A number of ventricular assist devices (VADs) are available for acute circulatory support. As opposed to long-term VADs, which are designed primarily for bridge to transplantation or long-term support in the nontransplant patient, temporary VADs are designed to reestablish adequate organ perfusion rapidly. Patients in cardiogenic shock require early aggressive therapy. Despite relief of ischemia, inotropic drugs, and control of cardiac rhythm, some patients remain hemodynamically unstable and require some type of mechanical circulatory support in order to restore a normal cardiac output and maintain end-organ perfusion. Cardiogenic shock occurs in 2.4 to 12% of patients with acute myocardial infarction (AMI).1 The landmark Should We Emergently Revascularize Occluded Coronaries for Cardiogenic Shock (SHOCK) trial demonstrated mortality of greater than 50% despite revascularization.2 If instituted promptly, temporary mechanical support leads to improved survival in this group of patients.3 The need for circulatory support in the postcardiotomy period is relatively low and has been estimated to be in the range of 0.2 to 0.6%,4 but when it occurs, it needs to be managed effectively if the patient is to be salvaged. Additional indications for acute VADs are in chronic heart failure patients who suffer cardiovascular collapse, and severe cases of myocarditis and postpartum cardiomyopathy.
All practicing cardiac surgeons should have an understanding of current devices, and have at least one of these support systems available. Studies show that even smaller facilities that do not have advanced heart failure programs can have improved patient survival if a device can be implemented rapidly and the patient is transferred to a tertiary care facility with expanded capabilities.5
This chapter describes the devices currently available, indications for support, patient management, and the overall morbidity and mortality associated with temporary mechanical support. In addition, it describes some of the more promising devices that have just received approval or are currently undergoing trial. The goal of all temporary assist devices is to alleviate shock and establish an environment in which the native heart and end organs can recover. If recovery is unlikely, then a bridge to a long-term device, typically for bridging to a heart transplant, may be the best strategy. Rarely should individuals be supported directly to heart transplantation from an acute support device, because the newer generations of long-term devices typically provide more reliable support and allow for better rehabilitation in an out-of-hospital setting. It may be possible to bridge a patient who is not a transplant candidate to a long-term device, but given that these patients often are older with comorbities, bridging a patient from an acute VAD to a long-term DT pump must be done with caution.
Intra-aortic balloon pumps (IABPs) are often the first line of mechanical assistance utilized for patients in cardiogenic shock. The concept of increasing coronary blood flow by counterpulsation was demonstrated by Kantrowitz and Kantrowitz in 1953 in a canine preparation and again by Kantrowitz and McKinnon in 1958 using an electrically stimulated muscle wrap around the descending thoracic aorta to increase diastolic aortic pressure.6 In 1961, Clauss and colleagues used an external counterpulsation system synchronized to the heartbeat to withdraw blood from the femoral artery during systole and reinfuse it during diastole.7,8 One year later, Moulopoulos and colleagues produced an inflatable latex balloon that was inserted into the descending thoracic aorta through the femoral artery and inflated with carbon dioxide.8 Inflation and deflation were synchronized to the electrocardiogram to produce counterpulsation that reduced end-systolic arterial pressure and increased diastolic pressure. In 1968, Kantrowitz reported survival of one of three patients with postinfarction cardiogenic shock refractory to medical therapy using an IABP.9 These pioneering studies introduced the concept of supporting the failing circulation by mechanical means.
The major physiologic effects of the IABP are a concomitant reduction in left ventricular afterload along with an increase in coronary perfusion pressure secondary to an increase in aortic diastolic pressure.10 Important related effects include reduction in left ventricular systolic wall tension and oxygen consumption, reduction in left ventricular end-systolic and end-diastolic volumes, reduced preload, and an increase in coronary artery and collateral vessel blood flow.11 Cardiac output increases because of improved myocardial contractility owing to increased coronary blood flow and the reduced afterload and preload, but the IABP does not directly move or significantly redistribute blood flow.12 IABP counterpulsation reduces peak systolic wall stress (afterload) by 14 to 19% and left ventricular systolic pressure by approximately 15%.12 Because peak systolic wall stress is related directly to myocardial oxygen consumption, myocardial oxygen requirements are reduced proportionately. As measured by echocardiography and color-flow Doppler mapping, peak diastolic flow velocity increases by 117% and the coronary flow velocity integral increases by 87% with counterpulsation.13 Experimentally, collateral blood flow to ischemic areas increases up to 21% at mean arterial pressures greater than 90 mm Hg.14
Several variables affect the physiologic performance of the IABP. The position of the balloon should be just downstream of the left subclavian artery (Fig. 18-1). Diastolic augmentation of coronary blood flow increases with proximity to the aortic valve.15 The balloon should fit the aorta so that inflation nearly occludes the vessel. Experimental work indicates that for adults, balloon volumes of 30 or 40 mL significantly improve both left ventricular unloading and diastolic coronary perfusion pressure when compared with smaller volumes. Inflation should be timed to coincide with closure of the aortic valve, which for clinical purposes occurs at the dicrotic notch of the aortic blood pressure trace (Fig. 18-2). Early inflation reduces stroke volume, increases ventricular end-systolic and -diastolic volumes, and increases both afterload and preload. Diastolic counterpulsation is visualized easily as a pressure curve in the arterial waveform and indicates increased diastolic perfusion of the coronary vessels (and/or bypass grafts).16 Deflation should occur as late as possible to maintain the duration of the augmented diastolic blood pressure but before the aortic valve opens and the ventricle ejects. For practical purposes, deflation is timed to occur with the onset of the electrocardiographic R wave. Active deflation of the balloon creates a suction effect that acts to decrease left ventricular afterload (and therefore myocardial oxygen consumption).
FIGURE 18-2
Illustration showing the effect of the intra-aortic balloon on aortic pressure. After ejection produces the pulse (A), inflation of the balloon increases aortic diastolic pressure (B). At end diastole, sudden deflation reduces aortic end-diastolic pressure (C) below that of an unassisted beat and reduces afterload and myocardial oxygen demand.

Physiologic factors that influence the in situ hemodynamic performance of the IABP include heart rate and rhythm, mean arterial diastolic pressure, competence of the aortic valve, and compliance of the aortic wall. Severe aortic regurgitation is a contraindication to use of the IABP as a very low mean aortic diastolic pressures reduce aortic root pressure augmentation and coronary blood flow. A calcified, noncompliant aorta increases diastolic pressure augmentation but risks injury to the aortic wall. On the other hand, young patients with a highly elastic, compliant aorta may manifest decreased diastolic pressure augmentation.
For an IABP to perform optimally, a regular heart rate with an easily identified R wave or a good arterial pulse tracing with a discrete aortic dicrotic notch is required. Current balloon pumps trigger off the electrocardiographic R wave or the arterial pressure tracing. Both inflation and deflation are adjustable, and operators attempt to time inflation to coincide with closure of the aortic valve and descent of the R wave. During tachycardia, the IABP usually is timed to inflate every other beat; during chaotic rhythms, the device is timed to inflate in an asynchronous fixed mode that may or may not produce a mean decrease in afterload and an increase in preload. In unstable patients, every effort is made to establish a regular rhythm, including a paced rhythm, so that the IABP can be timed properly. The newer-generation IABP consoles come with algorithms that select the most appropriate trigger mode and time inflation and deflation automatically which have been further enhanced by fiber-optic transducers at the tip of the IABP which do not require a conventional fluid-filled transducer to accurately reflect arterial pressure and are more easily timed.17
The traditional indications for insertion of the IABP are cardiogenic shock, uncontrolled myocardial ischemic pain, and postcardiotomy low cardiac output.18 Additional indications for IABP have broadened to include patients with high-grade left main coronary artery stenosis, high-risk or failed percutaneous transluminal coronary angioplasty, atherectomy, or stents; patients with poorly controlled ventricular arrhythmias before or after operation; and patients with postinfarction ventricular septal defect (VSD) or acute mitral insufficiency after myocardial infarction (MI).19 Occasionally an IABP is used prophylactically in high-risk patients with poor left ventricular function (LVF) with either mitral regurgitation or preoperative low cardiac output owing to hibernating or stunned myocardium. These patients may benefit from temporary afterload reduction in the prebypass period and during weaning from cardiopulmonary bypass.
The IABP usually is inserted into the common femoral artery either by the percutaneous technique or surgical cut down. A cut down is used most often during cardiopulmonary bypass when the pulse is absent. To avoid a formal cut down, a femoral artery catheter may be inserted in anticipation of IABP use in patients undergoing complex procedures who have myocardial dysfunction .The superficial femoral artery is avoided because of its smaller size and increased possibility of leg ischemia. For patients with small vessels, a 7-French catheter without a sheath is recommended to lessen the possibility of leg ischemia. The iliac and axillary arteries and, very rarely, the abdominal aorta are infrequently used alternate sites.20,21 Direct insertion into the ascending aorta can be used intraoperatively in patients with severe aortoiliac or femoral occlusive disease that prevents passage of the balloon catheter.22,23
An IABP is inserted using Seldinger’s technique. In the catheterization laboratory, both the guidewire and balloon are monitored by fluoroscopy, but this is not essential if not readily available. In the operating room, the guidewire and balloon are typically placed and positioned under transesophageal echocardiographic guidance.24 The catheter can be inserted without the sheath when the size of the common femoral artery is a concern.25
Typically patients with an IABP receive heparin for anticoagulation, but this practice varies from center to center. The exit site of the catheter must be kept clean with antiseptics and covered in an effort to prevent local infection or septicemia.
A percutaneous IABP can be removed without exposing the femoral puncture site. The exit site is prepped and securing sutures are cut. The balloon catheter is disconnected from the pump and completely deflated using a 50-mL syringe. When the balloon is removed, the proximal artery is compressed and retrograde bleeding is allowed, which flushes any distal clot into the wound. The distal femoral artery then is compressed, and antegrade flushing then is allowed. Finally, steady nonocclusive pressure is held over the femoral puncture site. Pressure is maintained over the puncture site for 30 minutes to ensure that thrombus closes the hole. If the balloon is inserted via a cut down, the balloon preferably is removed in the operating room. The puncture site is closed with sutures. If blood flow to the lower limb is impaired after removal, a formal thromboembolectomy is required.
If the percutaneous needle punctures the iliac artery above the inguinal ligament intentionally or inadvertently in obese individuals, removal should be done through a surgical incision in the operating room because the backward slope of the pelvis makes pressure difficult to maintain after withdrawal, and substantial occult retroperitoneal bleeding may occur.
An IABP may be useful before insertion of more long-term left ventricular assist systems to optimize perfusion and right ventricular function. Use of an IABP in patients with chronic systolic heart failure as a bridge to transplantation or left ventricular assist device (LVAD) therapy is a common practice with over half of the patients being stabilized with this strategy.26 More importantly, placement of the IABP through a graft sewn to the subclavian artery is a strategy that has allowed ambulation of the patients while waiting for the next stage of therapy with a reported success rate of 93%.27
In this technique, a 6-mm graft is sewn to the artery in an end-to-side manner and the shortened standard IABP sheath is inserted into this graft.27 The IABP is then placed through a separate stab wound, or through the externalized IABP sheath and then positioned under fluoroscopy and transesophageal echocardiography (TEE). The wound over the sheath is then closed. Some groups bury the hub of the IABP sheath, but our preference is to externalize it so that if the IABP needs to be repositioned, the wound does not need to be reopened.
In patients waiting for a new heart, the presence of an IABP qualifies a patient as 1A, or the highest status, on the UNOS heart transplant waiting list.
Reported complication rates of the IABP vary between 12.9 and 29% and average approximately 20%.28 Life-threatening complications are rare. Leg ischemia is by far the most common complication (incidence 9-25%); other complications include balloon rupture, thrombosis within the balloon, septicemia, infection at the insertion site, bleeding, false aneurysm formation, lymph fistula, lymphocele, and femoral neuropathy.29 There is no significant difference in limb ischemia in the different types of IABPs clinically available.28,30
Balloon rupture occurs in approximately 1.7% of patients and usually is manifested by the appearance of blood within the balloon catheter and only occasionally by the pump alarm. Rupture may be slightly more common with transaortic insertion. Although helium usually is used to inflate the balloon, gas embolism has not been a problem. If rupture occurs, the balloon should be deflated forcibly to minimize thrombus formation within the balloon and should be promptly removed. If the patient is IABP dependent, a guidewire is introduced through the ruptured balloon, the original balloon is removed, and a second balloon catheter is inserted over the wire. If the ruptured balloon is not removed easily, a second balloon is inserted via the opposite femoral or iliac artery or through the axillary artery to maintain circulatory support.
Removal of a kinked or thrombosed ruptured balloon that cannot be withdrawn easily requires operative removal as forcibly removing a ruptured IABP which contains clot can disrupt the iliac or femoral artery upon removal and lead to uncontrollable hemorrhage. The catheter should be withdrawn as far as possible until resistance is met. The location of the tip should be determined by x-ray or ultrasound, and an incision should be planned to expose that segment of the vascular system. The trapped balloon is removed through an arteriotomy after control of the vascular segment is obtained.
Although the incidence of clinically significant lower leg ischemia varies in from 9 to 25% of patients, up to 47% patients have evidence of ischemia during the time the IABP is used.29 Thus, the preinsertion status of the pedal pulses should be determined and recorded before the IABP is inserted in every patient. After insertion, the circulation of the foot is followed hourly by palpating pulses or Doppler ultrasound. Foot color, mottling, temperature, and capillary refill are observed. The appearance of pain, decreased sensation, and compromised circulation indicate severe ischemia that requires restoration of the circulation to the extremity as soon as possible. There are three alternatives. If the patient is not balloon dependent, the balloon is removed immediately. In the majority of patients, this relieves the distal ischemia; a few patients require surgical exploration of the puncture site, removal of thrombus and/or emboli, and reconstruction of the femoral artery. If the patient is balloon dependent, a second balloon catheter can be introduced into the opposite femoral or iliac artery and the first is removed. Several risk factors for development of leg ischemia have emerged. Female gender, peripheral vascular disease, diabetes, cigarette smoking, advanced age, obesity, and cardiogenic shock are reported to increase the risk of ischemic complications after IABP. Because the IABP is inserted for compelling indications, identification of risk factors does not influence management, except to encourage removal of the device as soon as the cardiac status of the patient allows. In some series, longer duration of IABP counterpulsation is associated with an increased risk of complications.29 Although most ischemic complications are the result of impairment of arterial inflow, severe atherosclerotic diseases of the descending thoracic aorta may produce embolization of atherosclerotic material. Approximately 1% of patients develop false aneurysms at the femoral puncture site either in the hospital or shortly after discharge, and rare patients develop an arteriovenous fistula. Both conditions are confirmed readily by duplex scanning.
Very few complications of IABP cause death. Rare instances of bleeding (retroperitoneal or aortic), septicemia, central nervous system injury, or aortic dissection may cause or contribute to a patient’s death. Mortality is higher in patients with ischemic leg complications than in those without this complication.
Without revascularization, IABP produces a marginal increase in survival, but with revascularization, both short- and long-term survival, as well as quality of life have been shown to be improved.31 However, mortality is high in patients who receive an IABP because of the cardiac problems that led to the need for the device. Overall hospital mortality ranges from 26 to 50%.32,33 Risk factors for hospital mortality include advanced age, female gender, high New York Heart Association (NYHA) class, preoperative nitroglycerin, operative or postoperative insertion, and transaortic insertion in one study and age and diabetes mellitus in another. A third study correlates hospital death with AMI, ejection fraction of less than 30%, NYHA class IV, and prolonged aortic cross-clamp and bypass times.34 Time of insertion affects hospital mortality. Preoperative insertion is associated with a mortality of 18.8 to 19.6%.18 Mortality for intraoperative insertion is 27.6 to 32.3%.17 Postoperative IABO insertion is associated with a mortality of 39 to 40.5%. Mortality is highest at 68% for patients with pump failure, lowest at 34% for patients with coronary ischemia, and 48% for patients who had a cardiac operation. Long-term survival varies with the type of operation and is highest in patients who had cardiac transplantation or myocardial revascularization.18 Patients who received an IABP and required valve surgery with or without revascularization have a poorer prognosis. Creswell and colleagues found 58.8% of all patients alive at 1 year and 47.2% alive at 5 years. Naunheim and associates found that nearly all survivors were in NYHA class I or II.34 Approximately 18% of hospital survivors have some symptoms of lower extremity ischemia.
Given the overall ease of IABP insertion, excellent physiologic augmentation of coronary blood flow and left ventricular unloading, an IABP should be considered as the first line of mechanical support in patients who do not have significant peripheral vascular disease. There is some suggestion that preoperative prophylactic IABP insertion in high-risk patients (eg, left ventricular ejection fraction [LVEF] of less than 40%, unstable angina, left main stenosis of greater than 70%, or redo coronary artery bypass grafting [CABG]) can improve cardiac index, length of intensive care unit (ICU) stay, and reduce mortality.35 However, with meticulous myocardial protection and the judicious use of inotropes, such as epinephrine and milrinone, most groups experienced in dealing with such high-risk patients do not find routine IABP insertion helpful.
According to latest 2013 ACC/AHA guidelines on ST elevation myocardial infarction (STEMI), use of MCS devices (including IABP) is reasonable in patients with STEMI who are hemodynamically unstable and require urgent CABG (Class IIa recommendation).36 Prior to this, 2004 ACC/AHA and 2010 ESC STEMI guidelines listed specifically IABP use in cardiogenic shock as class 1B and IC recommendations, respectively, although there were no randomized trials supporting those recommendations. The IABP SHOCK II trial was a recent randomized trial of 600 patients who presented with AMI and cardiogenic shock who were to be treated with revascularization strategies. Three hundred patients received an IABP and 298 patients were treated medically. The degree of shock, and patient characteristics were similar. In this trial, there was no difference in the 30-day mortality (IABP 39.7%: control 41.3%)37 and also no differences in adverse events, leading to downgrading in guideline recommendation class.38
Many surgeons consider prophylactic insertion of IABP before high-risk cardiac surgery. Although the impact of this strategy is debatable, a recent meta-analysis of randomized-controlled trials has demonstrated that in high-risk patients, prophylactic IABP insertion leads to decrease in mortality, reduces the incidence of low output cardiac syndrome and translates into shorter ICU length of stay.39 Application of IABP in postcardiac surgery varies widely. Most surgeons consider this as the first line of mechanical circulatory support in situations of persistent hypotension or low cardiac output in the immediate postoperative phase. Again, in postcardiotomy situations, in presence of persistent low cardiac output requiring high-dose pressor and inotropic support, the impact of IABP is limited and consideration for devices that can significantly increase flow should be given.
The standard IABP has a volume of 40 cc. A 50-cc IABP has been introduced and studies show that it leads to higher augmented diastolic pressure, greater systolic unloading, and a greater reduction of pulmonary capillary wedge pressure.40
While an IABP can be helpful in the management of shock patients, it is important to understand that it is not the final therapy in terms of mechanical support for the failing heart. If the shock state persists, as evidence by a depressed cardiac index, then some form of direct mechanical support must be implemented so as to restore adequate end-organ perfusion. Failure to adequately treat a patient in cardiogenic shock will most assuredly result in the patient’s demise.
The need for acute cardiac support beyond cardiopulmonary bypass was clear from the early days of cardiac surgery. In 1966, the first successful use of a LVAD was reported after a double-valve operation by DeBakey, who used an assist device that was implanted in an extracorporeal location between the left atrium and the axillary artery.41 The patient was supported for 10 days on the pump, eventually was discharged home, and was a long-term survivor.41
The ideal acute support device should be capable of providing adequate flow, maximizing hemodynamics, and unloading the ventricle for patients of all sizes. Current devices have addressed the problem associated with variations in patient size by being designed as extracorporeal systems. Therefore, by virtue of having small-diameter cannulae transversing the chest, the pumps can support patients with varying body surface area. The disadvantages of such a system are the potential for driveline and mediastinal infections, as well as limiting patient mobility. In addition, the length of the cannula between the heart and the device, particularly the inflow cannula, predisposes to areas of stasis and potential thrombus generation. All current pumps require anticoagulation, which increases the ever-present threat of early postoperative bleeding. In addition, requirements for transfusion of large amounts of coagulation factors and platelets enhance the inflammatory response that is induced by surgery and is further perpetuated by the circuit. Activation of the contact and complement systems and the release of cytokines by leukocytes, endothelial cells, and macrophages further increase the potential negative and detrimental effects of use of temporary assist devices.44 The ensuing inflammatory cascade and volume overload can have detrimental effects on the pulmonary vascular resistance and lead to right ventricular overload, often necessitating the addition of a right ventricular assist device (RVAD).
Current temporary assist devices can be configured for biventricular support as needed, provided that the lungs can support oxygenation and ventilation. In cases of acute lung injury superimposed on circulatory failure, extracorporeal life support (ECLS) can be configured with conventional or the more recent centrifugal pumps.
The clinical scenarios that lead to the need for mechanical support all require that support be instituted in an expeditious manner. Therefore, all current devices must be easily implantable. In the postcardiotomy setting with access to the great vessels, the cannulae should allow the versatility of choosing any inflow or outflow site that is clinically indicated. In an active resuscitative setting, such as cardiac arrest in the catheterization laboratory, in which time is critical and transport to the operating room often impractical, percutaneous cannulation must be an option.
At present, the ideal device does not exist. Until innovations in the field of circulatory support lead to the development of an ideal device, the currently available technology must be tailored to the specific requirements of each patient, taking into consideration the duration of support needed.
A wide range of indications exist for acute mechanical support, the primary goal of all being rapid restoration of the circulation and stabilization of hemodynamics. The routine use of TEE has helped greatly in assessing the etiology of cardiogenic shock by allowing evaluation of ventricular function, regional wall motion abnormalities, and valvular mechanics. In a patient with mechanical complications secondary to MI such as acute rupture with tamponade, acute papillary muscle rupture, or postinfarction VSD, emergent surgical correction may obviate the need for device support. Similarly, in the postcardiotomy setting with failure to separate from cardiopulmonary bypass, TEE may direct the surgeon to the need for additional revascularization and reparative valve surgery and successful weaning from bypass.
If echocardiography fails to reveal a surgically correctable cause for cardiogenic shock, most surgeons use hemodynamic data to consider the need for mechanical assistance. These criteria include, but are not limited to, a cardiac index of less than 2.2 L/min/m2, systolic blood pressure of less than 90 mm Hg, mean pulmonary capillary wedge pressure or central venous pressure of greater than 20 mm Hg, and concomitant use of high doses of at least two inotropic agents.42 These situations may be associated clinically with arrhythmias, pulmonary edema, and oliguria. In such circumstances, use of an IABP may be considered as the first step. In the postcardiotomy setting, the preceding hemodynamic criteria, in absence of mechanical support, are associated with a greater than 50% chance of mortality.31 In this setting, some believe that earlier implantation of an assist device capable of supporting higher flows and allowing the heart to rest may improve results and allow for recovery of stunned myocardium.43 Furthermore, pharmacologic agents such as the phosphodiesterase inhibitor milrinone, nitric oxide, and vasopressin have helped to optimize hemodynamics during this critical initial period, reducing the need for concomitant right ventricular support.44,45
Once mechanical assistance has been instituted, the stabilized patient can undergo periodic evaluation to assess native heart recovery, end-organ function, and neurologic status. If appropriate, evaluation for cardiac transplantation ensues. Patients without malignancy, severe untreated infection, or neurologic deficit and who are not at an advanced age are selected for cardiac transplantation if all other criteria are met, and there is no sign of cardiac recovery. In this subgroup, we generally transition to a chronic VAD until an organ becomes available, a strategy that has been termed “a bridge to a bridge,” in that a short-term device is used to “bridge” the patient to a more durable “bridge to transplant” VAD. In patients with improvement in myocardial pump function, the devices may be weaned and removed.
Devices currently approved by the Food and Drug Administration (FDA) for temporary support include centrifugal pumps, roller pumps, venoarterial extracorporeal membrane oxygenation (ECLS, ECMO), the ABIOMED AB5000 Ventricle and Impella devices (ABIOMED, Danvers, MA), Thoratec Centrimag (Thoratec Inc., Pleasanton, CA), and the Thoratec paracorporeal VAD (pVAD) (Pleasanton, CA), and the TandemHeart system (CardiacAssist, Inc.). A number of other devices are undergoing investigation for short-term support.
Two types of pumps are available commercially for extracorporeal circulation: roller pumps and centrifugal pumps. In adults, roller pumps are used rarely, if ever, for temporary circulatory support beyond routine cardiopulmonary bypass applications because of important disadvantages. Although inexpensive, roller pumps are insensitive to line pressure and require unobstructed inflow. Additionally, roller pumps may cause spallation of tubing particles and are subject to tubing failure at unpredictable times. These systems require constant vigilance and are difficult to operate for extended periods. Use of roller pumps beyond 4 to 5 hours is associated with hemolysis and, for this reason, is inappropriate for mechanical assistance that may involve several days to weeks of support.46 Axial flow pumps, in which the pump rotor is parallel to the blood path, have entered the field of temporary support with the introduction of the Impella devices but most of the experience with temporary support devices has been with centrifugal pumps.
Centrifugal pumps are familiar assist systems because of their routine use in cardiopulmonary bypass. In these devices, the blood path is perpendicular to the rotor. Although many different pump-head designs are available, they all work on the principle of generating a rotary motion by virtue of moving blades, impellers, or concentric cones. These pumps generally can provide high flow rates with relatively modest increases in pressure. They require priming and de-airing prior to use in the circuit, and the amount of flow generated is sensitive to outflow resistance and filling pressures. The differences in design of the various commercially available pump heads are in the numbers of impellers, the shape and angle of the blades, and the priming volume. The Medtronic Bio-Pump® (Medtronic Bio-Medicus, Inc., Eden Prairie, MN), which is based on two concentric cones generating a rotary motion, has been extensively utilized in the field of acute circulatory support.47 The pump heads are disposable, relatively cheap to manufacture, and mounted on a magnetic motorized unit that generates the power that drives the pump. Although earlier designs caused mechanical trauma to the blood elements leading to excessive hemolysis, the newly engineered pumps are less traumatic and can be used for longer periods.
The Rotoflow (Maquet Cardiovascular) is another example of a centrifugal pump that is extensively utilized in the field of acute circulatory support.
Complications with temporary mechanical assistance are high and are very similar for patients on centrifugal pump support or ECLS. The major complications reported by a voluntary registry for temporary circulatory assistance using primarily LVADs, RVADs, and biventricular assist devices (BVADs) are bleeding, persistent shock, renal failure, infection, neurologic deficits, thrombosis and emboli, hemolysis, and technical problems. Neurologic deficits occurred in approximately 12% of patients, and in Golding’s experience, noncerebral emboli occurred equally often.48 Golding also found that 13% of patients also developed hepatic failure. An autopsy study found anatomical evidence of embolization in 63% of patients, even though none had emboli detected clinically.49
Although a meaningful comparison of results of centrifugal support from different institutions is not possible, in general, overall survival has been in the range of 21 to 41%. A voluntary registry reported the experience with 604 LVADs, 168 RVADs, and 507 BVADs; approximately 70% were with continuous-flow pumps and the remainder with pulsatile pumps.50 There were no significant differences in the percentage of patients weaned from circulatory assistance or the percentage discharged from the hospital according to the type of perfusion circuitry. Overall, 45.7% of patients were weaned, and 25.3% were discharged from the hospital.50 The registry also reports that long-term survival of patients weaned from circulatory support is 46% at 5 years.50 Most of the mortality occurs in the hospital before discharge or within 5 months of discharge.
Golding reported an identical hospital survival rate for 91 patients in 1992 using only centrifugal pumps, and Noon reported that 21% of 129 patients were discharged.49,51 Patients who received pulsatile circulatory assistance were supported significantly longer than those supported by centrifugal pumps, but there were no differences in the percentage of patients weaned or discharged. Survivors were supported an average of 3.1 days using continuous-flow pumps. Patients supported for AMI did poorly; only 11.5% survived to be discharged. Joyce reports that 42% of patients supported by Sarns impeller pumps eventually were discharged.52
By the 1960s, it was clear that cardiopulmonary bypass was not suitable for patients requiring circulatory support for several days to weeks. The development of ECLS as a temporary assist device (also referred to as extracorporeal membrane oxygenation [ECMO]) is a direct extension of the principles of cardiopulmonary bypass and follows the pioneering efforts of Bartlett and colleagues in demonstrating the efficacy of this technology in neonatal respiratory distress syndrome.53
There are a number of key differences between cardiopulmonary bypass and ECLS. The most obvious difference is the duration of required support. Whereas cardiopulmonary bypass typically is employed for several hours during cardiac surgery, ECLS is designed for longer duration of support. With ECLS, lower doses of heparin are used, and reversal of heparin is not an issue. Because a continuous circuit is used, areas of stasis, such as the cardiotomy suction and venous reservoir, are not present. These differences are thought to reduce the inflammatory response and the more pronounced coagulopathy that can be seen with cardiopulmonary bypass54 although there is generally a rapid rise of inflammatory cytokines with initiation of ECLS support.55
A typical ECLS circuit is demonstrated in Fig. 18-3. The system consists of the following:
FIGURE 18-3
Percutaneous extracorporeal membrane oxygenation (ECMO) support is attained via femoral vessel access. Right atrial blood is drained via a catheter inserted into the femoral vein and advanced into the right atrium. Oxygenated blood is perfused retrograde via the femoral artery. Distal femoral artery perfusion is not illustrated.

Hollow-fiber membrane oxygenator with an integrated heat-exchange system. The microporous membrane provides the necessary gas-transfer capability via the micropores where there is direct blood-gas interface with minimal resistance to diffusion. By virtue of the membranes being close to each other, the diffusion distance has been reduced without a significant pressure drop across the system. Control of oxygenation and ventilation is relatively easy. Increasing the total gas flow rate increases CO2 removal (increasing the “sweep”) by reducing the gas-phase CO2 partial pressure and promoting diffusion. Blood oxygenation is controlled simply by changing the fraction of O2 in the gas supplied to the oxygenator.
Centrifugal pump. These pumps are totally nonocclusive and afterload dependent. An increase in downstream resistance, such as significant hypertension, will decrease forward flow to the body. Therefore, flow is not determined by rotational flow alone, and a flowmeter needs to be incorporated in the arterial outflow to quantitate the actual pump output. If the pump outflow should become occluded, the pump will not generate excessive pressure and will not rupture the arterial line. Similarly, the pump will not generate significant negative pressure if the inflow becomes occluded. This protects against cavitation and microembolus formation. The newer generation magnetically levitated centrifugal pumps have been also used recently in the ECLS circuit and may have less traumatic effect on the blood elements.56
Heat exchanger. This allows for control of blood temperature as it passes through the extracorporeal circuit. Generally, the transfer of energy occurs by circulating nonsterile water in a countercurrent fashion against the circulating blood. Use of water as the heat-exchange medium provides an even temperature across the surface of the heat exchanger without localized hot spots. The use of a heat exchanger allows for maintenance of normothermia given the potential heal loss that can occur through the long circuit.
Circuitry interfaced between the patient and the system. The need for systemic anticoagulation on ECLS and the complications associated with massive coagulopathy and persistent bleeding during the postcardiotomy period led to the development of biocompatible heparin-bonded bypass circuits. In 1991, the Carmeda Corporation in Stockholm, Sweden, released a heparin-coating process that could be used to produce an antithrombotic surface.57 This process was applied to extracorporeal tubing and the hollow-fiber microporous oxygenator surface.58 Initial experience suggested that the need for systemic anticoagulation had been eliminated. In addition, heparin coating has been associated with a decrease in the inflammatory response with reduced granulocyte59 and complement activation.60 Bindslev and colleagues61 and Mottaghy and colleagues62 reported excellent hemodynamic support with minimal postoperative blood loss in experimental animals for up to 5 days. Magovern and Aranki reported similar excellent results with clinical application.63,64
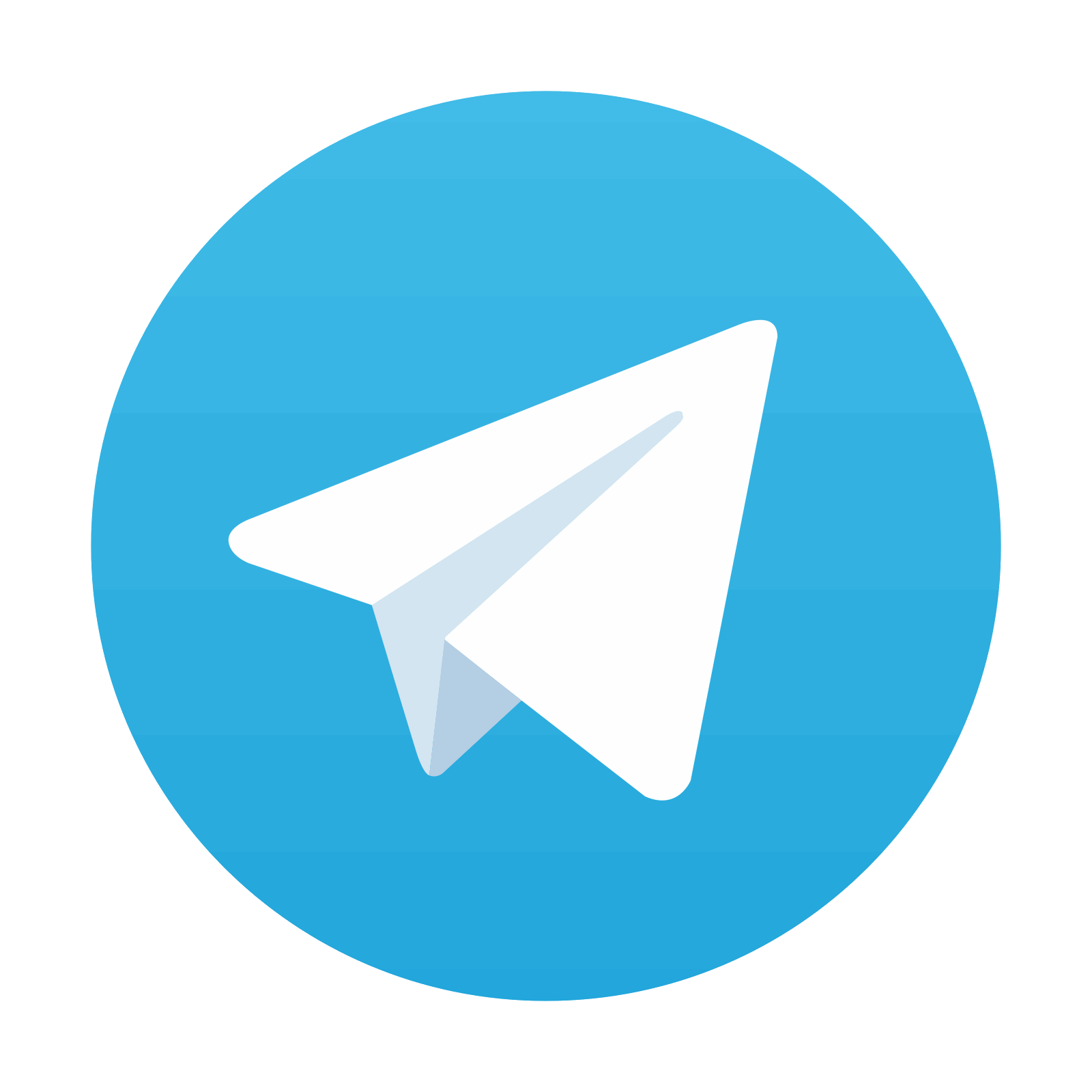
Stay updated, free articles. Join our Telegram channel

Full access? Get Clinical Tree
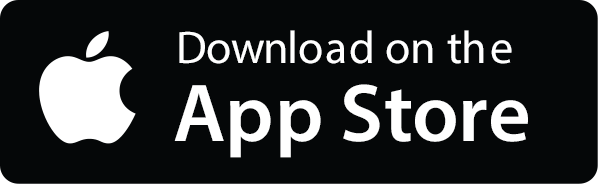
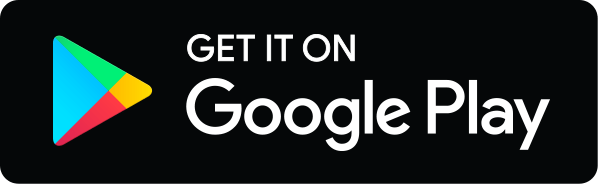