Fig. 3.1
Pre-TAVR work-up. Preprocedural work-up in patients with severe aortic stenosis who are candidates for a TAVI procedure. CT computed tomography, LIMA left internal mammary artery, TAVR transcatheter aortic valve replacement

Fig. 3.2
MSCT: femoral accesses. Iliofemoral axes measurement with MSCT scan (Osiris software); Iliofemoral axes 3D reconstruction
The size of the aortic annulus is usually measured by transesophageal echocardiography (TEE), aortic root angiography (during coronary angiography), MDCT, or a combination of these imaging techniques. Accurate measurement of the aortic annulus is crucial to determine the appropriate transcatheter valve size, and although sizing the aortic annulus with 2DTEE has been associated with good clinical results in most cases, many studies have suggested a tendency toward an underestimation of the aortic annulus by echocardiography as compared with MDCT [26–31]. MDCT studies have clearly shown the oval shape of the aortic annulus in most patients, and nowadays this is the gold standard method for accurate aortic annulus measurement, to assess the most appropriate degree of valve oversizing, as extreme oversizing may increase the risk of aortic annulus rupture and coronary occlusion, while undersizing increases the risk for paravalvular leak and valve embolization (Fig. 3.3). Only in case of severe renal failure TEE might be an alternative to avoid the use of contrast media.


Fig. 3.3
MSCT: annulus measurement. Annulus measurement with MSCT scan (Osiris software)
Coronary angiography is performed before the procedure to evaluate the presence and severity of coronary artery disease (CAD). Many concerns arise when undergoing TAVR in the presence of significant yet untreated coronary atherosclerosis, such as possible coronary access limitations because of the presence of the prosthesis, and worsening of myocardial ischemia during implant. But on the other hand, percutaneous coronary intervention (PCI) in a patient with severe aortic stenosis may be problematic, and PCI prior to TAVR may increase complications because of the necessity of dual antiplatelet therapy and could potentially increase the risk of contrast-related kidney injury in such elderly patients. In cases of severe stenoses in the main coronary vessels, complete or partial coronary revascularization is often performed before TAVR. Data about both safety and feasibility of this strategy are limited but encouraging [32, 33].
Apart from this specific TAVI work-up to determine the feasibility and planning of the procedure, a complete risk stratification including assessment of concomitant diseases, comorbid conditions, and frailty is needed to adequately assess and determine the risk of the procedure.
3.2 General Anesthesia vs. Sedation
TAVR implantation can be done in general anesthesia or in local anesthesia and conscious sedation. In any case, the presence of the anesthesiologist during the procedure is strongly recommended. Subsequently in the progressive ease of the procedure over the years, many centers opted for an anesthesiologist “on call.” General anesthesia is mandatory for transapical, transaortic, and transcarotid access and is preferable for trans-subclavian and surgical femoral access and when transesophageal echo is used. For the percutaneous femoral procedure that represents the large majority of interventions, a local anesthesia with Xylocaine and Chirocaine, and if necessary a mild conscious sedation, is the best option. This approach is well tolerated by the patient and allows a lower complication rates, a quicker mobilization, and early discharge [33, 34]. This option is moreover mandatory in patients with severe obstructive respiratory disease where the intubation may lead to difficult weaning from the ventilator. In general, all the team has to be ready in case of severe and life-threatening complications to immediately convert into general anesthesia.
3.3 Percutaneous Femoral Access: Technique of Puncture, Prevention and Treatment of Complications of the Sheath Positioning
The transfemoral route (Fig. 3.4) is the first choice in the vast majority of centers performing TAVR procedures. Most centers are now using a fully percutaneous technique using vascular closure devices instead of the surgical cutdown [35]. Bleeding complications are strongly related to mortality. In the early phase of TAVR experience, the complication rate related to the femoral vascular access, as reported in all the registries and randomized trials, was dramatically high and was the major cause of immediate [13, 20] and late mortality [36]. The progressive reduction of the devices’ size; the accurate planning by means of CT scan to detect size, calcifications, tortuosity, and atherosclerotic disease; the correct puncture of the artery; and the contralateral protection led to a dramatic reduction of vascular complications [37–40]. The technique of femoral puncture for TAVR significantly differs from the conventional femoral artery puncture. The first step consists of the puncture of the secondary access leading to the crossover of the abdominal aorta with a catheter (pigtail, JR, LIMa catheter) and to the selective injection of few mls of contrast to visualize the correct puncture at the main access (Fig. 3.5). The latter should ideally be far above the bifurcation of the common femoral artery and below the inguinal ligamentum. After the injection, a 0.018-in. extra-stiff wire is positioned distal segment of the superficial femoral artery (Fig. 3.6). If crossover is impossible, the wire protection may be done from the radial artery. The puncture is then performed under fluoroscopy with a quite vertical angle (Fig. 3.7). For the transfemoral access, vascular pre-closure can be done with one ProStar or two ProGlide systems (Abbott Laboratories, IL, USA) (Fig. 3.8). After the insertion of an 0.35 extra-stiff wire, an 18-Fr sheath is advanced up to the abdominal aorta. In case of severely tortuous femoral artery, the use of even stiffer wires as Lunderquist or Backup Meier wires is useful or sometimes necessary. The new Edwards Sapien 3 and Medtronic Evolut R require smaller-caliber introducers. All maneuvers with the introducer sheath should be done gently and under fluoroscopic guidance. In case of excessive resistance to the sheath advancement, peripheral balloon dilation may be considered. At the end of the procedure, sheath retrieval is one of crucial points for complete TAVR success. Best practice suggests to gently remove the introducer with pressure monitoring at the tip just above the puncture site. If sheath retrieval is difficult, we suggest to reinsert the mandrel to avoid the “sucking effect” possibly leading to the avulsion of the iliac artery. Then using a crossover balloon over the 0.018 crossover wire to completely occlude the common femoral artery and reduce bleeding during the suture is safe (Fig. 3.9). After that a selective angiography has to be performed to assess the final result (Fig. 3.10). In case of visualization of bleeding, the balloon has to be inflated at the puncture site for some minutes to achieve the vessel closure and avoid major bleedings [38]. In case of failure, the sealing may be easily obtained using a covered stent from the contralateral access through the crossover wire (Fig. 3.11). Different 18-French femoral introducers are now available on the market. The Cook sheath was suggested from the beginning for CoreValve implantation for its stiffness allowing the device retrieval (Fig. 3.12). Medtronic Sentrant, Saint Jude, Jo-tech, Boston Scientific, and GORE are good alternatives. There are two other introducers, such as the “eSheath” and the “Solopath,” which share a common feature such as the modifiable profile, i.e., they implement a dynamic expansion mechanism aiming at reducing the vascular injury (Fig. 3.13).











Fig. 3.4
Multiple accesses. Approaches used for transcatheter aortic valve implantation. A the transfemoral approach. B the transapical approach. C the transaortic approach. D the subclavian approach. E the transaxillary approach (usually the left vessel)

Fig. 3.5
Crossover angiography. The aortic crossover is performed with a 5-Fr catheter; after that a selective injection is performed in the iliofemoral axes with few mls of contrast to visualize the correct puncture at the main access

Fig. 3.6
Crossover wire. Using the 5-Fr. crossover catheter, a 0.018-in. extra-stiff wire is positioned in the distal segment of the superficial femoral artery

Fig. 3.7
Main vessel: femoral puncture. The puncture is performed under fluoroscopy with a quite vertical angle of the needle

Fig. 3.8
Femoral pre-closure: ProStar and ProGlide. For the transfemoral access, vascular pre-closure can be done with two ProGlide (a) or one ProStar system (b)

Fig. 3.9
Crossover balloon inflation. It’s strongly recommended to use a crossover balloon over the 0.018 crossover wire to completely occlude the common femoral artery and reduce bleeding during the suture (a); the pressure drop recorded on the tip of the balloon assesses the vessel occlusion (b)

Fig. 3.10
Final selective angiography. “Y” connector with a 10 cc Luer-Lok syringe allows manually dye injection, keeping the protection wire in place (a); a selective angiography has to be performed to assess the final result (b)

Fig. 3.11
Covered stent. In case of vessel closure failure with bleeding, (a) the sealing may be easily obtained using a covered stent from the contralateral access through the crossover wire. A selective angiography has to be performed to assess the final result and the artery sealing (b)

Fig. 3.12
Cook sheath. The Cook sheath was suggested from the beginning for CoreValve implantation for its stiffness allowing the device retrieval

Fig. 3.13
eSheath and Solopath. The “eSheath” (a) and the “Solopath” (b) share a common feature. With a modifiable profile, they implement a Dynamic Expansion Mechanism aiming to reduce the vascular injury
3.3.1 Alternative accesses: Subclavian, Transaortic, Transapical, Transcarotid
3.3.1.1 Transapical Approach
Lichtenstein and colleagues [4] in 2006 implanted a Cribier-Edwards via the transapical approach for the first time. Potential advantages of the transapical approach include the avoidance of large catheters through the iliofemoral system, aortic arch, ascending aorta, and aortic valve and an improved coaxiality of the delivery system with the aortic annulus. The main disadvantages are the need for general anesthesia; a thoracotomy; a greater degree of myocardial injury, owing to the apical perforation of the left ventricle [41]; and the potential bleeding complications associated with the surgical repair of the apex. Transapical approach is associated in literature with worse outcomes compared to transfemoral approach [20, 25, 42].
3.3.1.2 Transaortic Approach
In 2009 and 2010, the use of the transaortic approach through a hemisternotomy (mini-J sternotomy) or a right thoracotomy was proposed as an alternative approach with the MCV and ESV systems [43]. Possible advantages are avoidance of large catheters through the iliofemoral system and aortic arch and avoidance of puncture of the ventricular apex. Possible disadvantages are the surgical local complications including those to the chest wall and pleurae.
3.3.1.3 Subclavian Approach
The subclavian approach has emerged as an alternative to the transfemoral approach with the MCV system [44, 45]. A surgical cutdown is needed to isolate the subclavian artery (both left and right). Possible advantages rely on the very short distance between the vascular access and the aortic valve leading to a better control of the MCV prosthesis during positioning and deployment. However, any injury of the subclavian artery will translate into a major intrathoracic bleeding that might be difficult to control. Minor injury of the subclavian artery such as dissection or stenosis due to the surgical sutures is easily managed percutaneously. Recently, a direct percutaneous approach has been developed by Schafer and his colleagues using a vascular closure device or a graft stent when this fails [46].
3.3.1.4 Transaxillary and Transcarotid Approach
De Robertis and colleagues described the first-in-human MCV implantation by the surgically isolated transaxillary artery [47]. The potential advantage of this approach versus the subclavian approach is that any injury to the axillary artery can be easily repaired with no major clinical consequences. Indeed, and unlike in iliofemoral vessels, occlusion of the axillary artery would be compensated by the collateral circulation between the thyrocervical trunk of the subclavian artery and the subscapular artery. Some case reports and small series described successful implant via the common carotid artery [48].
3.4 How to Find Good Projection: Crossing Aortic Valve
3.4.1 Aortography
After introduction of the appropriate sheath, a pigtail catheter is placed in the bottom of the noncoronary cusp, and aortic root injection is performed using 10–15 mL of contrast at a rate of 15–20 mL/s. This aortogram should be obtained in 15° left anterior oblique (LAO) projection to align the three aortic cusps (Fig. 3.14). Usually, this will visualize the valve opening to guide the crossing of the aortic valve. If the valve opening is not well visualized, a 15° right anterior oblique (RAO) projection may be useful.


Fig. 3.14
Angio with three cusps’ visualization. A pigtail catheter is placed in the bottom of the noncoronary cusp, and aortic root injection is performed using 10–15 mL of contrast at a rate of 15–20 mL/s. This aortogram should be obtained in, e.g., a 15° left anterior oblique (LAO) projection aligning the three aortic cusps
In case of impaired renal function, cinematography without contrast will often visualize leaflet calcification and valve orifice. The baseline aortogram should be stored and used as a reference point to avoid multiple contrast injections during valve implantation.
3.4.2 Crossing the Aortic Valve
The way of crossing the aortic valve is according to operator’s discretion. This may be done using an AL1 or AL2 catheter and a straight standard or soft guidewire.
When the aortic valve is crossed, the AL catheter may be replaced over a standard J-tip exchange guidewire to a pigtail catheter. This allows better hemodynamic assessment and also reduces the risk of left ventricle perforation when the stiff guidewire is introduced.
3.4.3 Stiff Wire in the Left Ventricle
An either manually shaped or dedicated pre-shaped super stiff wire with a minimum length of 260 can be used. If manually shaped, the curved part should include both the soft and stiff distal part of the wire. The size of the wire curvature may be based on the left ventricular dimensions.
The stiff guidewire is introduced through the pigtail catheter in, e.g., RAO 15° projection to ensure placement at the left ventricular apex. The pigtail shape of the guidewire is used to prevent left ventricular perforation when advancing the valve system and if pushing on the guidewire is used during valve deployment. An example of a non-pre-shaped guidewire is the Amplatz Super Stiff wire (Boston Scientific, MN, US). Dedicated pre-shaped guidewires include Confida (Medtronic, MN, US) and the even stiffer Safari (Boston Scientific, MN, US) guidewire (Fig. 3.15).


Fig. 3.15
Safari wire and Safari in the ventricle. Dedicated pre-shaped guidewires: technical features of Safari (Boston Scientific, MN, US) wire (a); Safari wire placed in the left ventricle (b)
3.5 Predilation
3.5.1 Balloon Aortic Valvuloplasty
Balloon aortic valvuloplasty (BAV) with rapid ventricular pacing (e.g., 160–180 bpm) is suggested before valve implantation. The size of the balloon should be slightly smaller than the annulus diameter to minimize the risk of annulus rupture. Both straight and dog bone-shaped and compliant and noncompliant balloons can be used.
In case of severe calcification of the aortic valve, an even smaller balloon may be used.
There are many cases where direct valve implantation may easily be done avoiding predilation, especially with self-expandable valves as CoreValve, Portico, or Boston Lotus, but is feasible even with Sapien 3 balloon-expandable valve [49, 50]. In our experience, we perform direct implant in case of minimal valve calcification, large size annulus, and presence of aortic regurgitation, low flow-low gradient aortic stenosis to avoid rapid pacing in reduced left ventricular function, and in valve-in-valve procedures to reduce the risk of debris embolization.
Simultaneous contrast injection with a pigtail during BAV may be helpful in ensuring the correct size of the valve prosthesis in case of doubt (Fig. 3.16). Appropriate annulus sealing by the use of balloon diameter may help in estimating the required device diameter to achieve optimal sealing. This technique may also reveal the motion of the native valve leaflets toward the sinuses of Valsalva and potential coronary occlusion by calcified nodules.


Fig. 3.16
Valvuloplasty and aortic root injection. Simultaneous contrast injection with a pigtail during BAV may be helpful in ensuring the correct size of the valve prosthesis in doubtful cases
The procedure described above is the same for all the transcatheter aortic valves implanted through the femoral route, but valve implantation significantly differs depending on the type of device used.
3.6 Technique of Valve Release (Balloon Expandable vs. Self-Expandable): Tips and Tricks
3.6.1 Medtronic CoreValve and Edwards Valves
Two transcatheter heart valve (THV) designs have been largely used in Europe for many years and are now both approved in the United States (Fig. 3.17) and described in Table 3.1: the Edwards Sapien THV (ESV; Edwards Lifesciences, Irvine, CA, USA), in bovine pericardium which utilizes a balloon-expandable tubular frame, implanted at the intra-annular position and the Medtronic CoreValve prosthesis (MCV; Medtronic Inc, Minneapolis, MN, USA), in porcine pericardium which utilizes a self-expanding multistage frame. A unique feature of the MCV is that this device is anchored not only within the aortic annulus but also extends superiorly to anchor in the supracoronary aorta and works in supra-annular position. Early versions of both the ESV (prototypic Cribier-Edwards and the more widely used Sapien THV) and MCV devices required large 22–25-Fr delivery systems. The subsequent evolution of the devices (Sapien XT and last generation of CoreValve) led to the use of low-profile delivery systems, and until 2014 both were available with comparable 18-Fr delivery systems, allowing transfemoral application in the majority of patients with aortic stenosis. The MCV device is a long device allowing for a wide range of implant depths and is associated with less hemodynamic instability during deployment as it can be implanted without rapid ventricular pacing. It could also be implanted without balloon predilation entirely eliminating the need for rapid pacing [49]. It is potentially retrievable if positioned incorrectly, although this is technically demanding. The risk of acute coronary obstruction by a displaced native valve leaflet may be lower [50]. Both valves are supported by a larger body of publications. Two randomized trials one with ESV [19] and one with MCV [21] have shown the great benefit of TAVR in terms of mortality over medical management in non-operable patients. PARTNER A trial [20] in 2011 with ESV and CoreValve US trial [22] in 2014 demonstrated clearly that TAVR is the best option even for operable but at high surgical risk patients with comparable or superior immediate- and long-term results. The efficacy of TAVR compared to surgery in intermediate- and low-risk patients has to be demonstrated in ongoing randomized trials with both valves (PARTNER 2 and SURTAVI), but propensity matched registries [22–24] have already confirmed this hypothesis. Balloon-expandable valves and self-expandable valves have different pros and cons in different clinical and anatomic subsets, and it is difficult compare their results in randomized trial. The only published Comparison of Transcatheter Heart Valves in High-Risk Patients with Severe Aortic Stenosis: Medtronic CoreValve versus Edwards Sapien XT is the CHOICE trial that compared for the first time the two different THV technologies. The primary end point of the trial was device success and showed a lower frequency of residual more than mild aortic regurgitation and less need for implanting more than one valve for ESV but without difference in clinical outcomes at 30 days [52]. Comparisons with regard to durability are largely speculative; nevertheless, the available long-term follow-up of MCV and ESV patients documents no major structural valve deteriorations up to 3 and 5 years, respectively, after valve implantation [36, 53, 54].


Fig. 3.17
THV. Transcatheter heart valves currently used for transcatheter aortic valve implantation. (a, b) The Edwards Sapien XT (Edwards Lifesciences, Irvine, CA, USA) valve. (c, d) The third-generation of the CoreValve prosthesis (Medtronic Inc, Minneapolis, MN, USA)
Table 3.1
Comparison of the Edwards Sapien XT and the Medtronic CoreValve prosthesis
Frame | Edwards Sapien XT | Medtronic CoreValve |
---|---|---|
Cobalt chromium | Nitinol | |
Leaflets | Bovine pericardial | Porcine pericardial |
Expansion | Balloon expandable | Self-expandable |
Retrievable | No | Prior to release (difficult) |
Annular/valvular fixation | Yes | Yes |
Ascending aorta stabilization | No | Yes |
Manufacturer diameters and delivery system | 20 and 23 mm (16-Fr expandable sheath) | 26, 29, and 31 mm |
(18-Fr sheath and delivery system) | ||
26 mm (18-Fr, expandable sheath) | (23-mm CoreValve Evolut device) | |
Annulus diameter (mm) | 18–27 | 18–29 |
Minimum arterial diameter | 6 | 6 |
Suitability | AS | AS |
Combined AS and AR | Combined AS and AR | |
Pulmonary position | Valve in valve (aortic position only) | |
Valve in valve (all four positions) | ||
Transapical access | Yes | No |
Pacemaker incidence | 4–8 % | 15–40 % |
Randomized trials | PARTNER trial (completed) | CoreValve US trial (completed) |
AR: aortic regurgitation; AS: aortic stenosis |
3.6.2 CoreValve Implantation
The technique of CoreValve implantation is similar to other self-expandable valves.
The delivery system is advanced over the stiff guidewire in the left ventricle until the distal part of the valve frame has crossed the aortic valve. There are two options for the C-arm projection during valve deployment, having either the three aortic cusps aligned or the delivery system aligned. For the first option, this projection can be determined from computed tomography (CT) scanning used for work-up or by aortic root injections until the correct projection is found (Fig. 3.14). Often a LAO/cranial projection is used. For the second option, the projection is determined reducing the “parallax effect” by “closing” the distal marker band of the protective sheath (Fig. 3.18). The marker band should appear as a line, often requiring a LAO/caudal projection. Usually, the C-arm orientation will remain unchanged during the entire deployment but may also be adjusted several times in order to have the inflow part of the valve frame in-plane. Crossing the aortic valve is usually easy due to the low profile of the protective sheath and the atraumatic tip. However, in case of difficult crossing, combination of pushing the system and pulling the guidewire can facilitate advancement to the intended position. Alternatively, a stiffer guidewire can be used (i.e., Lunderquist, Cook Medical, IN, USA). All maneuvers should be done gently, especially when a very stiff guidewire is used, in order to avoid left ventricular perforation. Deployment of the valve begins with clockwise rotation of the deployment wheel on the handle. Usually two operators are involved when deploying the valve: one operator controls the depth of implantation by pulling or pushing on the delivery system, while the second operator rotates the wheel. Any adjustment of the guidewire will affect the control of the deployment and thus the depth and angulation of implantation. The ideal depth of implantation is represented by the frame’s inflow edge placed 2–6 mm below the aortic annulus (range 0–12 mm). Low implants are associated with higher rate of PVL and AV block [64]. The pigtail in the noncoronary cusp serves as a landmark, and small aortic root injections (e.g., 10 ml at 20 ml/s) may be used to evaluate the implantation depth (Fig. 3.19). When the protective sheath is withdrawn and the valve prosthesis is exposed, the system tends to dive into the left ventricular outflow tract (LVOT). This movement can be minimized by slow rotation of the wheel and by adjusting the position of the valve frame by pulling the delivery system or pushing on the guidewire. Furthermore, some tension should be applied to the delivery system should in order to prevent such diving motion. Further rotation of the wheel allows more exposure and flaring of the frame that is slowly apposed on the left coronary cusp wall, fixing the distal part of the device to the virtual basal ring (Fig. 3.19). At this point, due to the supra-annular position, the Corevalve bioprosthesis might be still closed thus determining a drop of systemic pressure. A quick rotation of the wheel until two-thirds of the frame allows the increase of the pressure. At that point, it is possible to control the position before the release, and small adjustments may be done pulling the catheter. For the valve release, any tension or compression should be released by ensuring that the delivery system is central within the ascending aorta and that there is no remaining tension or compression on the guidewire. Fluoroscopic visualization of retention tabs being detached from the delivery system confirms complete valve release. Withdrawal of the delivery system is attempted only when valve release is confirmed. The stiff guidewire is slightly pulled back in order to lift the nose cone up as it crosses the inflow part of the valve. Once pulled back in the descending aorta, the delivery system is closed pushing forward the sledge of the protective sheath.



Fig. 3.18
Prosthesis alignment in the native valve. The prosthesis is advanced over the stiff guidewire until the distal part of the valve frame has crossed the aortic valve. Optimal alignment is crucial

Fig. 3.19
CoreValve implantation. (a) Baseline aortic angiography. (b) Initial stage of prosthesis deployment. (c) Prosthesis almost released. (d) Prosthesis fully deployed with trivial paravalvular leak
A deep implantation may be associated with moderate to severe paravalvular leakage because the sealing cuff is located below the annulus. Whereas indicated, a second valve should be implanted in a higher position (valve in valve). Snaring the implanted valve and pulling it to a higher position may cause vascular complications in the ascending aorta and is only recommended if the valve impedes on cardiac structures in the left ventricle or obstructs the coronary ostia.
3.6.3 Edwards Valve Implantation
The technique of implantation of ESV is unique and completely different from self-expandable valve implantation. It is probably more predictable, but being a “one-shot implant,” you have less possibility to correct wrong positions. Edwards XT and the new Sapien 3 valve is loaded over the balloon in a straight segment of descending aorta pulling back the catheter until the distal part of the valve matches exactly with the central marker band of the balloon. Then, in an angiographic projection perpendicular to the valve, counterclockwise rotate the fine alignment wheel for exactly centering the valve between the balloon’s markers. Once loaded the valve aortic arch is crossed clockwise rotating the flexion wheel to deflect the catheter avoiding to scrape the aortic arch. Then, the native aortic valve is slowly crossed avoiding abrupt movements. Before the release, it is possible to adjust the angiographic position and the centering of the valve by rotating the flexion wheel. Valve expansion is achieved by balloon inflation under rapid pacing (180–220 bpm) to minimize cardiac output and avoid valve embolization during valve implantation. Balloon inflation has to be maintained for at least 3 s. During the valve release, an angiography with few mls of contrast may be done to assess the position and the complete expansion. The rapid pacing has to be stopped after the complete deflection. Then the delivery system is retracting in the descending aorta and the result is evaluated (Fig. 3.20).


Fig. 3.20
Edwards XT implantation. (a) Baseline aortic angiography, (b) image showing the deployment of the valve, (c) the fully deployed Edwards Sapien transcatheter heart valve; at final aortic angiography, no paravalvular leak was observed
3.7 Assessment of the Result
The assessment of the result is the same for all the devices. Trivial or mild PVL is commonly observed after TAVI. More than mild PVL is reported in 15–40 % of cases, in early experience series, which is considerably higher than after SAVR [55–62]. Moderate to severe PVL can have important consequences on patient safety and outcome, leading to hemodynamic deterioration, left ventricular (LV) remodeling, and aortic valve reintervention, and is one of the major predictors of long-term mortality [13, 18, 56, 57]. Aortic root angiography and echocardiography, in addition to hemodynamic assessment, are currently used to estimate the degree of PVL during the procedure and indicate whether further interventions are necessary. Simultaneous aortic and left ventricular pressure measurements at the end of the procedure are important to calculate the aortic regurgitation (AR) index, which is the ratio of the transvalvular gradient between diastolic blood pressure in the aorta and left ventricular end-diastolic pressure to systolic blood pressure in the aorta: ([diastolic blood pressure – left ventricular end-diastolic pressure]/systolic blood pressure) ×100 [57]. The AR index showed an inverse proportion to the severity of PAR differentiating between patients suffering from mild, moderate, or severe PAR and independently predicting the associated 1-year mortality risk. The AR index is a helpful tool to identify cases in which corrective measures to decrease the severity of PAR must be applied; however, it still has to be validated in a larger and controlled study population. Semiquantitative evaluation by means of angiography and echocardiography is obviously very important to obtain a final multiparametric evaluation of the acute result. Angiography is limited in adjudicating the PVL as the classification proposed by Sellers [63] relates to the regurgitation of the native valve, not of a transcatheter bioprosthesis valves that is completely different because the regurgitant jet paints the ventricular wall and might be overestimated thus suffering of a interobserver variability. It depends also to the pigtail position and to the timing of angiography that usually is not standardized. Echocardiography maintains therefore a great importance to judge acute results in case of doubt and to assess the result at discharge and during follow-up. In all recent studies, it is considered the gold standard to judge residual aortic regurgitation. Significant PVL most commonly results from incomplete prosthesis apposition to the native annulus due to pattern/extent of calcification [64–68] or annular eccentricity [69, 70], undersizing of the device [71, 72], and/or malpositioning of the valve [73].
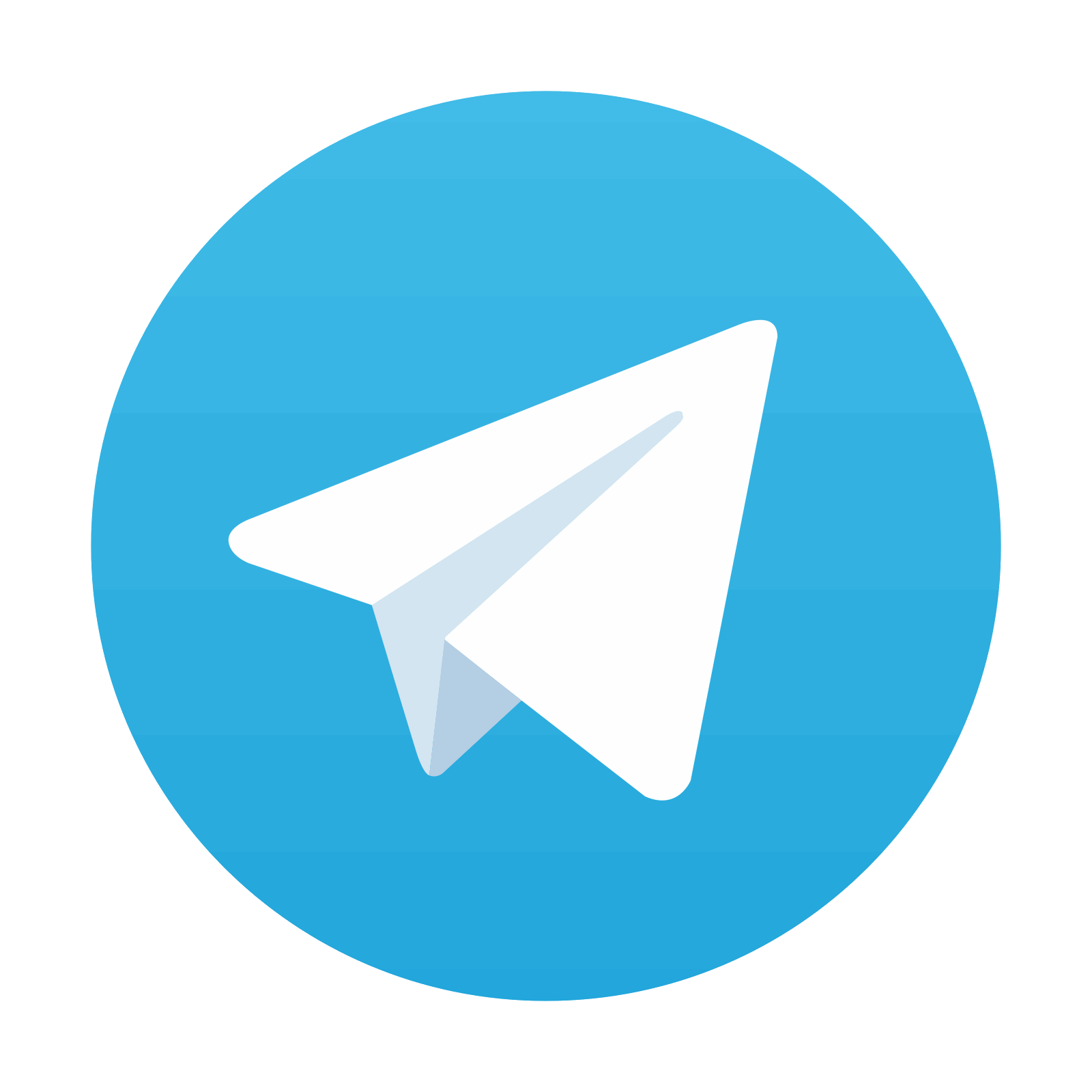
Stay updated, free articles. Join our Telegram channel

Full access? Get Clinical Tree
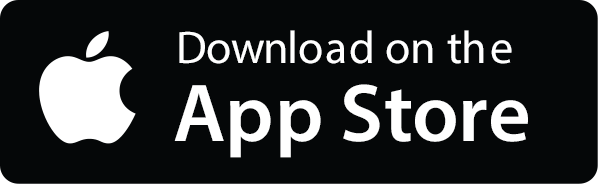
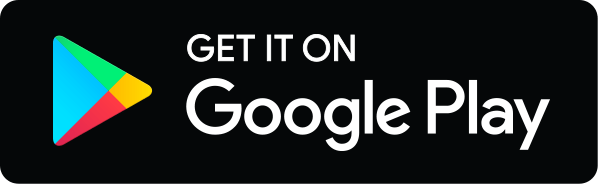