Background
Although patients with hypertrophic cardiomyopathy (HCM) have normal ejection fractions at rest, the investigators hypothesized that these patients have differentially abnormal systolic function reserves, limiting their exercise capacity compared with patients with hypertension (HTN).
Methods
Forty patients with HCM (mean age, 39.1 ± 12 years), 20 patients with HTN with LVH, and 33 healthy individuals underwent resting and peak exercise echocardiography using two-dimensional strain imaging. Peak longitudinal systolic strain (ε sys ) and strain rate were measured in apical views. Circumferential ε sys and left ventricular (LV) twist were analyzed from short-axis views. LV systolic dyssynchrony was measured from regional longitudinal strain curves as the standard deviation of time to peak strain (time from the beginning of the Q wave on electrocardiography to peak ε sys ) between 12 segments. The differences between resting and peak exercise values were analyzed, and functional reserve was calculated as the difference divided by the resting value.
Results
In patients with HCM, resting values for longitudinal ε sys , systolic strain rate, early diastolic strain rate, and atrial diastolic strain rate were significantly lower, while circumferential ε sys and twist were higher, compared with patients with HTN and controls ( P < .0001). Functional systolic reserve increased during exercise in controls (17 ± 6%), increased to a lesser extent in patients with HTN (10 ± 16%), and was markedly attenuated in patients with HCM (−23 ± 28%) ( P < .001). At peak exercise, even with augmented circumferential ε sys and twist in patients with HCM ( P < .01) compared with those with HTN, both remained lower than in controls ( P < .001). LV dyssynchrony was amplified during exercise in patients with HCM compared with those with HTN ( P < .001). Within the entire population, exercise capacity was clearly correlated with systolic functional reserve. However when taken separately, it was mainly related to resting LV dyssynchrony and diastolic function in patients with HCM, whereas it was linked to age and LV wall thickness in those with HTN.
Conclusions
Patients with HCM have significantly limited systolic function reserve and more dynamic dyssynchrony with exercise compared with those with HTN. Two-dimensional strain imaging during stress may provide a new and reliable method to identify patients at higher cardiovascular risk.
Pathologic left ventricular (LV) hypertrophy (LVH), described in hypertrophic cardiomyopathy (HCM) and hypertension (HTN), is often associated with myocardial dysfunction and increased risk for sudden death, which is entirely different from physiologic adaptive growth as described, for example, in athlete’s heart.
On the other hand, HCM is a genetically heterogeneous disease resulting from sarcomeric protein mutations characterized by inappropriate myocardial hypertrophy (without identifiable etiology), interstitial fibrosis, myofiber disarray, disorganized myocardial architecture, and impaired LV performance. In contrast, pathologic LVH in HTN is the response of cardiomyocytes to pressure overload. This is not simply adaptive hypertrophy but a complex remodeling process driven by the responses of the cardiomyocytic and the noncardiomyocytic components of the heart to dynamic mechanical and neurohumoral stimuli. So, despite common features between the two diseases, the underlying diseased myocardium is completely different, with subsequent phenotypic, clinical, and functional diversity.
In LVH, subendocardial blood flow misdistribution related to hypertrophy and increased wall stress and the changes in myocyte architecture contribute to subendocardial dysfunction and declines in long-axis function. Besides, it has been shown in some LVH cohorts, as with HTN and aortic stenosis, that alterations in LV circumferential function may not parallel those in longitudinal function, particularly at rest. Both functions can be reliably quantified by the measurement of myocardial deformation using two-dimensional (2D) strain imaging during exercise.
Recently, it has been shown that small exercise-induced changes in the LV ejection fraction (EF) may identify a subset of patients who are at increased risk for cardiovascular events. The aim of the present study was thus to investigate LV systolic functional reserve using 2D strain imaging in patients with HCM and its relationship to exercise capacity and whether it is indeed able to discriminate between patients with HCM and those with HTN and LVH.
Methods
Study Population
Between September 2011 and November 2012, we prospectively included 40 patients with HCM (mean age, 39.1 ± 12 years) who were referred to our echocardiography laboratories for risk stratification. They were examined at a single center (Yacoub Research Unite, Menoufiya University). The diagnosis of HCM was based on conventional echocardiographic demonstration of a nondilated, hypertrophic left ventricle (≥15 mm) in the absence of other cardiac or systemic diseases capable of producing the magnitude of hypertrophy evident.
Twenty patients with HTN (mean age, 44.9 ± 11 years) with LVH on the basis of conventional echocardiographic demonstration of a hypertrophic left ventricle were included in the study. Subjects were considered to have HTN if they had resting systolic blood pressures > 140 mm Hg or diastolic blood pressures > 90 mm Hg or if they were on antihypertensive medications. Both patient groups were compared with 33 age-matched and sex-matched healthy subjects without detectable cardiovascular risk factors and not receiving any medications. Control subjects were volunteers recruited from among the hospital staff, medical and nursing students, and members of the local community. The entire studied population was enrolled after informed consent was obtained and after approval of the ethics committee of the university hospitals was obtained.
Exclusion Criteria
Patients with LV outflow tract obstruction ≥ 30 mm Hg at rest or provoked during exercise, EFs < 50%, prior myectomy or alcohol septal ablation, implantable cardioverter-defibrillators, diabetes mellitus, evidence of coronary artery disease, atrial fibrillation, lung disease, and exercise-limiting diseases were excluded from the study.
Procedure
All patients and control subjects underwent 12-lead electrocardiography, conventional echocardiographic examinations, 2D strain imaging using Velocity Vector Imaging (Siemens Healthcare, Erlangen, Germany), and Bruce-protocol treadmill exercise testing.
Conventional Echocardiography
Echocardiographic images were obtained in the parasternal long-axis and short-axis and apical two-chamber and four-chamber views using standard transducer positions. The Esaote MyLab Gold ultrasound system (Esaote SpA, Florence, Italy) equipped with a 5-MHz phased-array transducer was used. LV dimensions and wall thickness, EF, and left atrial diameter and volume were measured in accordance with the recommendations of the American Society of Echocardiography. Continuous-wave Doppler was used to diagnose resting obstruction, by estimation of pulmonary artery pressure from tricuspid regurgitation velocity (using the Bernoulli equation). Peak early (E) and late (A) transmitral filling velocities were measured from mitral inflow velocities. Early diastolic (Em) velocity was obtained by placing a tissue Doppler sample volume at the septal and lateral mitral annulus in the apical four-chamber view, and the mean value was obtained. The E/Em ratio was also calculated.
Analysis of LV Deformation
Strain measurement was based on Velocity Vector Imaging. Global myocardial deformation was evaluated from standard 2D images at a frame rate of 70 ± 20 frames/sec at rest, adjusted depending on heart rate to 92 ± 23 frames/sec during exercise. The images were stored, during three cardiac cycles, in digital format for subsequent offline analysis. To avoid excessive translational motion seen during stress testing, the clips were always captured with complete breath holding during expiration. Tracking and subsequent strain calculations were performed using the software package XStrain (Esaote SpA), on the basis of a previously validated algorithm. Scanning was performed from the apex to acquire the best apical two-chamber and four-chamber views. Peak longitudinal systolic strain (ε sys ), systolic strain rate (SR sys ), early diastolic strain rate (SR e ) and atrial diastolic strain rate in the basal, mid, and apical segments of the septal, lateral, anterior, and inferior walls were measured and averaged to calculate global longitudinal deformation.
Circumferential strain and LV twist and untwist rate were assessed from short-axis views at the basal and apical levels. Care was taken to ensure that the basal short-axis plane contained the mitral valve and that the apical plane was acquired distally to the papillary muscle with the left ventricle as circular as possible and proximal to the level with luminal obliteration at end-systole. LV twist was calculated as the instantaneous difference between apical and basal rotation.
To estimate LV mechanical dyssynchrony, time to peak strain (TTP) was measured from regional longitudinal strain curves for each ventricular segment, as the time from the beginning of the Q wave on electrocardiography to the time of peak ε sys . Electromechanical delay was measured as the difference of TTP in 12 LV myocardial segments (the difference between the longest and shortest TTPs). LV dyssynchrony was defined as the standard deviation of the averaged TTP (TTP-SD). Stress echocardiographic images were acquired immediately at peak exercise (within 1 min) and focused on the analysis of LV deformation.
Variability Study
Using Velocity Vector Imaging, the ventricles of 22 participants were assessed by two expert echocardiographers blinded to each other’s findings. The coefficient of variability was calculated as the standard deviation expressed as a percentage of the mean value of two sets of paired observations. Intraobserver and interobserver variability, respectively, were as follows: longitudinal ε sys , 2.3 ± 0.25% and 2.6 ± 0.25%; longitudinal strain rate, 0.19 ± 0.04 and 0.22 ± 0.04 sec −1 ; circumferential ε sys , 2.12 ± 0.27% and 2.5 ± 0.3%; and TTP, 3.02 ± 0.7 and 3.5 ± 0.2 msec.
Exercise Testing
A standard multistage symptom-limiting exercise test was conducted using a motorized treadmill (MyFormula, RAM model 770M; Esaote SpA) according to the Bruce protocol. Patients were asked to withhold β-blockers (if any) ≥24 hours before stress testing. Exercise tests were interrupted promptly when age-related maximum heart rate was reached or severe HTN (systolic blood pressure ≥ 250 mm Hg) or significant ventricular arrhythmia developed. Subjects were instructed to understand that they could stop voluntarily by indicating if they had any symptoms such as chest pain, intolerable fatigue, dizziness, breathlessness, and so on.
The electrocardiogram, heart rate, and blood pressure were recorded during the last minute of each stage of exercise and after exercise. The electrocardiogram was continuously monitored, and the 12 leads were continuously displayed on the monitor throughout exercise. Exercise capacity was determined as (1) metabolic equivalents (METs), where 1 MET = 3.5 mL/kg/min oxygen consumption, estimated on the basis of the protocol, speed, and grade achieved ; (2) the duration of exercise (exercise treadmill time [ETT]); and (3) the rate-pressure product (RPP), a measure of myocardial oxygen uptake during clinical exercise testing, estimated as the product of maximal achieved heart rate and systolic blood pressure.
Statistical Analysis
Categorical data are displayed as frequencies and percentages, and comparisons were made using χ 2 or Fisher’s exact tests as appropriate. Continuous data are expressed as mean ± SD and correlated using Spearman’s test and compared between groups using one-way analysis of variance or unpaired Student’s t tests as indicated. Linear regression analysis was used to identify independent predictors of RPP, METs, and ETT in patients with HCM. All echocardiographic variables were included in the model, in addition to resting and exercise longitudinal ε sys , SR sys , SR e , atrial diastolic strain rate, circumferential ε sys , TTP-SD, the magnitude of change in each value (Δ), and the percentage change (Δ/resting value). P values ≤ .05 were considered statistically significant. All statistical analyses were performed using SPSS version 20 (SPSS, Inc, Chicago, IL).
Results
Clinical Characteristics and Hemodynamics during Exercise
The clinical, echocardiographic, and baseline characteristics of the study population are depicted in Table 1 . At baseline, patients with HCM were more symptomatic and had a higher prevalence of asymmetric hypertrophy and mitral regurgitation compared with patients with HTN and controls ( P < .001). The level of exercise achieved was maximal in all control subjects and in 22 patients with HCM (55%) and 17 (85%) with HTN ( P < .01). More symptoms developed in patients with HCM than those with HTN during exercise ( Table 2 ). Four patients (10%) had exercise-induced hypotension (reduction of systolic blood pressure at peak exercise ≥ 20 mm Hg), and three (7.5%) had flat blood pressure responses. Mean exercise duration and METs were similar between the HCM and HTN groups, and both were lower compared with controls ( P < .001). Although eight patients with HCM (20%) had low exercise capacity (METs < 7), only two with HTN (10%) had exercise intolerance. During exercise, heart rates increased significantly ( P < .0001) in patients and control subjects, but the peak heart rate was significantly lower and systolic blood pressure during exercise was higher in patients with HTN compared with those with HCM and controls ( P < .001). Meanwhile, the RPP was significantly lower in patients with HCM compared with those with HTN and controls ( P < .001).
Variable | Controls ( n = 33) | Patients with HTN ( n = 20) | Patients with HCM ( n = 40) |
---|---|---|---|
Age (y) | 42.4 ± 12 | 44.9 ± 11 | 39.1 ± 12 |
Men | 24 (72.7%) | 13 (65%) | 29 (72.5%) |
BSA (m 2 ) | 1.86 ± 0.15 | 1.9 ± 0.19 | 1.85 ± 0.18 |
NYHA class | |||
I | 33 (100%) | 14 (70.0%) | 5 (15.2%) ∗ |
II | 6 (30.0%) | 17 (51.5%) ∗‡ | |
III | 11 (33.3%) ∗‡ | ||
LVH type ∗ | |||
Asymmetric | 8 (40%) | 31 (77.5%) ‡ | |
Concentric | 12 (60%) | 9 (22.5%) ‡ | |
LA diameter (mm) | 30.9 ± 4.1 | 33.1 ± 4.6 | 37.7 ± 6.8 ∗ |
LA volume (mL) | 22.0 ± 8.7 | 41.2 ± 18 ∗ | 70.7 ± 26 †‡ |
LV ESD (mm) | 32.5 ± 5.4 | 26.9 ± 6.3 ∗ | 22.0 ± 5.9 ∗ |
LV EDD (mm) | 45.9 ± 6.4 | 43.7 ± 8.2 | 35.9 ± 6.6 ∗‡ |
LV EF (%) | 67.8 ± 13.4 | 69.0 ± 8.4 | 71.3 ± 10.8 ∗ |
MWT (mm) | 9.6 ± 2.0 | 18.1 ± 4.3 ∗ | 27.7 ± 6.9 †‡ |
Septal thickness (mm) | 9.6 ± 2.0 | 17.8 ± 4.4 ∗ | 26.0 ± 6.0 †‡ |
LV PWT (mm) | 9.6 ± 2.1 | 14.5 ± 3.8 ∗ | 14.5 ± 3.4 ∗ |
LVMI (g/m 2 ) | 103 ± 16 | 195 ± 90 ∗ | 223 ± 73 †‡ |
LVOT gradient (mm Hg) | 3.9 ± 1.8 | 3.8 ± 1.6 | 19.8 ± 6.3 †‡ |
Mitral E/A ratio | 1.13 ± .1 | 1.07 ± .2 | 0.98 ± .6 |
DT (sec) | 187 ± 34.0 | 178 ± 54.5 | 216 ± 80.6 ∗‡ |
PAP (mm Hg) | 22.0 ± 3.1 | 23.6 ± 4.4 | 24.1 ± 7.4 |
E/Ea ratio | 4.5 ± 1.0 | 7.3 ± 3.2 | 11.6 ± 4.2 ∗‡ |
Variable | Controls ( n = 33) | Patients with HTN ( n = 20) | Patients with HCM ( n = 40) |
---|---|---|---|
Rest HR (beats/min) | 82 ± 8.7 | 75 ± 9.5 | 70 ± 11 |
Exercise HR (beats/min) | 165 ± 1 | 138 ± 13 † | 142 ± 21 ∗‡ |
Rest SBP (mm Hg) | 118 ± 3 | 137 ± 19 ∗ | 128 ± 22 ∗‡ |
Exercise SBP (mm Hg) | 155 ± 7 | 169 ± 28 † | 145 ± 32 ∗‡ |
Rest DBP (mm Hg) | 79 ± 4 | 91 ± 10 ∗ | 81 ± 14 ‡ |
Exercise DBP (mm Hg) | 97 ± 4 | 100 ± 12 | 82 ± 12 ∗‡ |
RPP | 28,000 ± 24,330 | 23,2480 ± 43,26 ∗ | 20,9912 ± 65,809 ∗‡ |
ETT (min) | 9.2 ± 1.7 | 6.7 ± 2.4 ∗ | 7.3 ± 3.0 ∗ |
METs | 10.45 ± 1.8 | 7.95 ± 2.0 ∗ | 8.32 ± 3.2 ∗ |
Reason for termination | |||
Chest pain | 0 (0%) | 2 (10%) | 7 (17.5%) ∗‡ |
Dyspnea or fatigue | 0 (0%) | 5 (25%) | 14 (35%) ∗‡ |
Achieved target HR | 33 (100%) | 17 (85%) | 22 (55%) |
LV Deformation
The absolute values of longitudinal ε sys and SR sys were significantly smaller in patients with HCM and in those with HTN than in controls at the segmental and global levels both at rest and during peak exercise ( P < .001; Table 3 , Figures 1 and 2 ). Change in ε sys was also less in patients with HCM ( Figure 3 ), and the functional reserve (Δ/resting value) was also reduced. There was even a reduction of global strain of −23.5 ± 28% during peak exercise, compared with an increase of 10.2 ± 16% in patients with HTN and a 17.7 ± 6% increase in ε sys in control subjects during peak exercise ( P < .001). Among our patients, 32 of those with HCM (80%) had decreases in ε sys at peak stress, compared with five of those with HTN (25%). Furthermore, exercise SR sys was significantly reduced in patients with HCM by −15 ± 24%, increased by 25 ± 13% in those with HTN, and was significantly augmented by 40 ± 9% in healthy subjects ( P < .0001). In patients with HCM, SR e was significantly lower at rest compared with patients with HTN and controls and showed a greater reduction at peak exercise ( P < .001). There was a reduction of SR e of −10 ± 31% in patients with HCM compared with increases of 12 ± 27% in patients with HTN and 23 ± 7% in controls ( P < .001). Although atrial diastolic strain rate was significantly lower at peak exercise in the HCM group ( P < .001), the magnitude of changes and percentage change in the rate of deformation in late diastole did not differ between groups.
Variable | Controls ( n = 33) | Patients with HTN ( n = 20) | Patients with HCM ( n = 40) |
---|---|---|---|
Rest ε sys (%) | −18.5 ± 2.0 | −15.8 ± 3.7 ∗ | −13.5 ± 5.6 †‡ |
Exercise ε sys (%) | −23.1 ± 2.7 | −17.7 ± 2.4 ∗ | −11.8 ± 4.9 †‡ |
Δ ε sys (%) | 3.40 ± 1.13 | 1.09 ± 3.7 ∗ | −3.23 ± 5.0 †‡ |
% ε sys | 17.69 ± 6.2 | 10.2 ± 16.1 ∗ | −23.5 ± 28.2 †‡ |
Rest circumferential ε sys (%) | −19.3 ± 2.5 | −23.9 ± 2.9 ∗ | −22.8 ± 3.4 ∗ |
Exercise circumferential ε sys (%) | −24.7 ± 2.4 | −24.5 ± 3.4 | −25.8 ± 6.2 |
Δ circumferential ε sys (%) | 5.3 ± 1.2 | 1.7 ± 2.1 † | 2.3 ± 3.0 ∗‡ |
% circumferential ε sys | 27.4 ± 9.3 | 7.3 ± 5 † | 10.8 ± 4.3 †‡ |
Rest TTP-SD (msec) | 28 ± 7.5 | 28 ± 12.7 | 52 ± 28.9 ∗‡ |
Exercise TTP-SD (msec) | 20.9 ± 12 | 30 ± 20 ∗ | 60 ± 37 †‡ |
Δ TTP-SD (msec) | −7.2 ± 5.2 | 1.8 ± 7.3 | 7.8 ± 8.1 †‡ |
Rest SR sys (sec −1 ) | −1.25 ± .23 | −.91 ± .28 ∗ | −.78 ± .34 ∗‡ |
Exercise SR sys (sec −1 ) | −1.65 ± .26 | −1.14 ± .19 ∗ | −.94 ± .91 †‡ |
Δ SR sys (sec −1 ) | 0.6 ± 0.7 | 0.23 ± 0.21 | 0.08 ± 0.3 ∗‡ |
% SR sys | 40 ± 9 | 25.2 ± 13 ∗ | −15.4 ± 24 ∗‡ |
Rest SR e (sec −1 ) | 1.52 ± .89 | 0.82 ± .4 ∗ | 0.95 ± .6 †‡ |
Exercise SR e (sec −1 ) | 1.84 ± 1 | 0.92 ± .44 ∗ | 0.85 ± .2 ∗‡ |
Δ SR e (sec −1 ) | 0.32 ± 0.09 | 0.10 ± 0.3 ∗ | −0.10 ± 0.4 ∗ |
% SR e | 23.8 ± 7 | 12.2 ± 27 ∗ | −10.5 ± 31 †‡ |
Rest SR a (sec −1 ) | 0.61 ± .34 | 0.53 ± .4 | 0.48 ± .2 |
Exercise SR a (sec −1 ) | 0.89 ± .67 | 0.80 ± .6 | 0.62 ± .2 ∗‡ |
Δ SR a (sec −1 ) | 0.37 ± 0.9 | 0.34 ± 0.2 | 0.45 ± 2.2 |
% SR a | 31 ± 14 | 32 ± 33 | 29 ± 38 |
Rest twist (°) | 10 ± 1.9 | 11.2 ± 1.5 | 13 ± 2.4 ∗§ |
Exercise twist | 14.3 ± 2.2 | 12.1 ± 2.1 | 14.2 ± 2 § |
Rest twist rate (sec −1 ) | 66 ± 13 | 86 ± 12 ∗ | 94 ± 18 †§ |
Exercise twist rate | 131 ± 18 | 111 ± 12 ∗ | 121 ± 13 ∗‡ |
Rest untwist rate | −70 ± 17 | −69.2 ± 13 | −72 ± 12 |
Exercise untwist rate | −132 ± 24 | −119 ± 14 † | −118 ± 20 † |
‡ P < .001 versus patients with HTN.


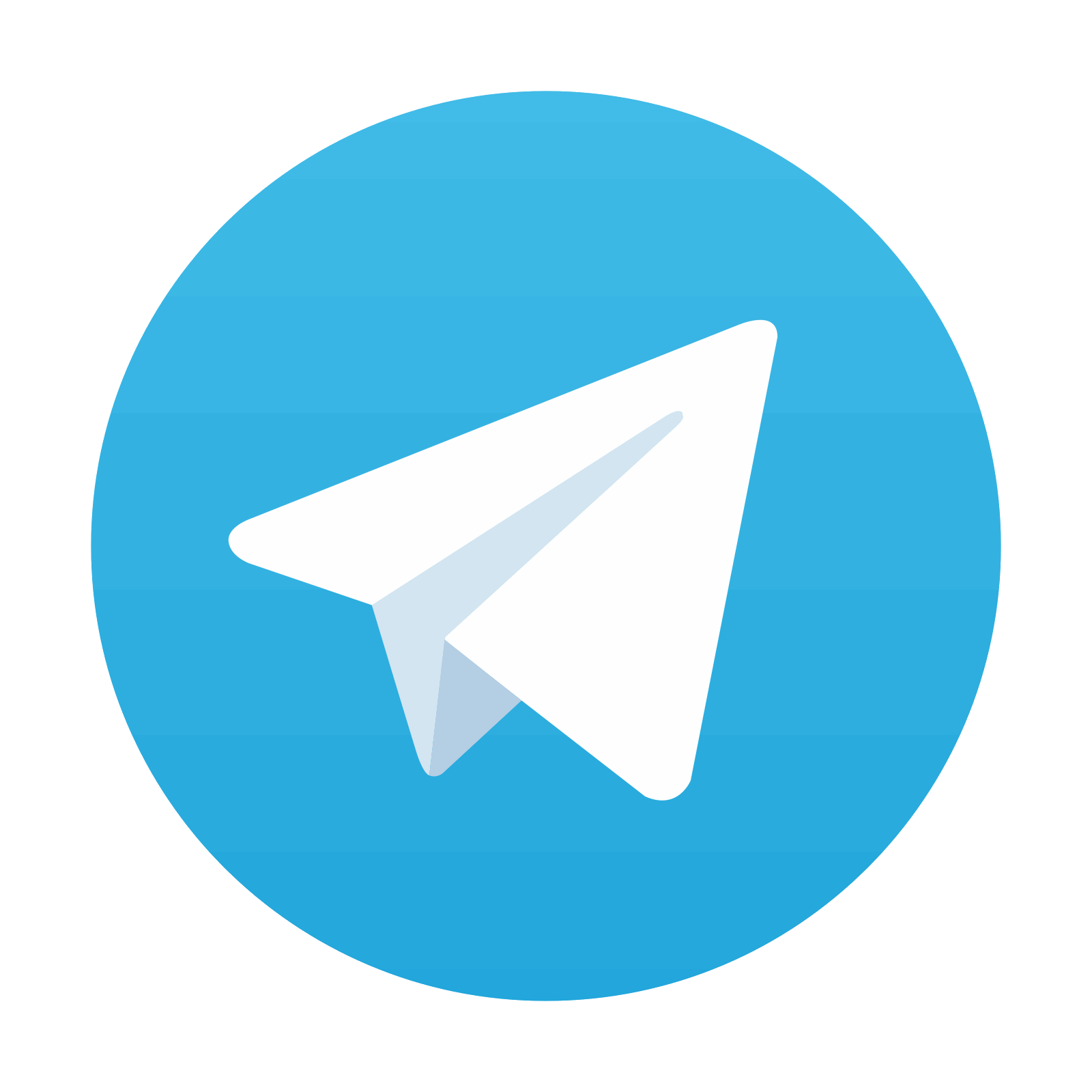
Stay updated, free articles. Join our Telegram channel

Full access? Get Clinical Tree
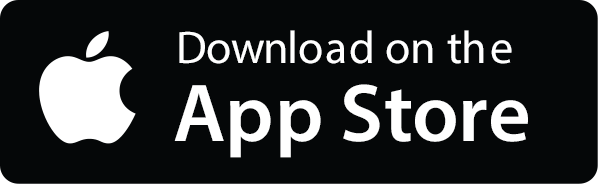
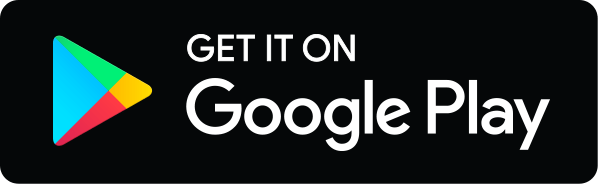
