Background
Substantial variability in sequential echocardiographic right ventricular (RV) quantification may exist. Interobserver and intraobserver values are well known, but acquisition (test-retest) variability has been rarely assessed. The objective of this study was to determine the test-retest variability of sequential RV volume and ejection fraction (EF) measurements by real-time three-dimensional echocardiography in patients with congenital heart disease and healthy controls.
Methods
Twenty-eight participants (21 patients with congenital heart disease, seven healthy controls; mean age, 30 ± 14 years; 43% men) underwent a series of three echocardiographic studies. To obtain interobserver and intraobserver test-retest variability, two sonographers acquired sequential RV data sets in each participant during one outpatient visit. RV volumetric quantification was done using semiautomated three-dimensional border detection. The variability data were analyzed using correlation coefficients, Bland-Altman analysis, and coefficients of variation.
Results
Absolute mean differences for sequential intraobserver acquisitions were 12 ± 12 mL for end-diastolic volume, 7 ± 6 mL for end-systolic volume, and 4 ± 3% for EF. Interobserver and intraobserver test-retest variability, respectively, were 7% and 7% for RV end-diastolic volume, 14% and 7% for end-systolic volume, and 8% and 6% for EF.
Conclusions
Good test-retest variability, besides the practical nature of real-time three-dimensional echocardiography for RV volume and EF assessment, makes it a valuable technique for serial follow-up. Although it may be challenging to diminish all factors that can influence echocardiographic examination for serial follow-up, standardization of RV size and functional measurements should be a goal to produce more interchangeable data.
Right ventricular (RV) volumes and ejection fraction (EF) are important factors for the initiation of therapy and assessment of response to treatment in patients with congenital heart disease (CHD), and therefore, RV volumes and EF are tested sequentially in everyday clinical practice. Subjective visual evaluation of RV function may be appropriate for single assessment, but it is insufficiently reliable for sequential use. Longitudinal RV function can more accurately be determined by measuring tricuspid annular plane systolic excursion or the myocardial performance index. Quantification of RV dimensions and function can be done by two-dimensional echocardiography, although the value of the estimations of RV volumes and EF is limited because of the need for geometric assumptions that are especially not true for the abnormal hearts that are of interest. Consequently, cardiac magnetic resonance (CMR) imaging has become the indicated technique for RV volume and EF assessment, even though the cost and availability of CMR may hamper its use in routine clinical practice.
Real-time three-dimensional (3D) echocardiography (RT3DE) is an alternative imaging modality for quantification of RV volumes and EF. In various studies, improved accuracy and interobserver and intraobserver values of RT3DE compared with two-dimensional echocardiography in patients with CHD have been shown. However, these interobserver and intraobserver values reflect only the variability caused by variations in analysis of the same data set. Another source of variability is the difference in the acquisition of the data sets, the test-retest variability between data sets that are acquired and subsequently analyzed. To what extent this affects patients with CHD with abnormally shaped right ventricles is unknown. Therefore, the objective of this study was to determine the test-retest variability of RT3DE for RV volumes and EF.
Methods
Study Design
We prospectively recruited 33 participants (21 patients with complex and/or surgically repaired CHD and 12 healthy controls) in sinus rhythm, who all underwent RT3DE. The patients were referred to the echocardiography laboratory for routine measurements of their cardiac function and had sufficient acoustic windows. The healthy controls were employees of the university or the hospital who had no medical histories or current symptoms suggestive of cardiovascular disease, including hypertension and/or systemic illnesses with potential cardiovascular components, such as diabetes or thyroid disease. The medical ethics committee approved the study, and informed consent was obtained from all patients and healthy controls.
To assess interobserver and intraobserver test-retest variability in a way that closely reflects everyday clinical practice, the following schedule was applied ( Figure 1 ). Sonographer 1 (J.S.M.) acquired an RV real-time 3D echocardiographic data set, after which sonographer 2 (E.J.A.W.-G.) independently acquired a data set using the same machine. Thereafter, sonographer 1 acquired a second data set. In this manner, RV data sets were obtained independently by two sonographers with various levels of experience regarding 3D echocardiographic assessment of the right ventricle (4 and 2 years, respectively), who were blinded to each other’s results. After the acquisitions, they analyzed their own data sets in a blinded fashion, resulting in interobserver and intraobserver test-retest variability. Furthermore, interobserver variability was determined by having all data sets that were acquired by sonographer 2 also analyzed by sonographer 1.

Acquisition and Analysis by RT3DE
Real-time 3D echocardiographic harmonic imaging was performed using the iE33 ultrasound system (Philips Medical Systems, Best, The Netherlands) equipped with an X3-1 matrix-array transducer with the patient in the left lateral decubitus position. A full-volume scan was acquired from a modified apical transducer position in harmonic mode from seven R-wave-gated subvolumes during a single end-expiratory breath-hold. The depth and angle of the ultrasound sector were adjusted to the minimal level still encompassing the right ventricle. Before each acquisition, images were optimized for endocardial border visualization by modifying time gain and compression and increasing the overall gain. The mean volume rate was 29 frames/cardiac cycle (range, 20–45 frames/cardiac cycle). The data sets were digitally exported to a TomTec server (TomTec Imaging Systems, Unterschleissheim, Germany) connected to a terminal workstation for further analyses.
The digital real-time 3D echocardiographic RV data sets were analyzed offline using TomTec’s 4D RV Function version 1.2. The data sets were judged for image quality: they should include an analyzable RV apex, lateral wall, and tricuspid valve area. The software performs 3D semiautomated border detection of RV volumes over one cardiac cycle. It uses a physics-based modeling algorithm that makes no assumptions regarding RV geometry. The function of the TomTec analysis program is reported in detail elsewhere. In short, the end-diastolic (largest RV volume) and end-systolic (smallest RV volume) phases need to be identified. Endocardial border contours are drawn onto still frames of the apical four-chamber view, short-axis view, and coronal view in both end-diastole and end-systole ( Figure 2 ). After the steps followed for contour tracing, the software automatically delineates the RV endocardial border from the end-diastolic and end-systolic phases. By sequential analysis, the software creates an RV mathematic dynamic 3D endocardial surface that represents changes in the RV cavity over the cardiac cycle. From this 3D endocardial surface, global RV volumes and EF are calculated.

Statistical Analysis
Statistical analysis was performed using SPSS version 15.0 (SPSS, Inc., Chicago, IL). Categorical data are summarized as numbers and percentages, and continuous data are presented as mean ± SD. Agreement between the sequential acquisitions was evaluated by linear regression analysis with Pearson’s correlation coefficient. The Z -statistic was used to evaluate differences between two correlation coefficients. Furthermore, the agreement was evaluated using Bland-Altman analysis by calculating the bias (mean difference) and the 95% limits of agreement (2 standard deviations around the mean difference). Paired t tests were used to analyze the significance of the biases in volumes and EF between sequential acquisitions. Moreover, the coefficients of variation were defined as the standard deviation of the difference between the two readings (or readers) divided by their mean value times 100. All statistical tests were two sided, and P values < .05 were considered statistically significant.
Results
Of the original 33 participants, five were excluded from analysis for echocardiographic images of insufficient quality (four because of poor acoustic windows and one because of the inability to include the RV lateral wall and apex). The baseline characteristics of 28 participants (mean age, 30 ± 14 years; 43% men) are listed in Table 1 . The patients who were included had the following types of CHD: atrial septal defect ( n = 5), tetralogy of Fallot ( n = 3), aortic valve pathology ( n = 5), pulmonary stenosis with or without ventricular septal defect ( n = 2), double-outlet right ventricle ( n = 1), ventricular septal defect ( n = 1), arterial switch for transposition of the great arteries ( n = 1), coarctation of the aorta ( n = 1), mitral valve insufficiency ( n = 1), and patent arterial duct ( n = 1). The heart rates did not change significantly over the various examinations.
Variable | Value |
---|---|
Men | 43% |
Age (y) | 30 ± 14 |
Heart rate (beats/min) | 60 ± 10 |
Height (cm) | 172 ± 10 |
Weight (kg) | 67 ± 8 |
Body surface area (m 2 ) | 1.8 ± 0.1 |
Specific characteristics of patients | |
Age at initial repair (y) ( n = 16) | 10 ± 19 |
Right-sided CHD | 11 |
Left-sided CHD | 10 |
The RV volumes and EF obtained by the two sonographers are displayed in Table 2 . Measurements on the sequential data sets by one sonographer were not different, while the end-diastolic volume measurements by the second sonographer differed from those obtained by the first sonographer ( P = .017).
Sonographer 1 | Sonographer 2 | ||||
---|---|---|---|---|---|
Variable | Acquisition 1 | Acquisition 2 | P ∗ | Acquisition 1 | P † |
End-diastolic volume (mL) | 183 ± 43 | 180 ± 37 | .36 | 173 ± 39 | .017 |
End-systolic volume (mL) | 88 ± 30 | 86 ± 23 | .79 | 83 ± 28 | .076 |
Stroke volume (mL) | 95 ± 22 | 94 ± 19 | .41 | 91 ± 19 | .14 |
EF (%) | 53 ± 7 | 53 ± 6 | .70 | 53 ± 7 | .68 |
∗ Paired t test for sequential measurements recorded by one sonographer.
† Paired t test for sequential measurements between two sonographers.
Interobserver Test-Retest Variability
The Z -statistic revealed no differences in the strength of the correlations found by sequential acquisitions and the corresponding measurements between sonographers versus correlations found by sequential acquisitions and measurements by one sonographer. The results of linear regression and Bland-Altman analysis for the assessment of interobserver test-retest variability are displayed in Figure 3 . For RV end-diastolic volume, end-systolic volume, and EF, interobserver test-retest variability was 7%, 14%, and 8%, respectively ( Table 3 ).
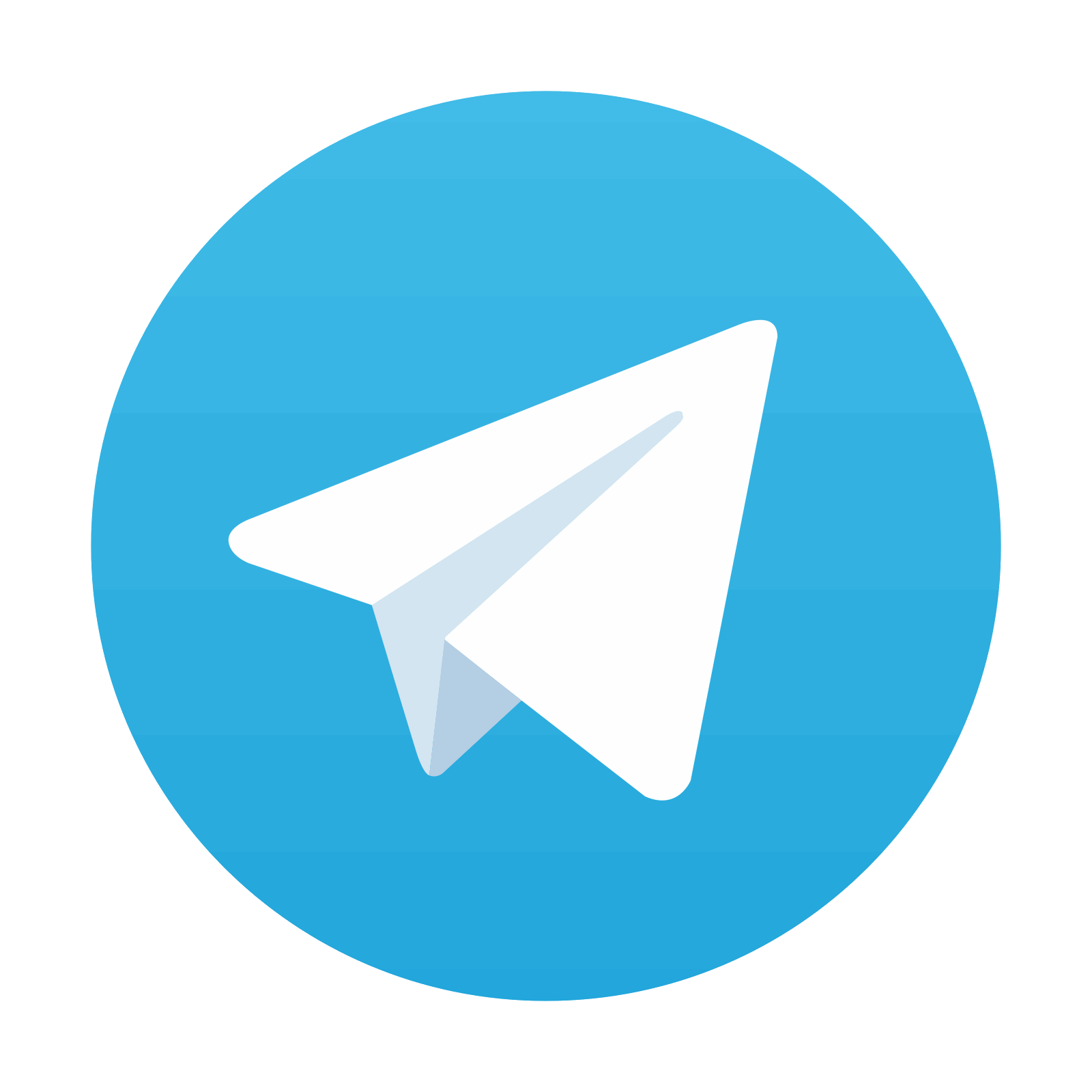
Stay updated, free articles. Join our Telegram channel

Full access? Get Clinical Tree
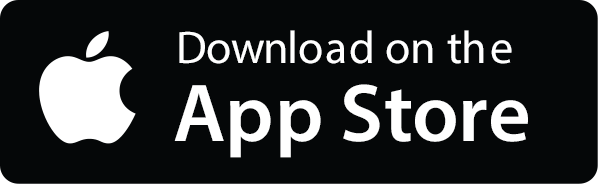
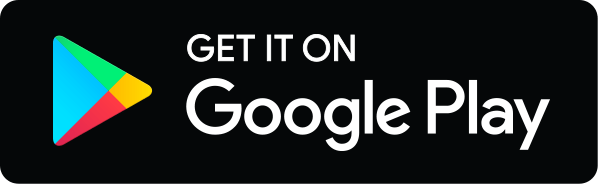
