Fig. 34.1
An intact blood vessel and the partitioning of coagulation. Within the blood vessel, larger molecular weight coagulation factors (VIII and von Willebrand Factor) are confined to the intravascular space and cannot leave the blood vessel unless damage occurs. The same is true with platelets. Additional smaller molecular weight coagulation factors (II, VII, IX, X, XI, and XII) can freely diffuse in and out of the blood vessel and can interact with tissue factor-coated cells. This creates a primed procoagulant environment around the blood vessel. By partitioning the coagulation factors and platelets, minimal coagulation will occur inside the blood vessel under healthy conditions. TF tissue factor, PLT platelet, Large Coag larger molecular weight coagulation proteins, Small Coag smaller molecular weight coagulation factor, RBC red blood cell

Fig. 34.2
Blood vessel injury and cell-mediated hemostasis. Large coagulation factors and platelets have leaked into the extravascular space. The once separated large coagulation factors can now interact with tissue factor-bearing cells and small coagulation factors to generate a small amount of thrombin. Thrombin is the active enzyme in coagulation that cleaves fibrinogen into fibrin which forms a clot. This represents clot initiation (1). Platelets begin to aggregate around the injured vessel and in the presence of thrombin and other activating factors release additional coagulation factors (e.g., V) that increase thrombin generation. This phase of coagulation is known as amplification (2). During amplification a larger amount of thrombin is generated, and platelets take on an activated form from receptors on their surface that are activated by thrombin and extracellular matrix proteins. These activated platelets form a scaffold of phospholipid membrane that enables a large thrombin generation known as the “thrombin burst” and causing the third phase of coagulation, propagation (3). This cell- and protein-based interactions emphasize the importance of assessing coagulation from a whole-blood standpoint. Assays that partition coagulation require careful interpretation as they neglect to account for these interactions. This represents the first half of coagulation known as thrombosis. The second phase of coagulation is fibrinolysis in which the clot breaks down, which is a natural physiologic process to prevent clot occluding the blood vessel. TF tissue factor, PLT platelet, Activated PLT platelet that has been activated by thrombin and extracellular matrix proteins, Large Coag large molecular weight coagulation factor, Small Coag smaller molecular weight coagulation factor, RBC red blood cell
Amplification of coagulation is dependent on platelets. There are numerous additional enzymatic reactions that regulate this process, but ultimately when platelets bind to an area of injury, coagulation begins to rapidly proceed. This process is thought to be mediated by the dual activation platelets from both collagen (binding site of injury) and thrombin (local initiation of coagulation). This leads to platelet aggregation and assembly of coagulation factors. Platelets orchestrate the thrombin burst resulting in clot propagation by localizing coagulation factors on their surface. This results in high levels of thrombin cleaving fibrinogen and strengthening the forming hemostatic plug, which is further strengthened by fibrin cross-linking from factor XIII. These critical interactions between platelets and coagulation factors are key to appreciate when interpreting the results of conventional coagulation assays, as both an INR and PTT have excluded the critical platelet component.
What Is a Viscoelastic Assay ?
During coagulation, blood transitions from a liquid to a solid state (thrombosis) and will naturally degrade back to a liquid (fibrinolysis). These changes can be assessed using viscoelasticity. Viscoelasticity is a composite measurement of viscosity and elasticity quantified by a force causing a deformity. A simplified analogy is mixing cement with a stick. As the cement hardens, the force required to push the stick increases. This increase in force represents the transition of the cement from a liquid to a solid. Therefore, the force required to move the stick in the hardening cement is a crude quantification of the viscoelastic strength of the cement over time. This is an overly simplified explanation of how these machines which, which involves physics beyond what a clinician needs to interpret a viscoelastic assay.
The most common methodology to cause deformity of blood (the stick in the cement) is with a pin and cup. Thrombelastography (TEG) uses a rotating cup to deform blood, while ROTEM uses a rotating pin in a stationary cup. Additional methodologies can be used, but are beyond the scope of this chapter. As blood transitions from a liquid to solid state, the shear strain created by polymerizing fibrin and platelet aggregation (increasing viscosity) is transmitted to the device. Resistance is then quantified by the machine, which is visualized on a display as a single line splitting into two (Fig. 34.3). The distance between the two lines is proportional to viscoelastic resistance transmitted from the clot. This resistance is measured over time until the clot forms its maximum strength, represented by the two lines reaching their maximum amplitude and distance apart. This concludes the clot formation aspect of the device. After this point, the clot begins to lose strength through fibrinolysis, via cleavage of fibrin. The two lines’ amplitudes decrease, and are quantified over time. The end result of a viscoelastic assay is an hourglass-shaped tracing, which has numerous properties that can be calculated to approximate abnormalities of the different components of coagulation.


Fig. 34.3
Phases of the viscoelastic assay. This figure represents the viscoelastic readout as blood transitions from a liquid to a solid (thrombosis) and back to a liquid state (fibrinolysis). In viscoelastic assays the Y axis represents time and X axis represents resistance (relative clot strength). In the figure, the liquid form of blood is associated with a single line. As coagulation initiates the line splits. The larger the split between the lines, the higher the viscoelastic resistance is generated from the clot. These lines will continue to split until the clot forms its maximum strength. This represents thrombosis. The clot will then begin to degrade overtime in a process known as fibrinolysis. As the strength of the clot begins to decrease, the lines come closer together. Once the clot has returned back to the liquid state, the lines have now intersected and represent a single line
What Type of Blood Tube Is Optimal for Running a Viscoelastic Assay?
Blood samples run on viscoelastic assays can be stored in citrate (light blue top), heparin (green top), and no anticoagulant (white top) vacuum-sealed containers. Manufacturers have specific recommendations on the timing between blood sample draw and time to run assay. Heparin-based TEGs are primarily used in cardiovascular surgery and will not be further discussed in this chapter, although may be useful when treating patients with vascular injuries who require systemic heparinization. In the acute setting of trauma, a citrated sample or non-anticoagulant tube can be used. Despite concerns that running a citrated blood sample early after blood draw can alter results, this methodology is favored to guarantee that the patients’ assays can be completed. Non-anticoagulant blood sample is problematic, particularly during emergency department evaluation, as a significant amount of samples is coagulated before they could be assayed. While in a healthy individual a non-anticoagulated sample can sit for over 5 min before coagulation begins, the majority of trauma patients will have a primed coagulation system and initiate clot formation much sooner. This is particularly true if the blood sample is obtained as the first tube of collected blood, as it can contain TF-bearing cells from the puncture of the surrounding venous tissue. In the research setting, the first ml of blood after venous puncture is always discarded to reduce the risk of TF contamination.
The location of the blood draw is also relevant when interpreting TEG results. Arterial blood samples tend to be more hypercoagulable than venous samples. As early arterial blood draws may be challenging in hypotensive trauma patients, it is the authors’ recommendations to rely on venous blood draws when possible. In the more stable patients, while a fresh venous stick would be ideal, existing arterial and central venous catheters can be used for blood sampling. When trending the effects of blood products during resuscitation, it is optimal to not alternate between blood sampling sites. In the end, the differences between sampling sites may be more of an academic argument, as patients who are clinically hypocoagulable will likely demonstrate abnormalities regardless of the blood draw source.
Activation of TEG/ROTEM -Based Assay
Whole-blood generation of clot can be initiated through various mechanisms. The two classic pathways of coagulation are the intrinsic and extrinsic activators. Hemostasis in reality is driven by both of these pathways that do not act in isolation of each other. However, activation of blood with different agonists can produce different results with viscoelastic assays , which needs to be taken into clinical consideration. Extrinsic pathway activation is typically via tissue factor, while intrinsic, also known as contact, pathway, is activated through kaolin. ROTEM has simplified the naming of their assays as EXTEM for tissue factor-based activation and INTEM for kaolin-based activation. TEG uses both tissue factor and kaolin in the rapid TEG (rTEG) to expedite clotting and uses a kaolin TEG (kTEG ) for intrinsic activation.
The decision for running the specific assay is dependent on the clinical scenario. For example, the time to obtain clotting indices in an rTEG to guide resuscitation is 10 min quicker than kTEG due to rapid activation of the clotting. Therefore, an rTEG is optimal for use in hemodynamically unstable patients in both the emergency department and operating room. Unlike an INR and PTT, viscoelastic assays detect hypercoagulability. Detection of hypercoagulability in the intensive care unit is better assessed with a kaolin-activated assay. kTEG also demonstrates that the majority of trauma patients have elements of hypercoagulability on presentation to the hospital, which is not appreciated with rTEG. This could be attributable to saturating the activation of the coagulation system with supraphysiologic levels of tissue factors. The authors routinely use rTEG to guide blood product transfusions and kTEG to guide antiplatelet therapy in the intensive care unit for post-injury deep venous thrombosis prophylaxis. An important caveat of differences between ROTEM and TEG parameters is that despite assays targeted to specific coagulation pathways, the composition of the reagents in these assays is not the same, and thus indices are not interchangeable.
Viscoelastic Indices’ Correlation to Coagulation Abnormality
Viscoelastic indices are correlated to specific abnormalities of the coagulation system. However, assumptions are made that these processes are independent of each other to guide resuscitation, but the reality is that these variables are representative of interdependent processes occurring at the same time. In both TEG and ROTEM , regression analysis of specific indices correlates with fibrinogen (clotting factor) versus platelet contribution to clot formation. Interestingly, platelets are believed to contribute 80% of the ultimate clot strength. Despite the existing limitation of these assays, reproducible results can be determined in both TEG and ROTEM to predict patients at risk of massive transfusion and guide resuscitation of specific blood products. Abnormalities of the manufactured reported normal limits of TEG (Table 34.1) and ROTEM (Fig. 34.4) can be used as relative indications for blood product transfusion. However, the optimal threshold for transfusions based on readout of these assays in trauma is yet to be defined. The following sections describe the anatomy of these viscoelastic assays (Fig. 34.5) and association with blood product use.
Table 34.1
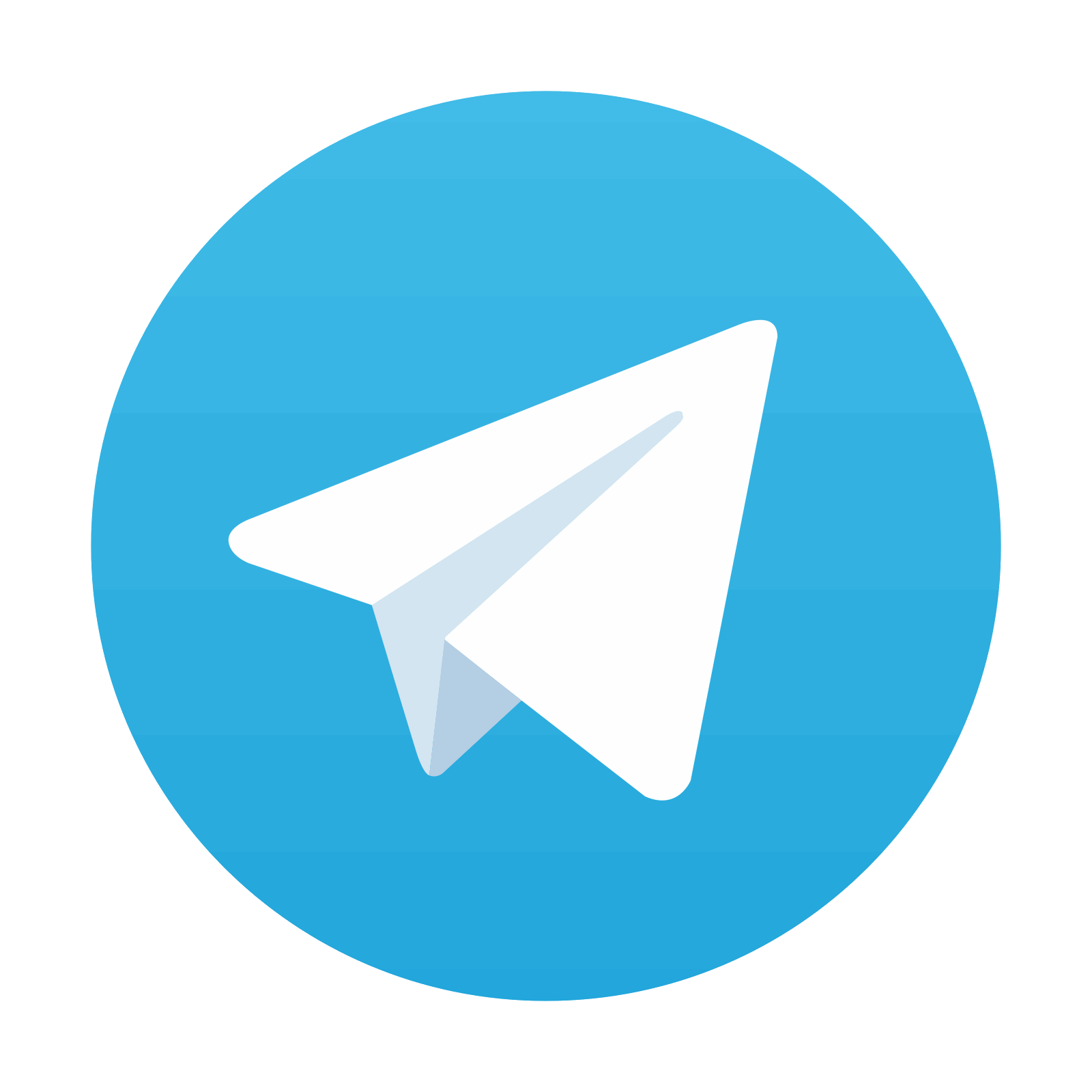
Manufacturer recommended normal limits of TEG 5000 device
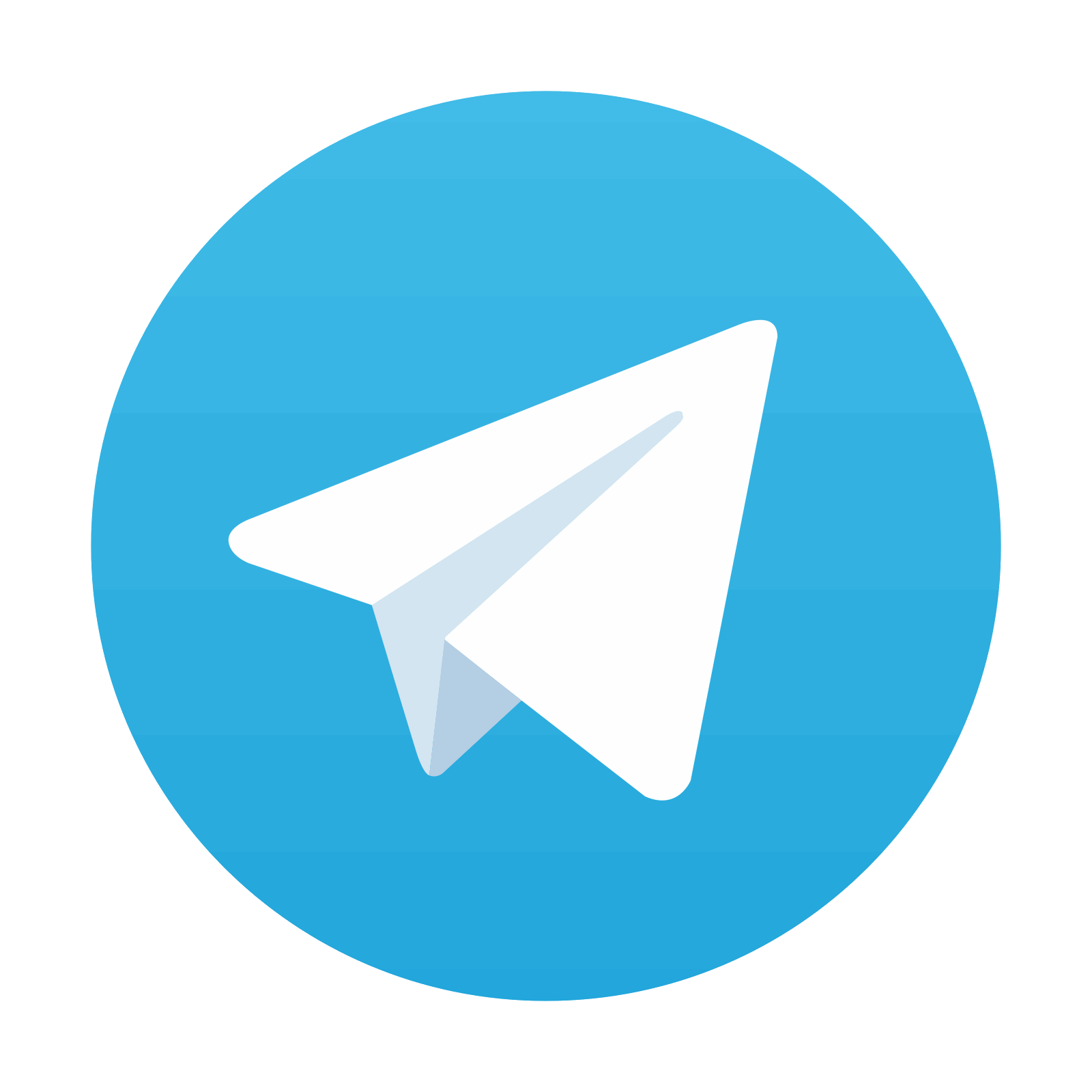
Stay updated, free articles. Join our Telegram channel

Full access? Get Clinical Tree
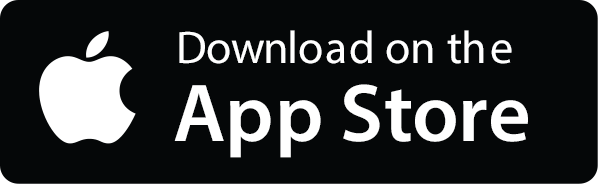
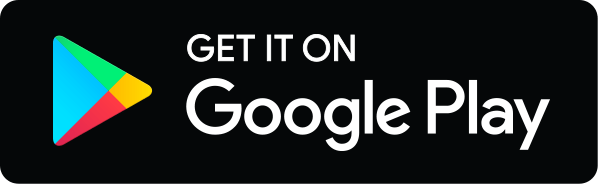
