanied by an ejection click. A continuous murmur may be present in TOF/PA over the site of a large MAPCA or PDA, which may increase in intensity as the pulmonary vascular resistance decreases.
Diagnostic Tests
Chest radiographic findings depend on the degree of pulmonary blood flow because pulmonary vascular markings may be decreased, normal, or increased, depending on the degree of RVOTO in TOF/PS and collateral circulation in TOF/PA. The great vessel shadow is diminished in the superior mediastinum because of the diminished caliber of the main PA. The hallmark of TOF is a boot-shaped heart with a prominent upturned cardiac apex related to the RV hypertrophy and concavity in the region of the main PA. The electrocardiogram should reflect the degree of RV hypertrophy and demonstrate right axis deviation, upright and peaked T waves in the right precordial leads, and reversal of the R/S ratio.
Echocardiography is the mainstay of diagnosis for intracardiac anatomy. Improvements in two-dimensional echocardiography and color flow Doppler have significantly reduced the need for cardiac catheterization. The intracardiac anatomy, aortic arch sidedness, presence of a PDA, and coronary anatomy can accurately be determined with echocardiography. The parasternal and subcostal windows best demonstrate the characteristic features of TOF, including anterior, cephalad deviation of the infundibular septum and the VSD ( Fig. 59.3 ). Interrogation of the blood flow across the RVOT in these views with color and spectral Doppler provides both qualitative and quantitative assessment of the degree of RVOTO. Measurements of the pulmonary valve annulus and main PA help determine the need for patch augmentation of the pulmonary annulus during surgical repair.

For patients with TOF/PA, echocardiography with color flow imaging can be used to demonstrate the lack of continuity between the RV and the main PA. There is an inverse relationship between the size of native branch pulmonary arteries and the presence of MAPCAs, such that MAPCAs are more likely to be present when native branch pulmonary arteries are severely hypoplastic (echocardiographically derived PA diameter z score < −2.5). Branch pulmonary arteries may also be discontinuous with bilateral PDAs supplying the right and left pulmonary arteries. The limitation of echocardiogram in TOF/PA is the inability to accurately delineate all sources of pulmonary blood supply when MAPCAs are present.
Cardiac catheterization and angiography are necessary in the majority of cases of TOF/PA. Angiography provides a thorough anatomic description of the native PAs and MAPCAs and the source of flow to each lung segment ( Fig. 59.4 ). Surgical planning relies upon this information to determine which MAPCAs should be unifocalized to preserve flow to a given segment. Catheterization may not be necessary if there are confluent central PAs supplied by a PDA without MAPCAs seen on ancillary imaging studies.

Angiographic assessment of the central PA size has been used as a predictor for postoperative RV pressures and mortality. The McGoon ratio is the summed diameters of the right and left PA divided by the diameter of the lower thoracic aorta. A McGoon ratio of greater than 1.2 predicts that postoperative RV systolic pressure will be acceptable, and a ratio of less than 0.8 suggests inadequate PA size for complete intracardiac repair. The Nakata index is determined by the summed cross-sectional areas of the right and left PA relative to body surface area, with an index of greater than 150 mm 2 /m 2 acceptable for a complete intracardiac repair. However, both the McGoon ratio and Nakata index may not apply to patients with multiple sources of pulmonary blood flow from unrepaired MAPCAs, and their usefulness in infants is unclear.
Computed tomography and magnetic resonance imaging (MRI) are increasingly used to clarify anatomy before surgical repair. These modalities are specifically useful to demonstrate coronary artery anatomy and the three-dimensional relationship of MAPCAs to other structures in the mediastinum. Also, for TOF/PA MRI-determined flows may help determine suitability for VSD closure.
Laboratory analysis of the patient with TOF is similar to that of other patients with congenital heart disease (see Part IV), with particular emphasis on genetic and calcium abnormalities.
Initial Medical Management
Initial medical management of a patient with TOF depends on the severity of the anatomic defects. In patients with TOF/PS and mild to moderate RVOTO with adequate systemic oxygen saturation, no specific medical therapy is necessary. If there is more severe RVOTO, preoperative stabilization and maintenance of adequate pulmonary blood flow may be necessary. Neonates presenting with ductal dependent pulmonary blood flow require prostaglandin infusion (prostaglandin E 1 [PGE 1 ]) to maintain ductal patency. Management of infants with TOF/PA depends on the degree and stability of pulmonary blood flow. Patients without a ductal dependent circulation will likely not require any specific medical intervention before surgical palliation or repair unless there is excessive pulmonary blood flow causing congestive heart failure, in which case management with diuretics as well as provision of adequate calories to maintain somatic growth preoperatively would be appropriate. Patients with ductal dependent circulation would be treated with prostaglandin infusion to maintain ductal patency while awaiting surgical palliation or repair.
Whereas classic palliation of ductal dependent TOF usually includes a surgically placed aortopulmonary shunt (SPAS), palliation of RVOTO with pulmonary valve balloon valvuloplasty or RVOT stent placement in selected cases may relieve significant cyanosis and allow for somatic growth and growth of PAs before definitive surgical correction. In addition, coiling of aortopulmonary collaterals providing dual-source pulmonary blood flow may also be performed to promote native PA growth.
Surgical Management
Intraoperative Monitoring
As for most cardiac procedures in the current era, access and monitoring include the following: (1) one to two peripheral intravenous lines, (2) central venous line, (3) arterial line, (4) pulse oximetry, (5) multilead electrocardiogram (ECG) monitoring, (6) Foley catheter, (7) esophageal and rectal or Foley catheter temperature probes, and in many centers (8) two-site near-infrared spectroscopy (NIRS) monitoring—cerebral and flank somatic. Preoperative and postoperative transesophageal echocardiography (TEE) is routinely performed for complete repair but may not be necessary for palliative SPAS.
Tetralogy of Fallot With Pulmonary Stenosis
Eventually, all patients will require surgical intervention. The major issues are (1) the timing of intervention and (2) whether to first palliate or to perform a complete repair. Patient condition determines the timing of intervention. The neonate dependent on PGE 1 to maintain adequate oxygen saturations should undergo intervention with either complete repair, SPAS, or stenting of the duct or RVOT. On the other hand, if oxygen saturations off PGE 1 are reasonable (>85%), repair may be deferred until the child is approximately 3 to 6 months of age or sooner if progressive cyanosis ensues. Timing of repair for the patient with minimal RVOT or PA stenosis (pink tetralogy) is identical to that for a patient with an unrestrictive VSD, preferably by 6 months of age.
Opinion or preference for initial palliation with SPAS versus complete repair varies among surgeons and institutions. b
b References .
In general, most would currently agree that an older nonneonatal patient who requires intervention should undergo primary repair, rather than palliation, with exception perhaps for unusual circumstances.Opinion is more variable for the neonatal age group. Proponents of primary repair cite a noninferior operative mortality, avoidance of acute shunt complications, benefits of earlier restoration of normal oxygen saturations, and the need for only one operation. Proponents of initial palliation cite a noninferior overall mortality with two procedures and less morbidity and shorter length of neonatal stay. The belief that a SPAS decreases the use of transannular patching at the time of subsequent complete repair has not been established as fact. Although opinion varies, various patient factors might favor an initial palliative approach—prematurity, low patient weight, an LAD arising from the right coronary artery in a context in which a transannular or ventricular incision is anticipated, and/or significant comorbid medical conditions that would render cardiopulmonary bypass (CPB) excessively high risk.
Based on a large cohort from the Society of Thoracic Surgeons (STS) database spanning the interval of 2002 to 2007, approximately 10% of patients received a shunt—53% of neonates and 15% of patients in the age-group of 30 days to 3 months. By 6 months of age 61% had undergone repair. According to a more recent STS data report, the median age (interquartile range) at repair was 0.4 years (0.3 to 0.6). Although neonatal repair was associated with a somewhat higher discharge mortality compared with SPAS (7.9% versus 6.2%), this multicenter data does not address the overall mortality or morbidity of a particular strategy. Because convincing data and guidelines do not exist, it is reasonable to proceed with either strategy, taking into account the local expertise and infrastructure and what works best for a given care team.
Systemic to Pulmonary Artery Shunt.
In contrast to historical management, currently patients undergo a SPAS with the intent of complete repair within the next 4 to 8 months. Types of SPAS include a modified Blalock-Taussig shunt (MBTS), which connects the subclavian artery to the ipsilateral branch PA, and a central shunt, which connects the ascending aorta with the main PA ( Fig. 59.5 ). (For TOF/PS the latter would typically be a Gore-Tex shunt.) In the more recent era there is trend to do the SPAS procedure through a median sternotomy (required for central shunt) because it does not require partial or complete lung collapse, CPB can easily be implemented if required, and it leaves the child ultimately with one chest incision. Opinion on optimal shunt size varies, but in general for a 3.5-kg baby most would place either a 3.5- or 4-mm MBTS or a 3-mm central shunt. A heparin bolus is given before creating the shunt—dosing varies from 50 U/kg to in excess of 100 U/kg. Anticoagulation and antiplatelet management after surgery vary considerably among institutions. It is our bias to start a heparin drip in the operating room, if feasible, at approximately 20 U/kg/h. We also prefer to initiate aspirin in the first 12 hours after admission to the ICU and adjust dosing based on objective measures of platelet inhibition.

Management options for the PDA include ligation after completing the shunt or no ligation and simply discontinuing the PGE 1 . There are no data proving superiority of a particular approach. Ligation eliminates competitive flow, which may enhance flow through the shunt and reduce the risk of thrombosis, whereas spontaneous closure preserves the option of restarting PGE 1 to reopen the duct if there is concern for shunt thrombosis later in the ICU.
Repair.
The goals of repair are to (1) close all septal defects, (2) provide a durable and reasonably patent and competent outflow tract, (3) avoid or correct branch PA stenosis, and (4) result in normal sinus rhythm with good ventricular function, cardiac output, and oxygen delivery. We would consider leaving a small (2 mm) PFO only in a higher-risk neonate. Repair is done using bicaval venous drainage for CPB at temperatures ranging from 28°C to 34°C. Cardioplegic arrest is used for the intracardiac portion of the repair. Surgical options include (1) transatrial and trans-PA repair with the combined exposure allowing resection of RVOT muscle, VSD closure, pulmonary valvotomy, if needed, and patch enlargement of the main PA; (2) in addition to a pulmonary arteriotomy, use of a ventriculotomy for both exposure and patch closure of the VSD and to relieve RVOTO; and (3) transannular patching in which the incision on the main PA is extended across the annulus onto the RV for a variable distance ( Fig. 59.6 ). Limited transannular incision (<5 to 10 mm of ventricular incision) has been advocated to reduce the potential long-term negative impact on RV function; however, longer-term data supporting this approach are not yet available. In the context of a transannular patch, creation of a monocusp valve may improve early hemodynamics; however, this speculation and the long-term competence of the valve have not been confirmed. Branch PA stenosis may be addressed either by a separate patch or extension of the patch on the main PA. In general, small branch PA expand adequately after repair and do not require patch reconstruction. Postoperative TEE is used routinely in the operating room to evaluate ventricular systolic function and assess for residual intracardiac defects.



There are no data demonstrating clear superiority of a particular inotrope strategy in the operating room. We typically wean from CPB using epinephrine (dose range 0.02 to 0.1 mcg/kg/min) and consider a low-dose milrinone infusion without a loading dose if blood pressure is generous. For the sake of this discussion we presume the TEE demonstrates no residual VSD and at most mild tricuspid regurgitation. Although the TEE provides important information about the RVOT and pulmonary valve, in the absence of clearly unacceptable findings, we would obtain direct pressure measurements in the RV and PA before making a decision to return to CPB for further relief of RVOTO. In general we would accept a gradient of 25 to 30 mm Hg with an RV to systemic systolic pressure ratio of up to 0.6 : 1, acknowledging the variation in thresholds that may be suitable for a particular patient and circumstance.
A residual VSD further complicates decision making and should be avoided using vigilance at the time of repair. The interplay between a VSD and residual RVOTO may mask the significance of the VSD and/or imply a worse degree of RVOT stenosis. Although a high shunt fraction (calculated from blood samples from the SVC and PA) is informative, a low shunt fraction is not necessarily reassuring. Unless the residual VSD is very small (estimated by TEE at approximately 1 mm) and the RVOT gradient low, our bias is to attempt to close the defect.
The debated issue with repair is the significance of a transannular or ventricular incision. First, a transannular patch ultimately will leave the child with pulmonary insufficiency. Second, an incision on the RV may impair function, and the resultant scar may be a source for future dysrhythmia. These concerns are played against (1) a desire to provide adequate relief of RVOTO; (2) in some circumstances, perhaps better exposure to the cephalad aspect of the VSD afforded by a ventriculotomy and no need to manipulate the tricuspid valve (in our opinion, transatrial neonatal VSD repair can be a very challenging procedure, due solely to the meticulous care of the tricuspid valve required for a perfectly competent postrepair valve); and (3) need for less muscle resection afforded by a ventriculotomy and/or a transannular patch. Moreover, the threshold for defining adequate relief of RVOTO varies. Most would agree that an RV to systemic systolic pressure ratio of 0.7 with a gradient across the RVOT by direct intraoperative measurement of 45 is unacceptable. However, it is less clear what to do with a pressure ratio of 0.6 or 0.7 and a gradient of 35 mm Hg, particularly if the valve is perfectly competent and expected to remain so. The risks of progressive RVOTO and need for reoperation are weighed against the potential longer-term risk of pulmonary insufficiency and RV dysfunction. Most would agree, however, that the younger patient, particularly the neonate, may not tolerate residual RVOT stenosis, VSD, or tricuspid insufficiency. The main point is that the surgical decision should be individualized and take into account the overall condition of the heart and the patient. There is no single correct RVOT gradient threshold or RV to systemic pressure ratio. The driving principle is achieving a good repair, not avoiding a ventricular or transannular incision.
According to recent multicenter data from the United States only one in five patients were repaired without a ventriculotomy or a transannular patch (46% were repaired with a transannular patch). In contrast to programs in the United States, there may be greater willingness to tolerate residual outflow tract gradients in other parts of the world, with the belief that a certain threshold of residual gradient is well tolerated and may reduce the long-term consequences of pulmonary insufficiency and the need for pulmonary valve replacement (PVR). However, it is also possible that patient characteristics and age at presentation are relevant factors. In the United States, for example, the percentage of patients undergoing transannular patching ranges from approximately 80% in neonates to 50% in the 3- to 6-month age-group. Very few surgeons desire to make an incision in the RV of a neonate unless absolutely necessary. Therefore, if done in 80% of patients, it more realistically reflects the fact that a neonate requiring surgery likely has a degree of RVOTO that requires transannular patching to achieve an adequate repair.
Tetralogy of Fallot With Pulmonary Atresia
Practical constraints preclude a full description of the rationale and technical considerations for the various approaches to surgical management of patients with TOF/PA. Therefore we will describe the primary concerns and decision making and refer the reader to several references for additional detail. c
c References .
Management of a baby with confluent, good-size or mildly hypoplastic central PAs is identical to that of the TOF/PS patient, with the added step of establishing continuity between the RV and PAs, either with patch augmentation of the native RVOT if feasible or more often with placement of an RV to PA valved conduit. The one exception may be the need to eliminate the infrequent MAPCA to normalize the volume load on the heart.For the patient with MAPCAs and either severely hypoplastic or nonconfluent central PAs, the strategy is to develop a suitable pulmonary vascular bed leading ultimately to an arrangement with VSD closure and an RV to PA conduit. The specific strategy for developing the pulmonary vasculature depends on what view one takes of the embryologic origin of MAPCAs and their suitability as long-term sources of pulmonary blood flow. One view is that MAPCAs are not suitable or effective in the long term, and surgical strategy should focus on the native PAs. In contrast, another view is that MAPCAs are very suitable, in many cases essential to establishment of an adequate pulmonary vascular bed, and should be unifocalized early in the first few months of life for their benefit to be fully realized. Unifocalization refers to a surgical procedure that reduces multiple origins of pulmonary blood flow to ultimately one origin—the RV ( Fig. 59.7 ). Regardless of the viewpoint, it is paramount to define the native PA and MAPCA anatomy early in life, the number of segments supplied solely by native PAs, the number supplied solely by MAPCAs, and those with dual supply. The ultimate goal is to establish a pulmonary vascular bed with sufficient surface area to permit VSD closure with an acceptably low RV pressure and good gas exchange.

In the anti-MAPCA view the only option is to do various forms of SPAS or an RV to PA conduit to develop the native PAs—the latter may afford better access for interval catheter-based rehabilitation of the PAs, if needed, with replacement and upsizing of the conduit at the time of VSD closure. With the pro-MAPCA view, unifocalization is done to incorporate the MAPCAs into the pulmonary circulation. This is done by anastomosing MAPCAs to the native PAs either centrally, at the hilum, or more distally as needed. For absent central PAs, patch material may be needed to reconstruct the central PA (see Fig. 59.7 ). Depending on circumstances and institutional preference, this may be done in a single stage, typically via a median sternotomy (CPB as needed for the unifocalization), or in two stages via sequential thoracotomies. With the latter approach the two procedures can be separated by a few days during the same hospital admission or the child discharged and readmitted a few weeks later for the second procedure. For either approach, once the PAs are unifocalized, the source of pulmonary blood flow can be a SPAS or an RV to PA conduit. The latter may provide greater ease of access for catheter-based intervention for stenosis and rehabilitation of the vascular bed. For the sequential approach, SPASs (one for each side) are placed, with the bilateral circulations unifocalized centrally during a later procedure, typically requiring patch material to complete the unifocalization. If not placed at this time, an RV to PA conduit would be placed at the time of VSD closure and the SPASs taken down.
In general, patient condition drives timing of the first intervention. If there is profound cyanosis, ductal dependent central or branch PA blood flow, or dual-supply central PA/MAPCA flow amenable to shunt for growth of the central PAs, neonatal intervention may be required. Otherwise, the goal is to perform unifocalization in the first 3 to 6 months of life to preserve blood flow to all lung segments and prevent the development of pulmonary vascular disease from unrestrictive pulmonary blood flow.
Timing of VSD closure is variable—it may be possible at the initial single-stage unifocalization or may require weeks to months of PA growth and rehabilitation. Criteria to determine suitability have been described and continue to be used but remain imperfect. In several series, septation has been achieved in 60% to 80% of patients; however, reports of 15- to 30-year follow-up are lacking. If septation results in an RV to systemic pressure ratio of greater than 0.5, options are VSD patch takedown or fenestration. Unless the PA bed is further improved, this leads to the difficult circumstance of a cycle of repeated upsizing of SPAS or conduit replacements and the chronic effects of cyanosis, potentially ultimately palliated only with heart-lung transplantation.
This condition highlights the relevance of skilled, multidisciplinary teams with good judgment based on the primary principle of achieving a good pulmonary vascular bed. There is no need to rush to VSD closure in the context of a borderline vascular bed. Even with a seemingly good single-stage repair early in life, patients remain at risk for requiring further surgical or catheter-based interventions.
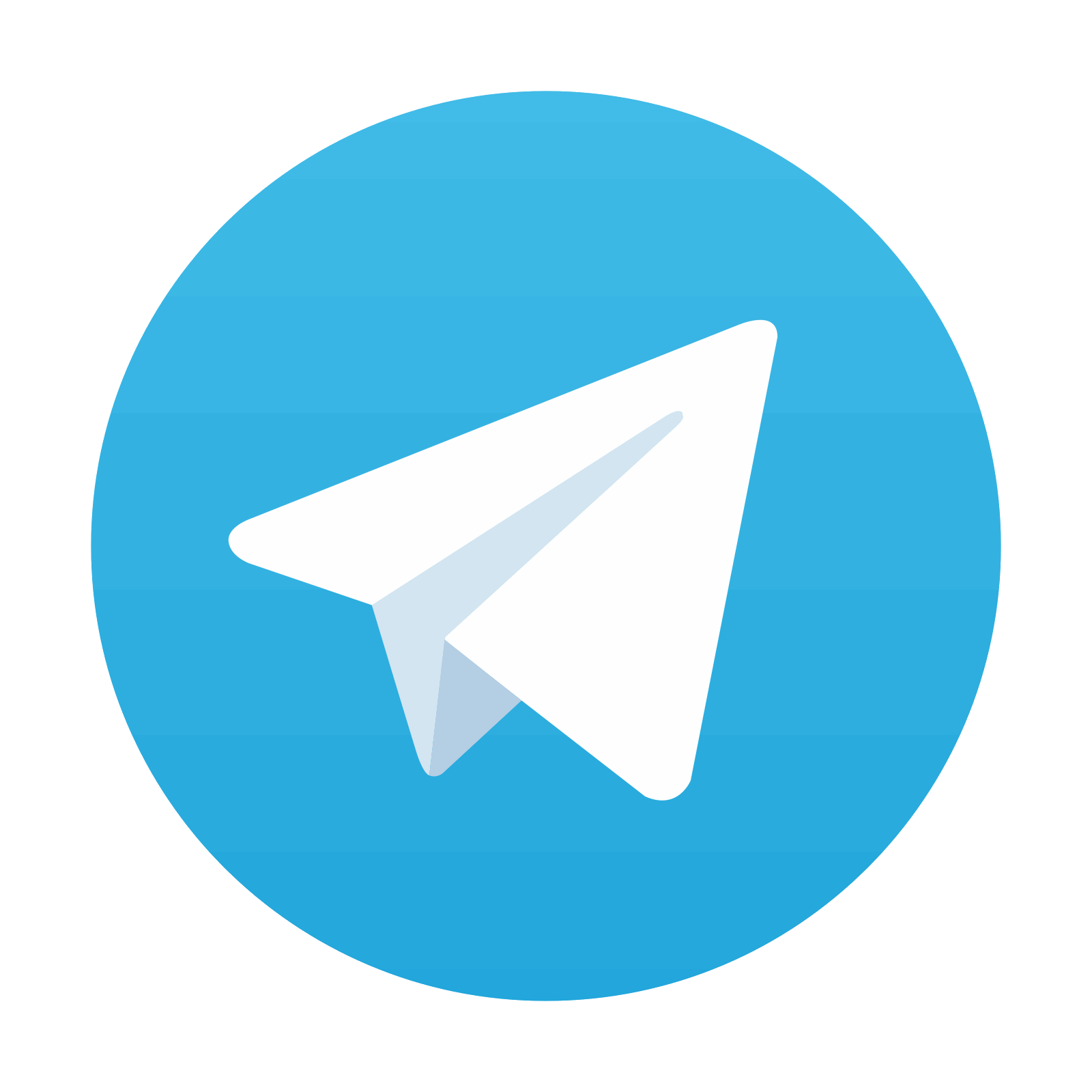
Stay updated, free articles. Join our Telegram channel

Full access? Get Clinical Tree
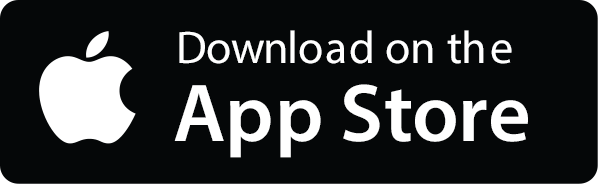
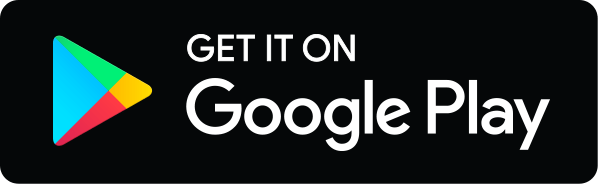