TETRALOGY OF FALLOT
Introduction
In Chapter 28, we began addressing individual cardiac defects and following the same theme, we will discuss tetralogy of Fallot (TOF) in this chapter. TOF is the most common cyanotic congenital heart defect (CHD) in children older than one year of age and forms 10% of all CHDs.1 But, in the neonate TOF is less frequently seen than transposition of the great arteries (the most common cyanotic CHD in the neonate)1,2 discussed in the preceding chapter. Fallot, a little more than 100 years ago,3 described a constellation of 4 abnormalities, now called TOF. These 4 abnormalities, as defined by Fallot are: (1) ventricular septal defect (VSD), (2) pulmonary stenosis (PS), (3) right ventricular (RV) hypertrophy, and (4) dextroposition of the aorta (Ao) (Figure 29.1). The VSD is usually large and nonrestrictive and is located in the subaortic region involving the membranous septum, sometimes extending into the muscular septum. Occasionally, additional muscular VSDs may be present. PS is variable both with regard to the site of obstruction and the degree of narrowing. The degree of obstruction may be very mild without any arterial desaturation and left-to-right shunt across the VSD or it may be very severe resulting in severe cyanosis even in the newborn period. The site of obstruction may be at the infundibular, valvar, supravalvar, or branch pulmonary artery (PA) level; infundibular stenosis is the most frequent lesion seen. The obstructive component may be at one site or may entail several sites. The most common infundibular obstruction in TOF is thought to be due to anterior and superior deviation of infundibular (conal) septum. The valvar obstruction may be secondary to pulmonary valve (PV) commissural fusion, valve ring hypoplasia or a combination thereof. RV hypertrophy is present in all cases and is usually severe in degree. Over-riding of Ao over the ventricular septum (dextroposition) is variable in degree. The Ao is enlarged and this is presumed to be secondary to developmental anomaly instead of pathophysiology of TOF. The Ao descends on the right side of the spine in 25% cases. Significant-sized atrial septal defects (ASDs) may be seen in 15% of the subjects with TOF; in such cases, it is described as pentology of Fallot.
Figure 29.1. Box diagram illustrating component lesions seen in tetralogy of Fallot: (1) ventricular septal defect, (2) pulmonary stenosis, (3) dextroposition of the Ao, and (4) RV hypertrophy. Ao, aorta; LA, left atrium; LV, left ventricle; PA, pulmonary artery; RA, right atrium RV, right ventricle. Reproduced with permission from Neonatology Today 2011;6(5):1–10.
The association of TOF with several syndrome complexes, namely, Down, CATCH-22, CHARGE, fetal alcohol, Pierre-Robin, Goldenhar’s, TAR (thrombocytopenia absent radius), VATER/VACTERAL, and Shprintzen’s (velocardiofacial) syndromes is well recognized. Anomalies of other organ systems, such as imperforate anus, tracheoesophageal fistula, omphalocele, and hydrocephalus, are also associated with TOF and may have therapeutic implications.
Pathophysiology
Since the VSD is large and nonrestrictive, the systolic pressures in the RV and the left ventricle (LV) are identical and both ventricles operate as one functional chamber. The amount of blood flow into the pulmonary and systemic circulations depends on their own resistances. The magnitude of systemic vascular resistance and right ventricular outflow tract (RVOT) stenosis resistance determine the flows. The more severe the pulmonary outflow stenosis, the less is the pulmonary flow. In the usual case of TOF, the resistance offered by pulmonary outflow tract obstruction is more than that offered by the systemic vascular tone and therefore, right-to-left shunt occurs across the VSD (Figure 29.2). The resultant O2 desaturation (hypoxemia) stimulates the production of erythropoietin in the kidney which in turn encourages bone marrow to produce more red blood cells.4 While increased red blood cells (polycythemia) are useful in augmenting the O2-carrying capacity, it becomes counterproductive when the hematocrit is extreme (>60% to 70%); however, this does not occur in the neonate. The additive effects of patent ductus arteriosus (PDA), aortopulmonary collateral (APC) vessels, and PA dilatation, compressing the trachea and bronchi, will be discussed in the respective sections.
Figure 29.2. Box diagram illustrating right-to-left shunt at ventricular level because of high resistance offered by PS. Abbreviations are same as in Figure 29.1. Reproduced with permission from Neonatology Today 2011;6(5):1–10.
Classification
There are a number of variants of TOF with differing presentations in the newborn period. In addition, the management of these neonates varies from one variant to the other. Based on these considerations, we prefer to classify5 neonatal TOF as follows: Type I: Classic TOF; Type II: TOF with pulmonary atresia; Type III: TOF with multiple aortopulmonary collateral arteries (MAPCAs); and Type IV: TOF with absent pulmonary valve syndrome. These types will be discussed individually.
Type I: Classic TOF
Classic TOF is a defect that was described in the introductory paragraph without any other major abnormalities such as pulmonary atresia or significant APC vessels.
Symptoms
Clinical presentation is largely dependent upon the degree of pulmonary outflow tract obstruction. When this obstruction is mild, symptoms may not be present until later in infancy and childhood. However, when the obstruction is severe, symptoms are exhibited in the neonatal period. In general, the infant is not cyanotic as a neonate, but develops cyanosis sometime between 2 and 6 months of life. Most typical methods of presentation are cardiac murmur detected on routine examination, bluish discoloration (cyanosis) noticed by the parents, nurse or a primary care physician, and hypercyanotic spells, although the latter are not common in the neonate. Because the hypercyanotic spells are not common during the newborn period, they will not be reviewed here, but the reader is referred to elsewhere4,6–8 and to Chapter 14 for further discussion on this subject.
Physical Examination
Central cyanosis is seen in nearly all cases of TOF. However, it should be understood that mild decrease in arterial saturation may not cause clinically discernible cyanosis, although recent use of pulse oximetry for detection of cyanotic CHD9 may uncover some of these babies. Clubbing of fingers and toes is not seen in the neonatal period and such changes take place after several months of arterial desaturation, RV impulse is prominent with a heave. A systolic thrill may be palpated at the left upper sternal border and/or in the suprasternal notch. The first heart sound is either normal or slightly increased in intensity and heard best at the apex. The second heart sound is heard best at the left upper sternal border and is single with no audible pulmonary component. A grade III to IV, long, ejection, systolic murmur is usually heard at the left upper sternal border and is secondary to turbulent blood flow across the stenotic RVOT. In contradistinction to PS with intact ventricular septum, the murmur of TOF becomes shorter and less intense with increasing severity of pulmonary outflow tract obstruction. During hypercyanotic spells, the systolic murmur becomes very soft or disappears altogether. A holosystolic murmur of VSD is heard at the left lower sternal border in infants with less severe and acyanotic types of TOF. Early diastolic murmurs are not auscultated in babies with classic TOF, although they may be heard in TOF with APV syndrome. Continuous murmurs associated with PDA are rarely appreciated. Signs of congestive heart failure (CHF) are notably absent.
Noninvasive Evaluation
Chest x-ray The size of the heart is either normal or minimally increased on chest roentgenogram. The apex of the heart may be uplifted and is thought to be a sign of RV hypertrophy and is described by some workers as “boot-shaped” heart. Concavity may be seen in the region of pulmonary conus, reflecting hypoplasia of the pulmonary outflow tract, although the presence of thymic shadow may make this assessment difficult. Pulmonary vascular markings are usually decreased. A right-sided aortic arch may be present (Figure 29.3). Whereas a right aortic arch is present in only 25% of TOF cases, the presence of a right aortic arch along with concave pulmonary segment and diminished pulmonary vascular markings in a chest x-ray makes the diagnosis of TOF almost certain.
Figure 29.3. Chest x-ray showing lifted up apex giving the appearance of boot shaped heart and right aortic arch, marked with arrows. Reproduced with permission from Neonatology Today 2011;6(5):1–10.
ECG Right axis deviation and RV hypertrophy (Figure 29.4) are usually seen in the ECG, although it is difficult to distinguish from normal RV preponderance of the neonate. Enlargement of the right atrium (RA) is less frequently seen.
Figure 29.4. ECG of a neonate with TOF showing right axis deviation and right atrial enlargement and RV hypertrophy.
Echocardiogram Echo-Doppler studies are useful in confirming the diagnosis. Large VSD, with aortic over-ride can easily be seen (Figure 29.5) along with the enlarged and hypertrophied RV. Shunting across the VSD, usually right to left (Figure 29.6) and RVOT obstruction (Figure 29.7) can be demonstrated. Increased Doppler flow velocity across the RVOT (Figure 29.8) can easily be shown. However, it should be recognized that (1) in the early neonatal period with normally high PA pressure, the Doppler gradients underestimate the degree of obstruction and (2) in extremely severe RVOT obstruction, the Doppler gradients are limited by the systemic arterial pressure and resistance. The size of the main pulmonary artery (MPA) and the proximal branch PA can be evaluated both by 2-dimensional (2D) and color flow imaging (see Figure 28.7), although the distal PAs are difficult to image by echo studies.
Figure 29.5. Selected video frame from a 2D echocardiogram in a precordial long axis view of neonate with TOF demonstrates a large VSD and an over-riding Ao. The RV is enlarged and hypertrophied (RVM). LA, left atrium; LV, left ventricle.
Figure 29.6. Color Doppler flow mapping of a similar echo frame as in Figure 29.5, demonstrating right-to-left shunt across the VSD—in blue flow. Other abbreviations are same as in Figure 29.5.
Figure 29.7. Selected video frame from a 2D echocardiogram in a precordial short axis view demonstrating a large Ao in the center with thickened PV leaflets and a small MPA to the left. The origins of the branch PAs are seen. B: Color Doppler flow mapping of an echo frame similar to (A) demonstrating color flow disturbance beginning proximal to the PV (arrow). The RPA and the LPA are more clearly seen on color. RV, right ventricle.
Figure 29.8. Increased Doppler flow velocity (3.9 m/s) across the RVOT demonstrating severe obstruction; the calculated gradient by modified Bernoulli equation is 60.7 mmHg.
Computed tomography (CT) and magnetic resonance imaging (MRI). These studies are not usually required in the neonate with typical TOF; interested reader is referred to Chapter 12 for further discussion on CT and MRI.
Cardiac Catheterization and Angiography
Cardiac catheterization and selective cineangiography are not routinely required because the echocardiographic and Doppler studies usually provide adequate data upon which decision regarding management may be made with reasonable accuracy. However, if noninvasive studies do not provide the data required for making decision for surgical management with reasonable certainty, cardiac catheterization and selective cineangiography may be performed. In addition, these procedures are integral parts of transcatheter balloon pulmonary valvuloplasty if such intervention is deemed helpful (see the section below).
Systemic venous O2 saturations are either normal or diminished depending on the degree of arterial desaturation; the latter is proportional to the degree of RVOT obstruction. Left-to-right shunts are usually not present. Pulmonary venous and left atrial saturations are generally normal. The LV and aortic saturations are decreased secondary to right-to-left shunt across the VSD. The O2 saturation in the Ao is a better indicator of the degree of desaturation than that in the LV because of better mixing distally.
The right atrial pressure is mildly increased. The peak systolic pressure in the RV is markedly increased and similar to that in the LV due to a large nonrestrictive VSD. The RV pressure curve is flat when compared to that in patients with PS with intact ventricular septum in which it is triangular. The PA pressures are low to normal with significant peak-to-peak systolic pressure gradient across the PV and infundibular region. Nevertheless, multiple gradients across multiple obstructions may not be seen on pressure pullback either because of technical (multiple holes in the catheter or rapid withdrawal) or secondary to physiologic reasons.10 Combined angiographic and pressure pullback data should be used for evaluation of degree and site of RV outflow obstruction. The pressures in the LV and the Ao are normal with no gradient across the aortic valve.
Selective cineangiography is an integral part of cardiac catheterization. Selective LV angiography in right anterior oblique (RAO) and left axial oblique (Figure 29.9) views are performed to show the size and function of the LV, the size and location of the VSD, and to exclude additional muscular VSDs. Selective RV angiography (sitting-up [15° left anterior oblique (LAO) and 35° cranial] and lateral views) demonstrates its size and function, and identifies any RV outflow obstruction (Figure 29.10). Pulmonary arteriogram may be performed in sitting-up and lateral views to show the size of the MPA and branch PA (Figure 29.11) and to exclude the branch PA stenosis. Aortic root angiography (not shown) is useful in visualizing coronary artery anatomy, particularly to exclude the coronary arteries crossing the RV infundibulum. It should be noted that origin of the left anterior descending coronary artery from the right coronary artery occurs in approximately 8% of TOF cases and when necessary it should be excluded by selective coronary angiography.
Figure 29.9. Selected frame from LV cineangiogram in a LAO view via a catheter (C) placed into it by advancing the catheter across the patent foramen ovale and mitral valve. A large subaortic VSD (thick arrow) is shown. Opacification of the Ao and brachiocephalic vessels (thin arrows) ensued. DAo, descending aorta.
Figure 29.10. Selected frame from RV cineangiogram in 15° LAO and 30° cranial projection demonstrating severe infundibular and valvar PS. The PA anatomy is also clearly demonstrated. Reproduced with permission from Neonatology Today 2011;6(5):1–10.
Figure 29.11. Selected frames from MPA cineangiogram demonstrate the size and continuity of the RPA and the LPA. C, catheter.
Management
TOF babies are initially managed similar to other cyanotic neonates, as reviewed in Chapters 13 and 28 and will not be reviewed in this chapter. Cyanotic (Tet) spells are rare in the newborn period. However, if they do happen, the management is as described elsewhere4,6–8,11 and is described in Chapter 14.
The objective of management of classic TOF patients is to undertake complete surgical correction with negligible mortality and morbidity. Most of the classic TOF patients either do not have cyanosis or have minimal cyanosis during the neonatal period, and do not need surgery at that juncture. They should be followed periodically and surgical repair may be performed electively sometime between 6 to 12 months of age. Total surgical correction involves closing the VSD to allow LV output directly into the Ao and resection of the infundibular obstruction, and/or relief of pulmonary valvar obstruction. Since the corrective surgery is usually performed beyond neonatal period, no further discussion regarding corrective surgery will be included in this chapter.
In some babies, the PS is very severe causing significant hypoxemia. If total surgical corrective procedure is not feasible because of hypoplasia of the PV annulus or the PA, small LV, and/or anomalous course of a coronary artery across the RV infundibulum, palliative surgery to augment pulmonary blood flow (PBF) may be performed to relieve hypoxemia and to allow the baby to grow, when complete correction may be undertaken more safely. Subclavian artery to ipsilateral PA shunt, described by Blalock and Taussig in 194512 was modified by de Leval and his colleagues13; they placed an interposition Gore-Tex graft between the right or left subclavian artery and the ipsilateral PA (Figure 29.12). Most surgeons prefer this modified Blalock-Taussig (BT) shunt or a variation thereof. Prostaglandin E1 (PGE1) may be infused to open the ductus while awaiting surgery.
Figure 29.12. Selected frame from Gore-Tex graft (BT shunt) cineangiogram demonstrating a patent shunt with good opacification RPA and LPA. Reproduced with permission from Neonatology Today 2011;6(5):1–10.
Though rare, some babies have predominant obstruction at the PV level. These babies may be palliated by balloon pulmonary valvuloplasty14–17; the procedure increases the PBF and improves systemic hypoxemia. Such procedures14–17 initially augment PBF and subsequently allow growth and development of the PA system and the LV so that a total surgical correction could be undertaken at a later time with a greater chance for success.14–17 For further discussion of the indications, technique, and results the reader is referred to our prior publications14–17 and to Chapter 20 of this book.
Type II: TOF with Pulmonary Atresia
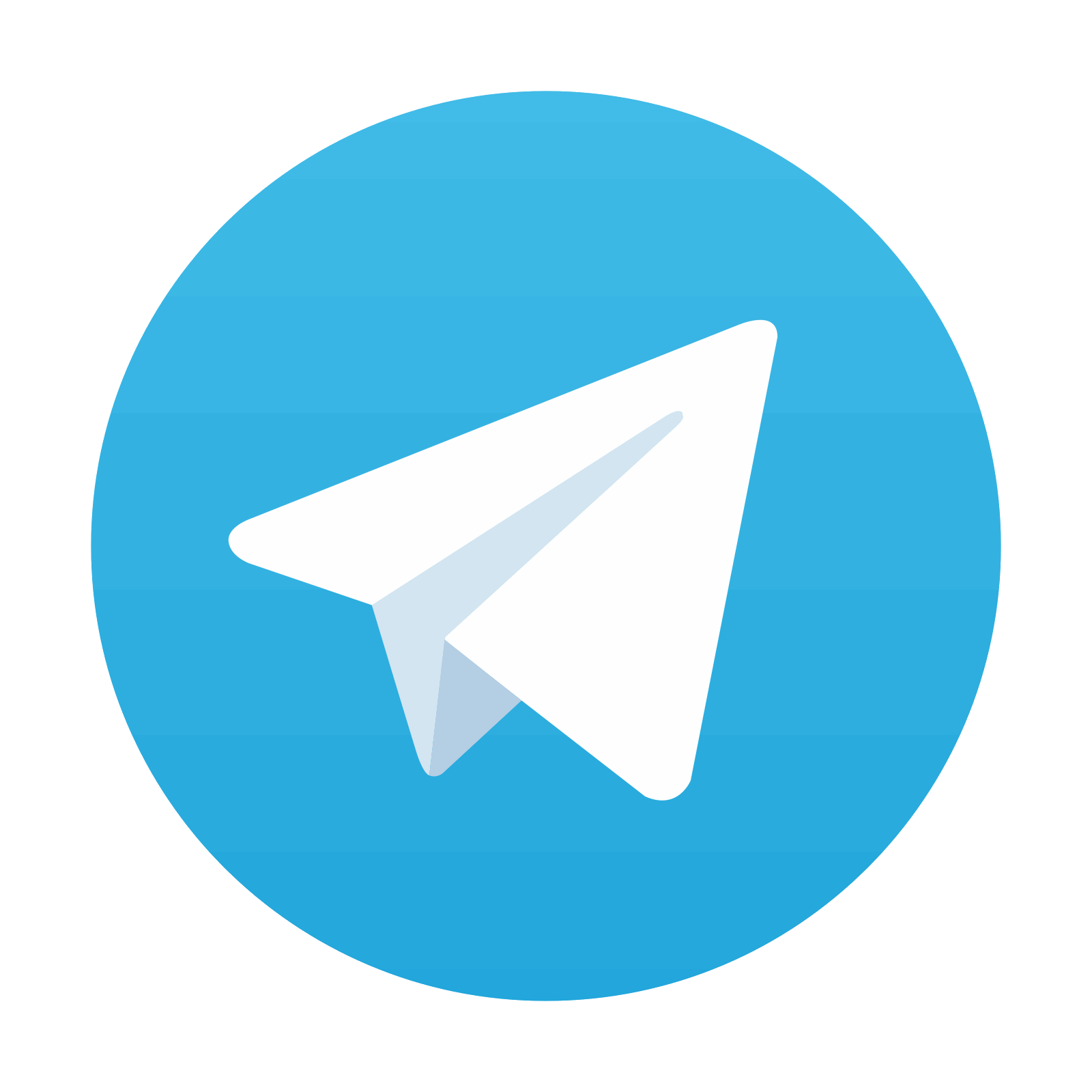
Stay updated, free articles. Join our Telegram channel

Full access? Get Clinical Tree
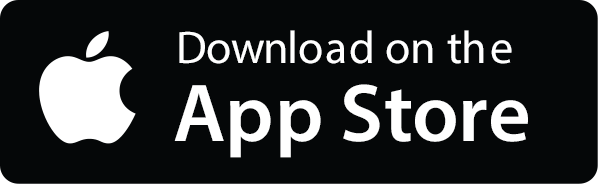
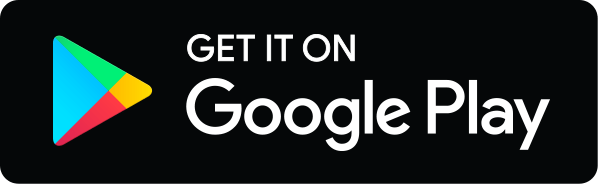