Introduction
Improvement in the acute treatment of myocardial infarction (MI) has made it possible for many patients to survive an initial MI and subsequently be exposed to the risk of recurrent MI and/or of heart failure (HF). The acute ischemic and reperfusion myocardial injury (see Chapter 24 ), as well as the acute hemodynamic effects of MI (see Chapter 25 ) and the potential complications of MI (see Chapter 26 ), are described elsewhere in this book (see also Chapter 13 ). The chronic consequences of MI leading to global structural and functional changes to the heart are summarized in a process known as adverse ventricular remodeling . The designation “adverse” refers both to the disadvantageous changes from a hemodynamic standpoint and to the negative prognostic implications of the process. This chapter reviews the definition of ventricular remodeling, its macroscopic and microscopic characteristics, and the therapeutic approaches to mitigation of adverse remodeling. The role of stem cell therapy in MI is, however, treated separately (see Chapter 22 ).
Definition of Adverse Ventricular Remodeling
Experimental animal models and longitudinal follow-up studies in patients with large non-reperfused MI have led to the identification of a dynamic process involving the heart muscle occurring after the MI, yet separate and largely independent from the initial ischemic changes. The definition of ventricular (or cardiac) remodeling refers to changes in size, shape, thickness, and elastance of the left and/or right ventricles involving both the infarcted and noninfarcted myocardial segments, and leading to impairment of regional or global systolic contractility and of diastolic function ( Table 36-1 ). The changes may progress for weeks and months after MI and lead to worsening left ventricular dilation and systolic dysfunction, HF symptoms, need for hospitalization, and premature death ( Figure 36-1 ). Quantitative evaluation of ventricular remodeling can therefore be used to predict clinical outcome and the therapeutic effects of a drug or device intervention.
Infarct Segment |
|
Border Zone Segment |
|
Non-Infarct Segments |
|
Global Dimensions |
|
Systolic Function |
|
Diastolic Function |
|
Dimensions and Function of the Atria |
|
Valvular Function |
|
Electrical Function |
|
Neuroautonomic Function |
|
Systemic Vascular Function |
|
Pulmonary Vascular Function |
|

Larger infarct size, greater extent of initial wall motion abnormalities, and greater initial reduction in global systolic function are all independent predictors of adverse ventricular remodeling ( Table 36-2 ). Ventricular dilation, indeed, begets further dilation. Accordingly, in the pre-reperfusion era, adverse ventricular remodeling—defined as a significant increase in left ventricular end-diastolic volume (relative increase greater than 25%) or a reduction in left ventricular ejection fraction (LVEF) (decreasing to below 45%)—was seen in more than one half of MI survivors. The degree of adverse remodeling was associated with mortality, and despite close follow-up, the mortality rates in MI survivors after hospital discharge exceeded 5% and 10% at 1 and 12 months, respectively. The advent of reperfusion strategies has changed the treatment and revolutionized the natural history of MI (see Chapter 2 and Chapter 13 ). In the current reperfusion era with primary percutaneous coronary intervention (PCI), not only has acute in-hospital MI mortality significantly decreased, but a majority of MI survivors have preserved left ventricular systolic function (at discharge and at 1-year follow-up evaluation), and correspondingly, the 1-year survival rate for MI survivors generally is excellent (greater than 97%). Despite the marked improvement in survival, the incidence of HF after MI is rising. This shifting incidence probably reflects multiple concurrent influences: (1) Higher-risk patients with MI are surviving the acute MI yet remain at risk for HF; (2) patients presenting with MI constitute an older age group than before and thus may have survived a previous MI, have more comorbid illnesses, and be at risk for preexisting HF; and (3) among today’s physicians, recognition of HF symptoms has improved. Of note, not all patients with HF after MI have systolic dysfunction. Indeed, the incidence and prevalence of HF with preserved LVEF—“diastolic HF” —are rising, reflecting that impairment in diastolic function can occur independently of changes in global systolic function, and it is common in the general population, as well as in the post-MI population. In a recent study in patients with ST-elevation myocardial infarction (STEMI) a restrictive diastolic filling pattern at echocardiography was associated with a five-fold increased risk of HF or cardiogenic shock, even in those patients with preserved left ventricular systolic function, whereas the association of restrictive diastolic pattern and depressed left ventricular systolic function (LVEF less than 45%) was associated with greater than an eight-fold increased risk.
Infarct Size |
|
Wall Motion Abnormalities |
|
Systolic Function |
|
Diastolic Function |
|
Cardiac Dimensions |
|
Valvular Function |
|
Clinical Conditions |
|
Biomarkers |
|
Cellular and Molecular Mechanisms
Although the original description of adverse remodeling refers to macroscopic changes, research over the past decades has identified a cellular and molecular remodeling process that precedes and causes the macroscopic changes of ventricular remodeling. The myocardium is composed of cardiomyocytes, endothelial cells, fibroblasts, and resident and infiltrating leukocytes (see also Chapter 4 ). Each of these cellular components plays a role in preventing or promoting adverse ventricular remodeling ( Figure 36-2 ).

Cardiomyocytes
Cardiomyocyte death is the hallmark of MI, and the release of sarcomere proteins (i.e., troponins) in the bloodstream is used to diagnose acute MI (see Chapter 6 and Chapter 7 ). The acute phase of cardiomyocyte death by oncosis or necrosis, referring to cell death with swelling and rupture, peaks within 24 to 48 hours of the onset of ischemia. Reperfusion provides rescue of the injured-but-salvageable cardiomyocytes but accelerates the demise of the nonsalvageable cardiomyocytes (see Chapter 24 ). Morphologic and molecular studies in acute MI have shown that multiple overlapping modalities of cell death contribute to the infarct core. Although the exact nature and nomenclature of cell death in MI is a subject of debate, apoptosis generally refers to a “silent”’ form of cell death, and all these forms of cell death are characterized and differentiated from oncosis, by the fact that they are mediated by active, energy-dependent, signaling processes. Necroptosis also is referred to programmed cell death but while it is an energy-dependent, coordinated process, it shares morphologic features of necrosis (i.e., cell rupture). Loss of cardiomyocytes continues for days to weeks after MI, occurring mainly in the myocardium bordering the infarct (border zone), and promoting progression toward adverse ventricular remodeling and HF.
The surviving cardiomyocytes in the border zone display structural and functional changes. These cells often are described as “degenerated,” “myofibrillarlytic,” “vacuolized,” or “autophagic” cardiomyocytes and exist in a delicate balance between death and survival. These changes lead to impaired contraction, with increased risk of infarct expansion and of arrhythmogenesis. Cardiomyocytes in the remote myocardium, by contrast, are subject to prohypertrophic stimuli such as increased wall stress (stretch) and locally or systemically released neurohormones—such as angiotensin II, aldosterone, and norepinephrine—or inflammatory mediators—such as interleukin-1β (IL-1β) and tumor necrosis factor-α (TNF-α). Increases in the cardiomyocyte cross-sectional area or volume (hypertrophy) and development of interstitial fibrosis without a parallel growth in the capillary bed lead to an imbalance between perfusion and demand as a consequence primarily of an impaired diffusion (increased distance between the capillary oxygen content and the cardiomyocyte mitochondria) and an impaired diastolic relaxation and increased intracavitary filling pressures, thereby further reducing the subendocardial perfusion gradient.
The imbalance in perfusion-versus-demand and the neurohormonal and proinflammatory milieu promote cardiomyocyte diastolic and systolic dysfunction and transition from prohypertrophy to proapoptosis signaling. This process results in further loss of viable myocardium, further stimulation of the compensatory mechanisms, in a vicious circle, leading to progressive ventricular remodeling and HF. Loss of contractile function and impaired relaxation in cardiomyocytes are, therefore, major contributors to adverse ventricular remodeling and HF after MI (see Chapter 25 ).
Endothelial Cells
The endothelium regulates the transit of oxygen and nutrients between the blood and the cardiomyocytes. Endothelial cells are exquisitely sensitive to ischemia. Death of endothelial cells precedes and promotes cardiomyocyte death in ischemia, through the release of proapoptotic factors. There is no restitutio ad integrum (restoration to original condition) after infarction in the heart—largely owing to the fact that once the vascular integrity is compromised, the microvasculature is not reestablished in a functional way. The capillary network in the infarct area resembles the granulation tissue in a wound with short, ectatic, and chaotically distributed capillaries. The endothelium also regulates adhesion and migration of the leukocytes during the repair phase. Proinflammatory changes in endothelial cells occur within minutes of onset of ischemia and persist for weeks. Loss of microvascular integrity is considered to be responsible for the lack of tissue level reperfusion despite the epicardial coronary revascularization that is occasionally seen in MI (“no reflow” phenomenon) and portends greater myocardium loss and adverse remodeling changes (see Chapter 24 ).
Fibroblasts
The heart contains a large number of fibroblasts. The interstitial collagen matrix in the myocardium is essential to maintain the myocardial structure compact during systole, when intense tension forces are applied. If the connective tissue was not present or if it had reduced tensile strength, the heart would “break” during systole by “leaking” at the points of least resistance. This matrix is carefully maintained by the resident fibroblasts. When the pressure in the heart increases, such as with systemic arterial hypertension or aortic valve stenosis, the collagen content increases in parallel. During acute MI, the infarct area undergoes a necrotic process that significantly reduces the tensile strength. Leukocyte-derived collagenases further weaken the infarct area by breaking up connective tissue. As a compensatory mechanism to the increased wall stress (stretch), fibroblasts proliferate and differentiate into myofibroblasts, which align along the ventricular wall, providing a partial contraction during systole and producing large amounts of collagen. These changes provide an opposing resistance to the systolic forces that would promote outward excursion of the infarct zone and rupture. Inflammatory cytokines, however, inhibit fibroblast proliferation and collagen synthesis, so unresolved inflammation delays infarct healing and repair (see also Chapter 4 ).
In the pre-reperfusion era, when infarcts were larger and more likely to be fully transmural, the incidence of cardiac rupture 3 to 5 days after MI was not negligible, with reported rates of 1% to 2%. With prompt reperfusion, the wavefront phenomenon of ischemic death is aborted, and the infarct more often involves 25% to 75% of the ventricular wall, leaving an epicardial rim of viable myocardium, and making cardiac rupture rather rare (less than 0.1%).
Another critical role of the fibroblast is the synthesis of collagen to progressively substitute the infarct area with a fibrotic scar. Although impairment in collagen deposition reduces tensile strength and promotes dilation and aneurysm formation, excessive collagen deposition may promote HF by increasing ventricular stiffness, leading to diastolic dysfunction and impaired filling.
Leukocytes
Leukocytes occupy a central role in the tissue response to injury (see also Chapter 4 ). During MI, myocardial inflammation peaks between 24 and 96 hours and then gradually resolves within 2 to 4 weeks. Injury is associated with the release of danger-associated molecular patterns triggering the formation of a macromolecular structure, the inflammasome, which initiates and amplifies the inflammatory response by releasing large amounts of IL-1β and IL-18. Although some degree of inflammation probably is necessary for normal healing, an exuberant inflammatory response promotes myocardial dysfunction, edema, infarct expansion, cell death in cardiomyocytes in the border zone, and worsening systolic and diastolic function. Resolution of the inflammatory response is a physiologic response that limits unnecessary edema, stiffness, and dysfunction. A delay in inflammation resolution, therefore, represents an additional potential pathogenetic mechanism of adverse ventricular remodeling.
Elevated levels of C-reactive protein (CRP) and other inflammatory biomarkers measured at hospital admission, during the first 2 to 5 days, or at discharge consistently predict adverse ventricular remodeling and HF. Elevated CRP levels independently predict impaired diastolic function in patients with acute MI, independent of impaired systolic function.
Determinants of Adverse Ventricular Remodeling
Timely Reperfusion and “No Reflow”
Infarct size is the single most important predictor of adverse remodeling, and it is linearly dependent on the amount of myocardial salvage by reperfusion (“time is muscle”). As such, lack of timely reperfusion due to ineffective treatment or delayed presentation portends an unfavorable prognosis. Impaired tissue-level reperfusion, or “no reflow,” relates to persistence of microvascular obstruction despite epicardial coronary artery patency. Reduction of time to reperfusion—including initiation of treatment before hospital arrival and optimization of antiplateletet and anticoagulant therapies—appears to favor more complete reperfusion, to prevent no-reflow, and to combat adverse remodeling changes (see Chapter 13 ). The exact duration of the myocardial salvage window in patients with MI is not established ( Figure 36-3 ). In the early fibrinolysis trials, the benefits of reperfusion were largest within the first 6 hours and minimal between 13 and 24 hours (see Chapter 15 ). By contrast, in a study in which primary PCI for MI was performed between 12 and 48 hours, primary PCI led to measurable myocardial salvage as compared with medical therapy and a subsequent survival benefit at 4-year follow-up versus medical therapy alone.

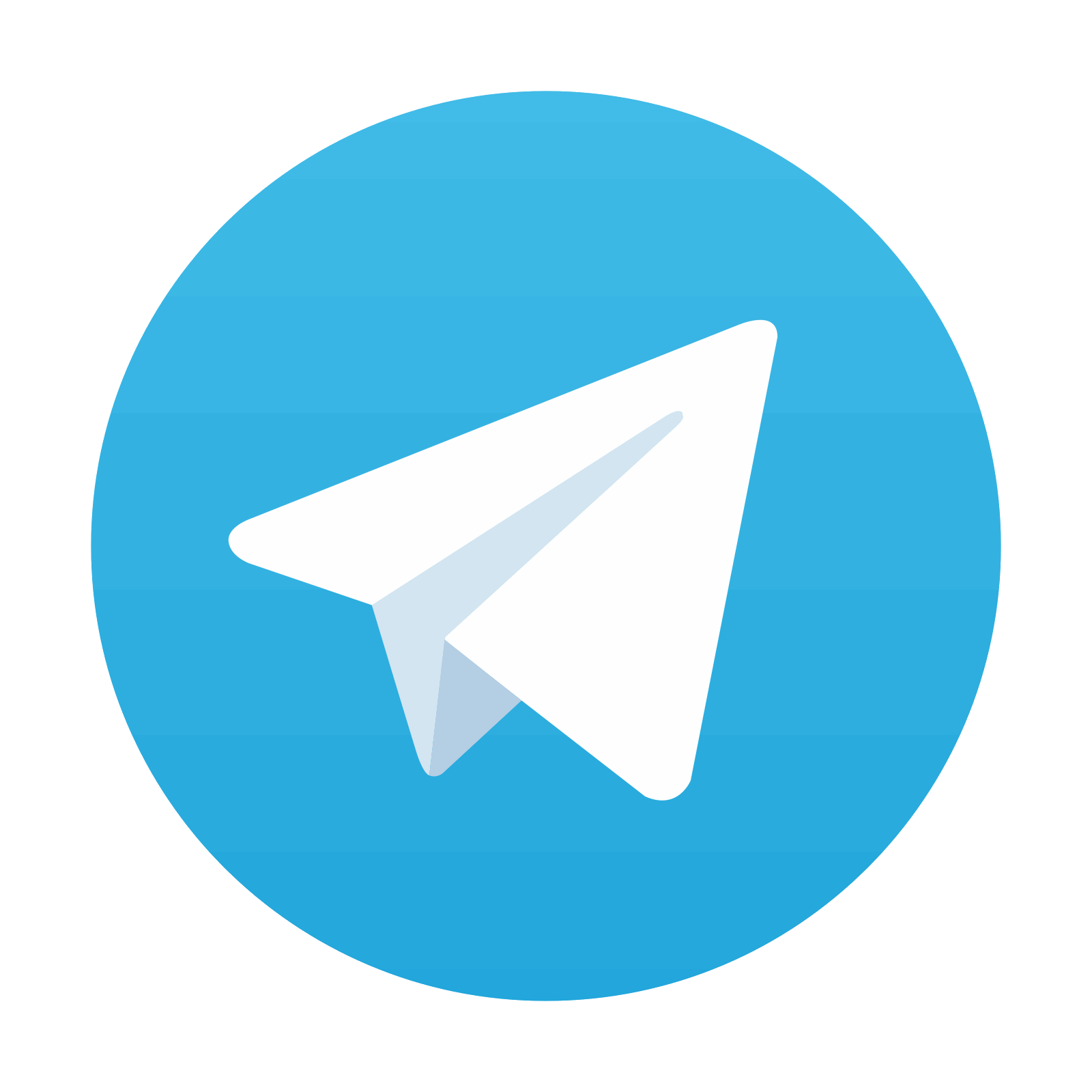
Stay updated, free articles. Join our Telegram channel

Full access? Get Clinical Tree
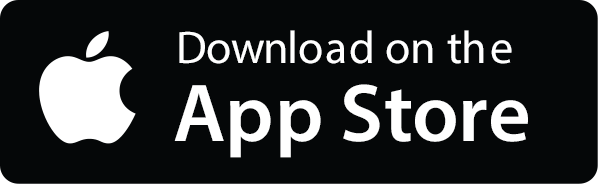
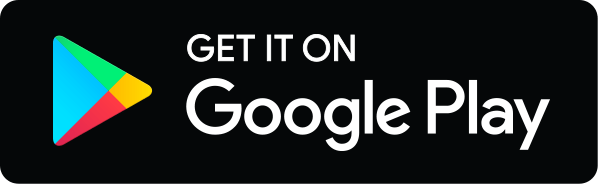
