Abstract
The field of human genetics has achieved dramatic growth since the publication of the second edition of this textbook. The capabilities of genetic testing technology to interrogate the genome have advanced rapidly, leading to fundamental changes in the way clinicians approach the diagnosis and treatment of many human diseases. One example of this ongoing growth in pediatric intensive care is the adoption of routinely testing infants in the cardiac intensive care unit with chromosomal microarray analysis. This growth has led to improved understanding of the genetic cause of many syndromic diseases and has begun to identify novel disorders based on molecular diagnoses. At the same time, clinical studies have advanced understanding of the clinical implications of genetic diagnoses in the cardiac intensive care unit (ICU). This chapter begins with an overview of the most common syndromes encountered in the pediatric cardiac ICU with a focus on the cardiac and noncardiac factors that influence the clinical course of patients. Next, we explore current approaches to genetic testing in the cardiac ICU and recent contributions of novel genetic testing technologies. Finally, we highlight the complex nature of implementing genetic testing in the ICU and the importance of multidisciplinary approaches that integrate genetic counseling and geneticists with the intensive care team.
Key Words
Congenital heart disease, genetic syndrome, trisomy 21, 22q11.2 deletion syndrome, Turner syndrome
The association between congenital cardiovascular malformations (CVMs) and genetic syndromes is well established. Traditionally, a characteristic constellation of clinical features has defined a genetic syndrome. The list of syndromes associated with CVMs is expansive and includes disorders caused by different classes of genetic abnormalities. These include abnormality of chromosome number or large structural chromosomal defects. Down syndrome, which is caused by the presence of three copies of chromosome 21, is the most common aneuploidy syndrome among patients born with CVMs. Alternatively, syndromic CVMs may be caused by microdeletions or microduplications, which encompass smaller chromosomal regions. DiGeorge syndrome, which is caused by the presence of a deleted segment on the long arm of one of the copies of chromosome 22 (22q11.2 deletion), is a common example. A third mechanism known to cause syndromic CVMs is the alteration of DNA sequence at the nucleotide level, which includes single nucleotide variants (SNVs) and small insertions or deletions. Noonan syndrome, which is typically caused by pathogenic SNVs within genes involved in the RAS-MAP kinase signaling pathway (e.g., PTPN11 ), is an example. In addition to the genetic syndromes associated with CVMs, it is increasingly clear that many cases of isolated, or nonsyndromic, CVMs also have a primary genetic basis. For example, there are many reports of multigenerational families with isolated CVMs showing Mendelian inheritance patterns or complex inheritance patterns. Furthermore, large epidemiologic studies confirm increased rates of familial recurrence of CVMs across the general population of patients with CVMs.
The field of human genetics has grown expansively since the publication of the second edition of this textbook. Novel genetic testing technologies coupled with clinical insight have fundamentally changed the way clinicians approach the diagnosis of many diseases. The basic categorization of disease, including syndromic disease, continues to improve by combining the established constellations of clinical signs and symptoms with specific molecular diagnosis. As an example of one major shift in the genetic evaluation of patients with CVMs, chromosomal microarray analysis (CMA) is increasingly used in many pediatric cardiac intensive care units (ICUs) to identify small deletions and duplications of chromosomal material, termed copy number variants (CNVs), across the genome. In addition, next-generation sequencing (NGS) technology now facilitates interrogation of the genome for nucleotide sequence variants across multiple genes in parallel. “NGS panels” apply this technology to test a defined set of genes that are known to be associated with the patient’s clinical presentation (e.g., Noonan syndrome panels test for all the genes known to cause Noonan syndrome simultaneously). Alternatively, NGS technology can be leveraged to sequence all of the coding regions of the genome (exome) or the entire genome in an increasingly time- and cost-efficient manner. This rapid expansion of genetic testing technology and clinical implementation not only has led to the discovery of new genetic diseases, but has significantly advanced our understanding of the molecular basis of previously described clinical syndromes. The availability and increasing affordability of CMA, NGS panels, and exome sequencing tests have accelerated advancements made in the research setting and are being integrated into routine clinical management of CVMs and other forms of pediatric cardiovascular disease.
The clinical practitioner who cares for critically ill patients with CVMs will encounter many patients with genetic syndromes. The syndromic diagnosis may already be established either by prenatal or postnatal evaluations before arriving at the operating room or ICU, or, especially in neonates, the patient may not have a diagnosis yet established at the initial encounter. In either case, it is important not only to understand the genetic cause of these syndromes, but also to be familiar with the clinical features that are typically encountered. This allows providers to predict certain risks and preemptively intervene and may offer the patient and/or family information regarding prognosis, risk of familial recurrence, and identification of occult disease in relatives.
This chapter begins with an overview of the interaction between the most common genetic syndromes associated with CVMs and the management of cardiac disease in the ICU, including the important considerations of noncardiac factors. This will include some discussion of clinical outcomes data. A comprehensive review of the impact of genetic syndromes on perioperative outcomes is available to the interested reader. Next, we explore our current understanding of the clinical utility of genetic testing in newborns with critical CVMs and highlight recent discoveries related to the genetic basis of syndromic and nonsyndromic CVMs using NGS technology. The chapter includes advisement for the routine involvement of genetics services within the pediatric cardiac ICU and surgical programs. The chapter focuses specifically on the genetic considerations for patients with CVMs, so readers should seek other resources for discussions of the genetics of other forms of pediatric cardiovascular disease, including cardiomyopathy, aortopathy, and channelopathy, for which the availability of comprehensive and affordable multigene panels using NGS techniques, has been a cornerstone of staggering growth in pediatric cardiology.
Syndromic Congenital Cardiovascular Malformations and the Factors Influencing Intensive Care
Large studies of pediatric patients with CVMs who require ICU care and/or cardiac surgery find that 10% to 30% of cases have a genetic diagnosis or noncardiac abnormalities. The true burden of syndromic disease is not completely known due to differences in genetic testing and evaluation practices among studies. Other factors, such as selection of certain age ranges or type of CVMs, may differ between studies and lead to differences in the reported prevalence of disease. These factors notwithstanding, given the high burden of syndromic disease, an important question is whether having a syndromic diagnosis in general influences perioperative outcomes of pediatric patients undergoing cardiac surgery. This question has been assessed by several studies using large cohorts of patients treated over the past two to three decades. The most consistent conclusion among these studies is that a genetic diagnosis significantly increases the risk for postoperative morbidities such as increased risk for respiratory, infectious, and renal complications, resulting in prolonged hospitalization. These complications may have long-standing, deleterious effects upon the child’s health in addition to causing increased burden on the family and health care system.
Increased hospital mortality following a cardiac operation has been observed in some, but not all, studies. As one may expect, there is strong evidence to support that the risk for mortality is increased for syndromic patients requiring more complex operations. For example, data from the Society of Thoracic Surgeons (STS; years 2002 to 2006) and the Congenital Heart Surgeons’ Society (years 1994 to 2001) showed increased postoperative mortality among patients with a genetic or noncardiac congenital anomaly following stage I palliation for left ventricular outflow tract lesions such as hypoplastic left heart syndrome (HLHS). The design of this study highlights a common approach to batch groups of patients with a genetic syndrome together with those having a noncardiac congenital anomaly into one “syndromic” group. Although this approach may increase the power to detect differences via study of larger groups of patients, understanding the risk for specific syndromes is more practically useful. Ideally outcomes data pertaining to specific syndromes will become increasingly available as the use of genetic evaluations for patients with CVMs expands, facilitating the study of larger syndrome-specific cohorts and more detailed classification of patients. Fortunately, modern outcomes data have become available for several of the most common syndromes associated with CVMs. We explore these syndromes in the remainder of this section.
Down Syndrome
Down syndrome, or trisomy 21, is the most frequent genetic syndrome among children born with a congenital CVM. It is estimated to affect between 200,000 and 300,000 individuals in the United States and occurs in at least 1 in 1000 live births. Congenital CVMs are present in 40% to 50% of affected children. Atrioventricular septal defect (AVSD) is the most common class of CVM identified (50% to 60%), followed by ventricular septal defect (VSD), atrial septal defect (ASD), and tetralogy of Fallot. Because of the high incidence of CVMs, all infants with known or suspected Down syndrome should have a comprehensive cardiac evaluation, including echocardiography, within the first few days to weeks of life. Many other organ systems are affected in Down syndrome ( Fig. 75.1 ), but the prominent characteristics include impaired cognition, delayed growth, and facial dysmorphism. Other problems of significant importance in the intensive care/surgical setting include upper airway obstruction, atlantoaxial instability, hypothyroidism, and immune deficiency. Congenital anomalies of the gastrointestinal system, including duodenal atresia, Hirschsprung disease, or tracheoesophageal fistula, occur at increased frequency. In spite of these factors, the average life expectancy for individuals with Down syndrome now exceeds 50 years. However, these patients also face risks for the development of leukemia and early-onset Alzheimer’s disease.

The cytogenetic abnormality responsible for Down syndrome is the trisomy of chromosome 21 (47, +21) in 93% to 96% of cases or a translocation involving chromosome 21 in 2% to 5% of cases. The diagnosis is typically established using the traditional karyotype. Approximately 2% to 4% of cases are associated with mosaicism due to postzygotic nondisjunction, in which case the clinical findings are usually milder. Although advanced maternal age is a risk factor, the majority of mothers bearing children with Down syndrome are between 18 and 35 years of age. If a balanced translocation is identified in a parent, genetic counseling should be performed to discuss that there is increased risk of recurrence in future offspring compared with the more typical cases caused by sporadic nondisjunction. At this time the precise pathogenetic mechanism by which trisomy of chromosome 21 leads to the Down syndrome phenotype remains incompletely understood.
A significant risk for patients with Down syndrome is the development of pulmonary vascular disease (PVD). This risk is particularly high among those with nonrestrictive interventricular communication such as complete AVSD or large VSD. For example, some studies suggest that more than one-third of patients with trisomy 21 and a CVM have concomitant pulmonary artery hypertension. Chronic hypoventilation secondary to upper airway obstruction and sleep apnea may significantly contribute to the development of pulmonary hypertension. This must be dutifully managed to avoid exacerbating what is likely an intrinsic predisposition to develop PVD (see Fig. 75.1 ). The risk for early development of PVD has led to the practice of early repair of large left-to-right interventricular shunts, including complete AVSDs, usually by age 6 months. Airway and craniofacial anomalies may further complicate preoperative and postoperative management by creating difficulty with intubation, secretion clearance, atelectasis, and chylothorax, leading to risk for prolonged mechanical ventilation. The palliation of single-ventricle lesions may be particularly precarious for patients with Down syndrome and PVD. Pulmonary hypertension and PVD may be major contributors to the increased mortality observed in patients with Down syndrome after stage I, II, and III palliations. Preoperative evaluations and postoperative protocols may be useful for the management of patients with Down syndrome and pulmonary hypertension.
Other important noncardiac problems that may complicate intensive care include atlantoaxial instability, immune deficiency, and hypothyroidism. Atlantoaxial instability may pose a risk of neurologic injury during intubation, particularly for older children and young adults. Dysfunction of the humoral or innate immune systems may predispose to postoperative infections, but whether this risk warrants alterations of typical postoperative antibiotic prophylaxis is not established. Hypothyroidism often develops in the first year of life. Because thyroid hormone contributes significantly to cardiovascular stability, it is good practice for all patients with Down syndrome to be screened for hypothyroidism in the time leading up to cardiac surgery and, if diagnosed, to receive adequate outpatient treatment before the operation. Despite the various health challenges associated with Down syndrome and established risk for postoperative complications, recent series including large numbers of patients indicate that hospital mortality after biventricular repairs, including complete AVSD, may be unchanged or even decreased compared with patients without Down syndrome. For all patients with Down syndrome a planned approach to timing and coordination of the health care team members involved is crucial for the child and family.
22q11.2 Deletion Syndrome
22q11.2 deletion syndrome occurs in approximately 1 in 4000 individuals and is the most common microdeletion syndrome associated with congenital CVMs. Nearly 75% of patients with 22q11.2 deletion syndrome have a CVM, which most commonly include conotruncal defects, abnormal patterning of the aortic arch and brachiocephalic arteries, and perimembranous VSD ( Table 75.1 ). The systemic features include facial dysmorphism ( Box 75.1 ; Fig. 75.2 ) and cleft palate. Additional features likely to impact intensive care include hypoparathyroidism, immunodeficiency, congenital renal anomalies, and airway anomalies ( Box 75.2 ). The long-term challenges associated with 22q11.2 deletion include developmental delay and neuropsychiatric disorders such as attention-deficit/hyperactivity disorder, autism spectrum disorders, and psychosis.
Defect | Percentage |
---|---|
No defect or clinically insignificant | 22-25 |
Tetralogy of Fallot | 17-20 |
Interrupted aortic arch | 14-17 |
Ventricular septal defect (VSD) | 14-16 |
Pulmonary atresia with VSD | 10 |
Truncus arteriosus | 9 |
Aortic arch anomaly | 5 |
Vascular ring | 3 |
Pulmonary valve stenosis | 2 |
Other significant defect | 4-5 |
Narrow palpebral fissures
Hooded eyelids
Hypertelorism
Small, rounded protuberant ears
Broad nasal bridge
Bulbous nose tip
Hypoplastic alae nasi
Small mouth
Retrognathia/micrognathia
Palatal abnormalities
Long thin digits

Difficult intubation
Laryngeal/tracheal abnormalities
Hypocalcemia
Seizures
Increased risk of infections
Possibility of transfusion-related graft-versus-host disease
Feeding difficulties
The 22q11.2 deletion syndrome is caused by hemizygous deletion of a small region of variable length on the short arm of chromosome 22 and is associated with a range of clinical phenotypes. The deletion was initially discovered in patients with the clinical characteristics of DiGeorge syndrome, but it is now understood that the deletion also causes other clinically established syndromes, including velocardiofacial syndrome and conotruncal anomaly face syndrome. The shift to combining these syndromes nominally into a single 22q11.2 deletion syndrome exemplifies the ongoing integration between syndromic diagnoses and their underlying genetic causes. The genetic test traditionally used to detect 22q11.2 deletion was fluorescence in situ hybridization (FISH), which uses a probe designed to target this chromosomal segment specifically. Practices are now turning toward use of CMA, which has higher diagnostic sensitivity because it is able to detect smaller deletions that may not be identified with FISH. When 22q11.2 deletion is not deleted, the CMA will potentially identify alternative pathogenic CNVs and therefore reach a diagnosis more efficiently. The length of the segment affected by this microdeletion is variable but typically contains between 30 and 40 individual genes. Hemizygous loss of the TBX1 gene may play a major role in causing some features of this syndrome, including CVMs.
Hypoplasia of the thymus is often present in patients with 22q11.2 deletion syndrome, typically leading to mild to moderate immunodeficiency. Rarely, the thymus is completely aplastic, resulting in severe immunodeficiency. Thymic hypoplasia leading to low T-lymphocyte counts and B-lymphocyte dysfunction may explain reports of increased infectious complications following cardiac surgery. This may justify the use of broadened postoperative antibiotic regimens, including antifungal therapy, although this approach has not been conclusively tested. Further, the immunocompromised status of the patients justifies use of cytomegalovirus-negative, irradiated blood products when transfusions are required to avoid the risk of iatrogenic infection and graft-versus-host disease. Airway management may be challenging due to retrognathia or a congenital airway anomaly, including laryngeal web. Depending on the severity of the anomaly, the equipment and personnel necessary to establish an alternative airway should be readily available before the administration of hypnotics and neuromuscular blocking agents. Postoperative vocal cord paralysis, particularly after operations involving the aortic arch, or diaphragmatic paralysis can have dramatic clinical consequences and should be investigated and treated. Overt cleft palate, submucous clefts, and velopharyngeal insufficiency may significantly impair swallow function. Hypocalcemia due to developmental hypoplasia of the parathyroid glands may be present, particularly in young infants, and tends to resolve or respond to calcium supplementation. Calcium level should be monitored preoperatively and postoperatively, especially given that patients with 22q11.2 deletion have increased seizure risk independent of the presence of hypocalcemia. Renal abnormalities detectable on ultrasonography, including absent, dysplastic, or multicystic kidneys, are another common finding.
The multiple noncardiac abnormalities associated with 22q11.2 deletion often complicate the postoperative course, leading to longer duration of postoperative intensive care. Although few studies are sufficiently powered to examine patients with 22q11.2 deletion syndrome in terms of mortality, most data support that there is a slightly increased risk of death following a cardiac operation. Mortality risk may be most significant for patients with pulmonary atresia, in which cases excessive bleeding may be a significant risk. This bleeding risk may be secondary to increased anatomic complexity, but dysfunction of hemostatic pathways, including platelet function, may be contributory. Altogether, the broad spectrum of noncardiac systemic anomalies in patients with 22q11.2 deletion syndrome highlights the importance of a multidisciplinary approach involving multiple pediatric specialists in the newborn, perioperative, and ambulatory setting. Box 75.3 lists the recommended specialties for the care team. These are ultimately tailored to match the spectrum of problems in an individual, once appropriate screening evaluations and testing have been completed.
Typical Subspecialty Evaluation
Genetics
Cardiology
Immunology
Otolaryngology
Plastic surgery
Speech and audiology
Neurodevelopmental
Oral motor and swallowing
Additional Subspecialties as Necessary
Endocrinology
Gastroenterology
Orthopedics
Urology
Pediatric surgery
Psychiatry
Laboratory Evaluation
Chromosomal microarray analysis
Serum calcium level
Complete blood count, T-cell studies, immunoglobulin concentrations
Echocardiography
Renal ultrasonography
Turner Syndrome
Approximately 1 in 2000 females is born with Turner syndrome, which is due to complete or partial absence of one of the two X chromosomes. Turner syndrome predisposes to left-sided CVMs such as bicuspid aortic valve and coarctation of the aorta. Many patients also manifest signs of arteriopathy, including thoracic aortic aneurysm and dissection (TAAD) and systemic hypertension. Venous anomalies, including partial anomalous pulmonary venous return and persistent left-sided superior vena cava, are also frequently encountered. The noncardiac features providing clues to the diagnosis are short stature, ovarian dysgenesis, neck webbing, and widely spaced nipples. There is general consensus that patients with Turner syndrome have intrinsic dysfunction of lymphatics. This may be one explanation for the high frequency of hydrops fetalis and spontaneous fetal loss. Peripheral lymphedema, which is typically present at birth and self-resolving by early childhood, may be another clue to early diagnosis. Most pertinent to intensive care is the risk for dysfunction of pulmonary lymphatics predisposing to chylothorax and pleural effusions. Congenital pulmonary lymphangiectasia may be the most drastic form of lymphatic dysfunction in Turner syndrome, but there is likely a spectrum of lymphatic abnormality that may manifest clinically only when confronted with stressors such as mechanical injury and/or inflammation associated with cardiopulmonary bypass surgery. Congenital renal anomalies occur frequently enough to mandate screening with ultrasonography. There is a long-term risk for development of autoimmune hypothyroidism, so patients with Turner syndrome should undergo routine screening and be tested before cardiac surgery if over 4 years of age.
Patients with Turner syndrome undergoing surgical repair of coarctation of the aorta have displayed good postoperative outcomes since the adoption of modern surgical techniques, which include careful manipulation of diseased aortic tissues to avoid postoperative hemorrhage. In contrast, consistent with arteriopathy, there is evidence that treatment of coarctation with implanted stents may introduce a risk for development of aneurysm or dissection. The most concerning outcomes data in Turner syndrome concern HLHS. For unknown reasons, patients with HLHS and Turner syndrome display very poor outcomes with mortality between stage I and stage II palliations reported to be as high as 80% and only 25% survival to stage III palliation. These poor outcomes may be related to underlying lymphatic disease, but there is a clear need for further study to understand the responsible mechanism.
To improve rates of early diagnosis, it is reasonable to perform a karyotype as a routine component of care for every female infant with coarctation. Recent data show testing yields approximately 5% to 12% in girls with coarctation, and external features may be subtle in the neonatal period or in cases of mosaicism. All patients with Turner syndrome require long-term cardiac surveillance for development of hypertension and TAAD, even in the absence of bicuspid aortic valve. Routine screening with cardiac magnetic resonance imaging in late childhood may identify clinically silent features, including aortic dilation and anomalous pulmonary veins. Thus an early diagnosis likely improves short- and long-term outcomes.
Noonan Syndrome
Noonan syndrome has a prevalence of 1 in 1000 to 2500 live births. Noonan syndrome is characterized by both cardiac and extracardiac defects ( Fig. 75.3 ). Noonan syndrome is genetically heterogeneous, but the currently known genes cluster within the RAS-MAP kinase signaling pathway, leading some to classify Noonan syndrome as a “RASopathy.” The most commonly mutated gene in this syndrome is PTPN11. Other causative genes in the pathway are SOS1, RAF1, KRAS, NRAS, BRAF, SHOC2, and CBL. Indeed, there are now several disorders related to Noonan syndrome with overlapping but clinically distinct phenotypes that are caused by mutations in the RAS-MAP kinase pathway. These include cardiofaciocutaneous syndrome (BRAF, KRAS) , Costello syndrome (HRAS) , and Noonan syndrome with multiple lentigines (formerly called LEOPARD syndrome; PTPN11 , RAF1 ). There are important clinical considerations for each of these syndromes. The focus of this chapter will be the typical Noonan syndrome.

In Noonan syndrome CVMs are observed in at least 80% of cases. Pulmonary valve stenosis, often with pulmonary valve dysplasia, is the most frequent cardiac anomaly, followed by hypertrophic cardiomyopathy (HCM). Secundum ASD is often associated with pulmonic stenosis, and a variety of other CVMs are reported. The noncardiac features of this syndrome include facial dysmorphism, short stature, kyphoscoliosis, vertebral anomalies, winged scapula, pectus sternal deformity, neck webbing, and cryptorchidism in males. Most cases represent de novo (i.e., not inherited) mutations. However, autosomal dominant familial presentations are also frequently encountered. Currently patients suspected to have Noonan syndrome are tested using an NGS panel designed to sequence all of the genes known to be associated with the clinical phenotype. When a molecular diagnosis of Noonan syndrome is established, screening of parents for the mutation should be strongly considered to identify previously unrecognized carriers who may lack obvious physical features.
Whether involved in the perioperative management of cardiac or extracardiac anomalies, intensive care and surgical providers should be cognizant of several features of Noonan syndrome. Abnormalities in coagulation or platelet function are common and may predispose to bleeding complications. Some of the initial descriptions of platelet function deficit and neonatal amegakaryocytic thrombocytopenia have been corroborated by subsequent reports. Although congenital thrombocytopenia is a rare occurrence, comprehensive testing of platelet function has identified abnormalities in greater than 80% of patients. In pioneering work Sharland and colleagues, studied 72 affected individuals with a mean age of 11.4 years and found that 47 (65%) had a history of abnormal bruising or bleeding and 29 (40%) had a prolonged activated partial thromboplastin time with deficiencies of factors VIII, XI, and XII. These findings were recently replicated in an independent cohort of 39 patients. Together these data suggest that preoperative assessment for coagulation-factor deficits and platelet dysfunction may be warranted. Providers should be alert to bleeding complications and exercise caution toward the use of antiplatelet medications.
Similar to Turner syndrome, Noonan syndrome may be complicated by disorders of the lymphatic system. Peripheral lymphedema is a common finding in neonates with Noonan syndrome that typically resolves early in life. However, lymphatic dysfunction may assume more severe forms, including lymphatic dysplasia or pulmonary lymphangiectasia. Recurrent pleural effusion is a rare but troublesome postoperative complication. This appears to be due to a nonspecific effect of thoracotomy in exacerbating previously mild chronic low-grade pulmonary lymphangiectasia. Good response is generally obtained with a combination of thoracentesis, restriction from dietary long-chain fatty acids, fluid restriction, and/or diuretics. Prenatal evidence of lymphedema or fluid accumulation, including fetal hydrops, may be another clue to the diagnosis.
In terms of the cardiovascular features of Noonan syndrome, the pulmonary valve is usually thickened and dysplastic. The short-term outcomes after balloon valvuloplasty do not appear significantly different from pulmonary valve stenosis in patients without Noonan syndrome, but long-term outcomes are unknown. HCM was originally described as developing late in childhood but has now been recognized in infancy and even prenatally. The presence of HCM, which is particularly high among patients with mutations in RAF1, increases anesthetic and intraoperative risk. A recent echocardiogram should be available before anesthesia for cardiac or noncardiac surgery in infants or children with Noonan syndrome. There is evidence that some patients with HCM and Noonan syndrome experience worse outcomes compared to non–Noonan syndrome HCM. A baseline preoperative electrocardiogram (ECG) is also recommended before surgery. ECG abnormalities, including negative forces in left precordial leads, left axis deviation, and abnormal Q waves, are often present and unrelated to the cardiovascular anatomic phenotype. Characterizing the ECG appearance at baseline may help to discern whether abnormalities observed on cardiac monitors during or after surgery represent acute pathology. Although Noonan syndrome does not predispose to cardiac arrhythmia, patients with the related Costello syndrome ( HRAS mutation) do have a significant risk for developing supraventricular tachycardia and multifocal atrial tachycardia in particular.
Most individuals with Noonan syndrome develop normal cognitive function. Indeed, most children are able to attend regular school. However, increased risk for long-term intellectual disability warrants close monitoring for early signs of developmental delay. In early reports there was concern for a possible association between Noonan syndrome and malignant hyperthermia, as postulated by Hunter and Pinsky. Fortunately, this connection has not been supported over time, and risk for malignant hyperthermia is considered to be no different from the general population.
With increased awareness and advances in genetic testing practices, including family screening, diagnoses are established earlier than in past series. Nonetheless the phenotypic variability, including subtlety of features and the changes in facial pattern with growth, still contributes to missed diagnoses. In the context of these diagnostic challenges, the pediatric intensivist confronted by a child with growth delay, pulmonary stenosis, and/or cardiomyopathy may be the first to recognize the syndrome in the child and in a parent.
Trisomy 18 (Edwards Syndrome)
Trisomy 18 is a severe genetic syndrome characterized by the presence of three copies of chromosome 18. Rarely this additional chromosome is present in only some cell lines, leading to a variable clinical presentation, referred to as mosaic trisomy 18. Trisomy 18 occurs in approximately 1 in 6000 live births and is the second most common trisomy syndrome after trisomy 21. Overall the clinical features of this syndrome are quite dramatic with a very high mortality (90% to 95% in the first year of life) coupled with severe cognitive impairment and diffuse multisystem anomalies among the approximately 50% who survive beyond the first week. Congenital CVMs are very common with a prevalence between 80% and 100%. Common cardiac lesions include VSD, ASD, and patent ductus arteriosus (PDA). More complex lesions such as tetralogy of Fallot, double-outlet right ventricle, polyvalvular dysplasia, and AVSDs are also encountered. Due to the severe impact of this syndrome on expected outcomes, rapid testing may be considered when the diagnosis is suspected postnatally.
Given the grim overall prognosis, including profound neurodevelopmental impairment, the role of cardiac intervention for patients with trisomy 18 has been a significant topic of discussion, and there is little consensus among congenital heart programs at present. There is significant debate surrounding the role of cardiac intervention in modifying mortality and morbidity for these patients. Recent studies have stirred significant debate surrounding this issue by showing optimistic short-term survival rates (80% to 90%) among the few trisomy 18 patients who undergo cardiac surgery. Others suggest that cardiac surgery and aggressive perioperative care may improve life expectancy and increase the likelihood of hospital discharge. Despite these observations it is clear that patient selection was a key aspect of the improvements seen in those who received cardiac surgery. Patients with low birth weight, unstable initial hospital course, very early mortality, and a high burden of noncardiac congenital anomalies and medical complications were significantly less likely to be offered surgical intervention. The appropriateness and utility of cardiac intervention for patients with trisomy 18 remain unclear. Individualized approaches to clinical decision making with the family in partnership with specialists in palliative care are most likely to achieve the best possible outcome for patients with this severe disease.
Heterotaxy Syndrome
Heterotaxy syndrome is characterized by disruption of normal thoracoabdominal situs and includes a spectrum of CVMs and noncardiac organ problems ( Fig. 75.4 ). This condition is discussed in detail in Chapter 67 of this textbook. Relevant to this chapter, the understanding of the genetic basis of heterotaxy is rapidly advancing. Genes in the Nodal signaling pathway, including DNAH5, ZIC3, CFC1, NODAL, ACVR2B, DNAI1, and LEFTY2, are known to harbor mutations that cause heterotaxy. NGS panels testing for these genes and others are now available clinically. Overall, the risk of familial recurrence of heterotaxy is increased compared with other classes of CVMs.

Postoperative outcomes for patients with heterotaxy are relatively poor. For example, the diagnosis of heterotaxy was associated with increased postoperative mortality among more than 70,000 total cases in the STS database from 1998 to 2009. Mortality risk may be especially elevated in patients with heterotaxy and single-ventricle lesions, but these outcomes may be improving over time. The presence of complex cardiac anatomy may explain some of these observations, including an increased risk for arrhythmia due to anatomic abnormalities of the cardiac conduction system. Frequent findings of asplenia and polysplenia, which are associated with decreased splenic function, increase the risk for postoperative bacterial infections and sepsis ( Table 75.2 ). Long-term antibiotic prophylaxis is indicated in the presence of splenic dysfunction. Recent data indicate that ciliary dysfunction is frequent in heterotaxy and may be a significant contributor to postoperative complications, including prolonged mechanical ventilation and increased need for tracheostomy. Screening for ciliary dysfunction may be useful in patients with single-ventricle lesions or unexpectedly prolonged duration of respiratory support.
Asplenia | Polysplenia |
---|---|
Common atrioventricular canal (85%) with DORV, transposed great arteries, and pulmonic outflow atresia or extreme stenosis | Ventricular septal defect with pulmonic stenosis, normal great arteries or DORV without subaortic conus |
IVC intact: frequent anomalous drainage of hepatic vein | IVC interruption with azygos drainage |
SVC bilateral, unroofed coronary sinus | SVC may be bilateral |
Pulmonary veins often drain into systemic vein, obstructed | Pulmonary venous drainage divided(RPV to RA, LPV to LA) |
Lungs: bilateral trilobed | Lungs: bilateral bilobed |
Liver: symmetric | Liver: symmetric or normally lobulated, risk of extrahepatic biliary atresia |
Viscera: heterotaxy with risk of obstruction due to malrotation | Viscera: heterotaxy with risk obstruction due to malrotation |
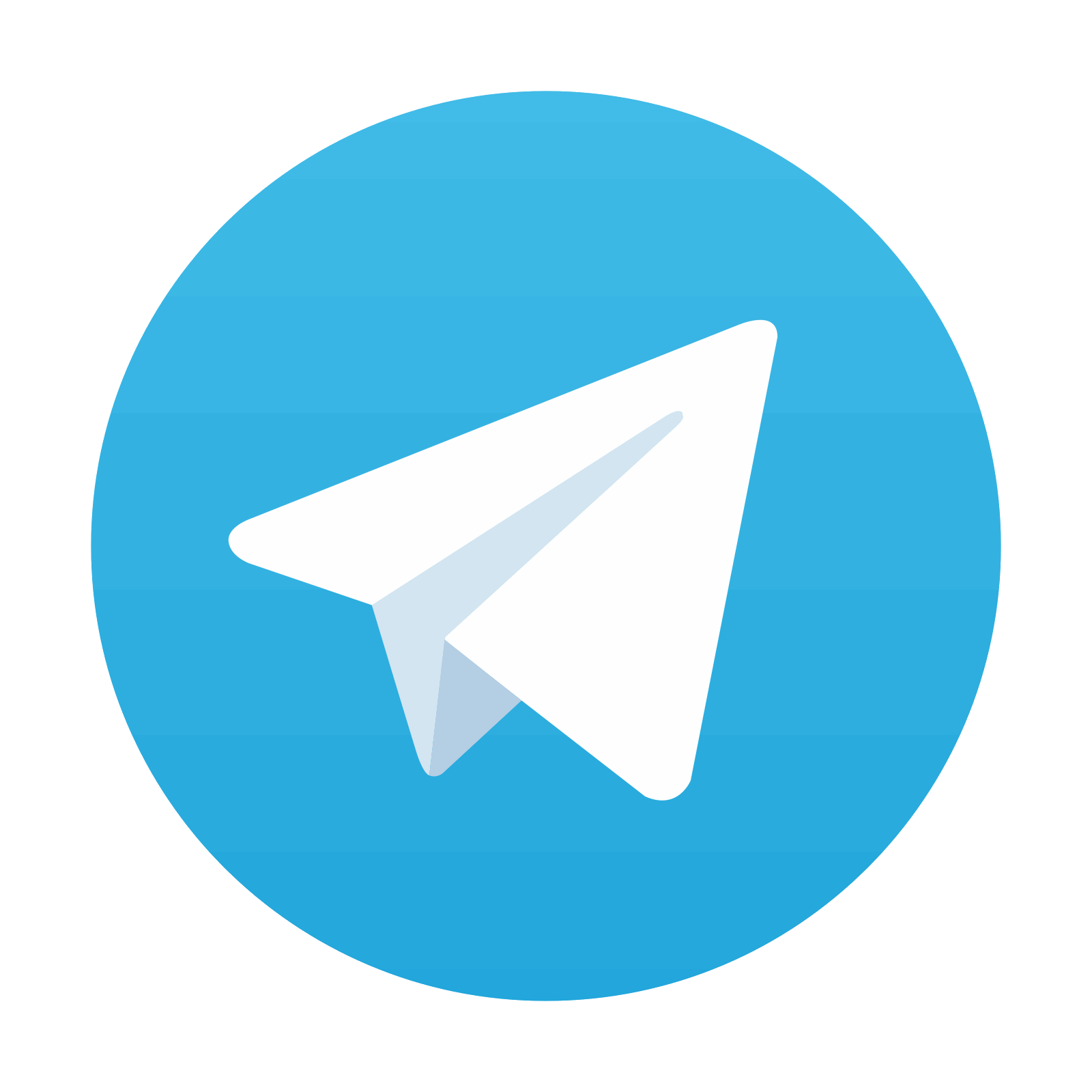
Stay updated, free articles. Join our Telegram channel

Full access? Get Clinical Tree
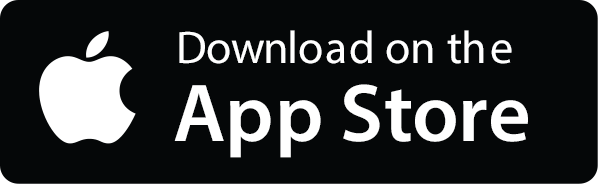
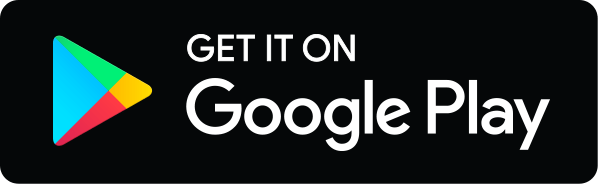
