Pericardial effusion
With or without cardiac tamponade including RV compression. Tamponade: respirophasic flow changes; poor RVOT SV
LV failure secondary to partial LV unloading
(by serial exam comparison)
(a) 2D/3D: increasing LV size by linear or volume measurements; increased AV opening duration, increased left atrial volume
(b) Doppler: increased mitral inflow peak E-wave diastolic velocity, increased E/A and E/e′ ratio, decreased deceleration time of mitral E velocity, worsening functional MR, and elevated pulmonary artery systolic pressure
RV failure
(a) 2D: increased RV size, decreased RV systolic function, high RAP (dilated IVC/leftward atrial septal shift), leftward deviation of ventricular septum
(b) Doppler: increased TR severity, reduced RVOT SV, reduced LVAD inflow cannula and/or outflow-graft velocities (i.e., <0.5 m/s with severe failure), inflow-cannula high velocities if associated with a suction event. Note: a “too-high” LVAD pump speed may contribute to RV failure by increasing TR (septal shift) and/or by increasing RV preload
Inadequate LV filling or excessive LV unloading
Small LV dimensions (typically <3 cm and/or marked deviation of interventricular septum toward LV). Note: may be due to RV failure and/or pump speed too high for loading conditions
LVAD suction with induced ventricular ectopy
Underfilled LV and mechanical impact of inflow cannula with LV endocardium, typically septum, resolves with speed turndown
LVAD-related continuous aortic insufficiency
Clinically significant—at least moderate and possibly severe—characterized by an AR proximal jet-to-LVOT height ratio >46% or AR vena contracta ≥3 mm; increased LV size and relatively decreased RVOT SV despite normal/increased inflow-cannula and/or outflow-graft flows
LVAD-related mitral regurgitation
(a) Primary: inflow-cannula interference with mitral apparatus
(b) Secondary: MR functional, related to partial LV unloading/persistent heart failure
Note: Elements of both a and b may be present
Intracardiac thrombus
Including right and left atrial, LV apical, and aortic root thrombus
Inflow-cannula abnormality
(a) 2D/3D: small or crowded inflow zone with or without evidence of localized obstructive muscle trabeculation, adjacent MV apparatus, or thrombus; malpositioned inflow cannula
(b) High-velocity color or spectral Doppler at inflow orifice. Results from malposition, suction event/other inflow obstruction: aliased color-flow Doppler, CW Doppler velocity >1.5 m/s
(c) Low-velocity inflow (markedly reduced peak systolic and nadir diastolic velocities) may indicate internal inflow-cannula thrombosis or more distal obstruction within the system. Doppler flow-velocity profile may appear relatively “continuous” (decreased phasic/pulsatile pattern)
Outflow-graft abnormality
Typically due to obstruction/pump cessation
(a) 2D/3D imaging: visible kink or thrombus (infrequently seen)
(b) Doppler: peak outflow-graft velocity ≥2 m/sa if near obstruction site; however, diminished or absent spectral Doppler signal if sample volume is remote from obstruction location, combined with lack of RVOT SV change and/or expected LV-dimension change with pump speed changes
Hypertensive emergency
New reduced/minimal AV opening relative to baseline exam at normal BP, especially if associated with new/worsened LV dilatation and worsening MR. Note: hypertension may follow an increase in pump speed
Pump malfunction/pump arrest
(a) Reduced inflow-cannula or outflow-graft flow velocities on color and spectral Doppler or with pump arrest shows diastolic flow reversal
(b) Signs of worsening HF, including dilated LV, worsening MR, worsened TR, and/or increased TR velocity; attenuated speed-change responses: decrease or absence of expected changes in LV linear dimension, AV opening duration, and RVOT SV with increased or decreased pump speeds; for HVAD, loss of inflow-cannula Doppler artifact
Role of Echocardiography After LVAD Implantation
The significant variability among individual patients’ clinical courses after LVAD implantation precludes taking a “one-size-fits-all” approach to postimplantation echocardiography. Nevertheless, an overall framework can be recommended to improve patient care while addressing the efficiency of both the LVAD clinic and the echocardiography laboratory. Surveillance echocardiography generally refers to standard transthoracic echocardiography (TTE) imaging that is performed in the echocardiography lab, in the LVAD clinic, or at the bedside.
In LVAD-supported patients, surveillance echocardiography is performed at the pump’s baseline speed setting and includes LVAD-specific views and Doppler flow assessments , in addition to all the elements of a standard TTE exam for heart failure (HF) patients. If an LVAD optimization protocol is added, further limited imaging can be performed at pump speeds above or below the baseline speed to optimize LVAD and native heart function, although standards for optimal pump speeds may vary among centers and according to patient-specific variables.
Patients with an uncomplicated postoperative course (i.e., absence of HF symptoms, successful weaning from intravenous pharmacologic inotropic and vasopressor agents within 14 days, absence of LVAD controller alarms, and no serologic evidence of hemolysis or infection) should undergo follow-up surveillance TTE at prespecified intervals. Periodic LVAD surveillance echo exams are recommended, to establish patient-specific “baseline” parameters for both LVAD and native heart function. An LVAD surveillance echo exam should be considered at approximately 2 weeks after device implantation or before discharge from the index hospitalization (whichever occurs first). Additional routine surveillance TTE should be considered at 1, 3, 6, and 12 months after implantation and every 6–12 months thereafter; however, at this time, no outcome data support a specific timetable. Figure 11.1 summarizes a reasonable sample schedule for timing postimplantation surveillance TTE .


Fig. 11.1
Sample schedule for initial and follow-up surveillance echocardiography of patients with no evidence of device malfunction
Comparing serial surveillance exam results with each other (for an individual patient) or to population-based benchmarks can also help the examiner understand a patient’s response to LVAD therapy over time. Moreover, surveillance data may allow early diagnosis of occult native heart abnormalities (e.g., development of LVAD-related aortic regurgitation [AR]) or device-related problems, including a drift from previously optimal device speed settings. When surveillance TTE is coordinated with the patient’s routine LVAD clinic visits, HF specialists can integrate the information obtained into their clinical assessments and care plans. A putative benefit of routine LVAD surveillance echocardiograms (with optimization protocols when indicated) is better patient outcomes, including early detection and treatment of complications and fewer hospitalizations for recurrent HF.
Key Points
Patients with an uncomplicated postoperative course should undergo LVAD surveillance echocardiography at predetermined intervals after LVAD implantation to assess the patients’ response to mechanical circulatory support (MCS) therapy and to screen for the development of subclinical complications.
When possible, LVAD surveillance echocardiography should be coordinated with routine LVAD clinic visits.
Clinical Data Acquisition Standards and Sonographer Reproducibility
Before initiating any LVAD echo exam, sonographers should always annotate the LVAD type and baseline LVAD speed in rotations per minute (rpm) on the imaging screen (Fig. 11.2), in addition to the standard patient demographic data. If the device speed is changed, this should be noted during the exam. The device type and speed information should also be routinely incorporated into reporting templates.




Fig. 11.2
Side-by-side comparison of multiple imaging metrics in the same patient before and after HM-II LVAD impeller thrombosis . (a) LVIDd , normal LVAD; (b) increased size by LVIDd after LVAD thrombosis; (c) AV M-mode, minimal opening (107 ms) during normal LVAD function; (d) markedly increased AoV opening durationFig. 11.2 (continued) (230 ms) after internal LVAD thrombosis; (e, g) inflow-cannula color-flow (arrow) and pulsed Doppler images, respectively, during normal LVAD function; (f, h) very-low-velocity inflow-cannula systolic flow on color-flow (arrow) and pulsed Doppler images, respectively, with nearly absent diastolic flow (view h) after development of impeller thrombosis; (i) RVOT pulsed Doppler VTI = 15 cm during normal LVAD function; (j) RVOT pulsed Doppler VTI = 7.9 cm after LVAD thrombosis. Inflow inflow cannula; vel. velocity
Blood Pressure
The patient’s blood pressure (BP ) , which reflects peripheral vascular resistance, is an important parameter that greatly influences ventricular unloading and the observed echocardiographic findings. Therefore, BP should be recorded just before the exam and immediately afterward if pump speed changes were made. Patients with a continuous-flow LVAD (CF-LVAD) have a reduced and narrowed pulse pressure and may not have a palpable pulse. Therefore, cuff-based BP assessment may be difficult or impossible. In the intensive care unit, BP may be obtained from invasive arterial monitoring devices. In other circumstances in which no pulse is present, a mean arterial BP can be obtained with a standard BP cuff, along with a handheld audible Doppler device for detecting brachial or radial artery flow [2]. Note that the arterial Doppler-derived BP reading lies between the systolic pressure and the mean arterial BP [3]. For practical purposes, if the patient has a pulse (i.e., the aortic valve [AV] is opening), the Doppler-derived BP is the same as the systolic BP. If the patient does not have a pulse (i.e., the AV is not opening), the Doppler BP is considered to be the mean arterial BP.
A current BP measurement is necessary for accurate echo interpretation and for safety reasons during “speed change” protocols, particularly when changing to higher speed settings. Susceptible patients may develop clinically significant hypertension in response to increased LVAD flow, and a mean arterial pressure of <85 mmHg is recommended [4]. Hypotension is generally defined as a mean arterial pressure of <60 mmHg and may be associated with traditional symptoms or signs of hypoperfusion. With CF-LVADs , one challenge is that a sonographer (or some other trained and available individual) needs to be facile at obtaining an arterial Doppler-derived BP reading. To facilitate the care of CF-LVAD recipients, better BP monitoring techniques may be needed [5].
Key Points
Although BP readings can be challenging to obtain in LVAD patients, this variable is important because it significantly influences echo findings and their interpretation.
In the absence of a palpable pulse, BP measurement may require audible Doppler interrogation by an appropriately trained individual before the echo exam.
Susceptible patients can have marked hypertension after the LVAD pump speed is increased. Therefore, BP measurement should be repeated after a significant pump speed increase, particularly if the patient’s BP is elevated at the baseline pump speed.
A mean arterial BP of <85 mmHg is recommended.
Hypotension is generally defined as a mean arterial pressure <60 mmHg. It may be associated with traditional symptoms or signs of hypoperfusion.
LV Size and Systolic Function
Linear and volumetric approaches for determining LV size and systolic function in non-LVAD patients have been described by Lang and colleagues [6]. These methods may or may not be appropriate for LVAD patients, as outlined below.
LV Size
As mentioned above, the left ventricular internal dimension at end-diastole (LVIDd) from the 2D parasternal long-axis image is considered the most reproducible measure of LV size in LVAD recipients (Fig. 11.2a, b). In patients with a normally functioning CF-LVAD, severely depressed native LV function, and altered mitral valve opening, determining end-diastole may be difficult. In this scenario, correlating the images to the electrocardiographic signal can be helpful. Additionally, using a microbubble contrast agent should be seriously considered when endocardial definition is insufficient for accurate LVIDd measurement [7]. Previous data from HeartMate II (HM-II)-supported outpatients in stable condition suggest that the LVIDd is probably at least 15% lower than the preimplantation value 3 months after implantation [8, 9]. Care must be taken to correlate LV end-systolic versus end-diastolic diameters with the electrocardiographic signal. Paradoxically, the LVIDd may be smaller than the left ventricular internal dimension at end-systole (LVIDs); this is an important finding, as it may indicate excessive LVAD unloading, severe right ventricular (RV) dysfunction, or both.
Left ventricular volumes, as determined by the Simpson’s biplane or single-plane method of disks (Fig. 11.3) , reflect the LV size more accurately than do linear measurements. However, measuring the LV size by volume may be technically challenging after LVAD implantation because of apical shadowing/dropout associated with the inflow cannula. This is one reason why postimplantation LV volumes assessed by echocardiography are smaller than those assessed by cardiac computed tomography [10]. A reasonable LV diastolic volume assessment is possible in many ambulatory LVAD patients, and this metric can be incorporated into the surveillance exam, particularly at the baseline pump speed setting. However, LVIDd measurement, being more expediently acquired and reproducible, is practical for tracking the relative LV size over time at a baseline pump speed (e.g., Fig. 11.2a, b vs. Fig. 11.3) and in the context of a speed-change exam (see below) for quick problem solving. That the serial LVIDd measurement (combined with the degree of AV opening) can be used as a surrogate marker for the degree of LV unloading in CF-LVAD patients seems intuitive and is supported by the limited available literature, which is derived primarily from HM-II studies. However, robust outcome data are limited, and applicability to patients with a HeartWare LVAD (HVAD) , from whom there is less evidence, has not been demonstrated at this time [11].


Fig. 11.3
Left ventricular end-diastolic volume (LVEDV) , as measured by Simpson’s biplane method of disks . This method is preferred for LV size assessment when possible. Simpson’s single-plane LVEDV method (using the best-/least-foreshortened (a) four-chamber [4Ch] or (b) two-chamber [2Ch] view) may suffice for LV size assessment and may be superior to linear measurements (e.g., Fig. 11.2). The inflow cannula (arrow) and anterolateral papillary muscle (Asterisk) are excluded from the endocardial tracing. Note: In view b, aneurysmal remodeling of the LV apex (relative to the LV base), which would cause underestimation of LV size by parasternal long-axis-view linear measurements (e.g., Fig. 11.2a, b)
LV Systolic Function
Accurately determining LV volumes is challenging after device implantation. So, too, is accurately and meaningfully assessing overall LV systolic function from the patient’s LV ejection fraction (LVEF) . The limitations of LVEF measurement are both technical (with regard to imaging quality) and physiologic. The LV endocardium may be difficult to visualize because of apical foreshortening, apical shadowing from the device, or acoustic dropout (signal attenuation). Physiologic challenges related to the LVAD include enhanced interventricular dependence and discordant septal and inferolateral wall motion, which can vary considerably in the same patient at different pump speeds. If the LV endocardium, including the apex, can be adequately visualized, with or without a microbubble contrast agent, the preferred method for calculating the LVEF is the biplane method of disks (modified Simpson rule; Fig. 11.3) [6]. Although other parameters for LV systolic function can be considered, LVEF is an important surrogate because it can reveal possible LV worsening or recovery. Therefore, surveillance and recovery LVAD exam reports should include an LVEF assessment, even if only a qualitative assessment is possible. However, LVAD support markedly reduces LV afterload, an important determinate of LVEF . Therefore, the value of LVEF for determining systolic function during LVAD support must be taken into consideration during clinical decision-making.
Other methods: In patients with suboptimal apical but adequate parasternal views, the following methods for measuring LV systolic function may be considered, although their accuracy has not been validated in LVAD patients:
- 1.
The LV fractional area change (FAC) method at the mid-papillary muscle level on 2D short-axis views: FAC (%) = [(end-diastolic area − end-systolic area)/(end-diastolic area)] [12].
- 2.
The Quinones method for determining the LVEF [13], with the assumption of an akinetic apex given the presence of the apical inflow cannula.
- 3.
The linear and volume measurements of systolic function noted above represent possible methods of tracking the course of individual patients, serving as their own controls, over time. However, routinely using the three methods described above may not be feasible or advisable for many LVAD patients because of segmental wall motion abnormalities, exaggerated paradoxical septal motion, ventricular dyssynergy, or ventricular septal shift, the extent of which could change at varying pump speeds in the same patient. Note that calculating the LVEF from the LV stroke volume is not recommended, because many LVAD patients have beat-to-beat variations in this parameter [15]. Previous data suggest that most outpatient HM-II recipients in stable condition have persistent moderately to severely depressed LV systolic function during the first 6 months after device implantation [8, 9].
Key Points
After CF-LVAD activation, the LVIDd may be the most reproducible measure of LV unloading that can be tracked over time and at different pump speeds.
The LV end-diastolic volume is a more accurate representation of LV size than is the LVIDd.
After LVAD implantation, measuring LV volumes and the LVEF can be technically challenging. When the LVEF needs to be obtained (particularly to assess for LV recovery), the Simpson biplane method of disks is recommended for use when possible.
LV Diastolic Function
It can be assumed that LVAD patients have markedly abnormal baseline diastolic function. Although the standard LV diastolic function parameters [16] can (and, in the context of clinical research, should) be measured and included in the report, there is a paucity of data validating their clinical usefulness in patients receiving LVAD support. However, the LVAD echo report should not include an assessment of LV diastolic function, which has not been validated in LVAD-supported patients. Using certain acquired diastolic parameters could be helpful, particularly when they are correlated with symptoms in individual patients. This should be done at the discretion of the interpreter, because these parameters may reflect changes in the degree of LV unloading when compared with data recorded during previous examinations or at different pump speeds during the same exam.
Previous data suggest that the mitral E velocity (cm/s), left atrial volume (mL), pulmonary vascular resistance (Wood units), and pulmonary artery systolic pressure (mmHg) are significantly reduced and that the mitral deceleration time (ms) is significantly prolonged in outpatients whose condition is stable 3–6 months after HM-II implantation [8, 9]. How these parameters should be integrated into postimplantation clinical management is currently unclear, as is their prognostic value for patient outcomes. For clinical LVAD echo reporting purposes, a practical approach at this time may be to use the following (or a similar) statement: “Interpretation of the degree of LV diastolic dysfunction (presumed abnormal) is not provided because of continuous-flow LVAD support.”
Key Points
It can be assumed that LVAD patients have markedly abnormal baseline diastolic function.
How LV diastolic parameters should be integrated into the interpretation and report of an LVAD echocardiography examination and any resulting treatment decisions is currently undefined, as is these parameters’ prognostic value for patient outcomes.
RV Size and Systolic Function
Many of the standard measures of RV size and systolic function [17], including linear dimensions, RV FAC , tricuspid annular plane systolic excursion (TAPSE) , and right-sided cardiac output, can feasibly be measured in LVAD patients [8, 18, 19]. However, recent data suggest that the correlation of TAPSE with overall RV systolic function may be weaker after cardiothoracic surgery, so this variable may have less clinical utility than the other measures [20]. Current data regarding the expected response of RV systolic function after LVAD implantation are conflicting: one study showed a significant improvement in RV FAC at 3 months [9], but another study did not show a significant difference in this parameter at either 1 or 6 months [8].
Valvular Assessment
Aortic Valve
Evaluating and reporting the degree of AV opening (if any) are important because it is affected by several other parameters, including LVAD speed, native LV function, volume status, and peripheral vascular resistance. In addition, whether the AV opens may have clinical implications. Whereas recent guidelines recommend that the LVAD speed be set low enough to allow at least intermittent AV opening [21], opening may not occur at any LVAD speed in patients with extremely poor native LV function. The frequency of AV opening is most accurately assessed by recording multiple (5 or 6) cardiac cycles at a slow M-mode sweep speed (e.g., 25–50 mm/s) (Fig. 11.4); the valve should be characterized as opening with every cardiac cycle, opening intermittently, or remaining closed [15, 22].


Fig. 11.4
The duration of AV opening during LVAD support can be easily measured by using M-mode during either TEE (a) or TTE (b). In view a, the AV “barely opens” intermittently (arrows); this maybe related, in part, to an arrhythmia and suggests normal LVAD function at a pump speed of 9600 rpm. In view b, there is near-normal AV opening, with durations of >200 ms; this may be an abnormal finding at a high LVAD pump speed (9800 rpm). (c–e) The expected progressively reduced duration of AV opening in the same patient during a ramp (speed-change) echo exam at different HM-II pump speeds: In view c (8000 rpm), the AV “barely opens,” in view d (8600 rpm), the AV “opens intermittently” (arrows); in view e (9000 rpm), the AV “remains closed”
Many HF teams also request that the duration of AV opening be measured (in ms) from the same M-mode acquisitions. This parameter may vary from beat to beat, so it is best to measure several beats and report an average value. When the AV opening duration is relatively constant, a faster sweep speed (e.g., 75–100 mm/s) may be appropriate (Fig. 11.4). An important potential pitfall of using M-mode to assess the presence and duration of aortic cusp separation is illustrated in Fig. 11.5. In this example, AV semilunar cusp conformation, combined with cardiac translational motion or slightly off-axis imaging, can create the false appearance of aortic cusp separation when the cusps are not actually separating. Careful attention and the additional use of color M-mode may be useful in difficult cases to avoid M-mode “pseudo AV opening ” or an exaggerated AV opening duration. However, in some cases of “minimal” AV opening, the duration of AV cusp separation and the duration of forward systolic flow are not always the same, and using color M-mode can help to document this finding (Fig. 11.6).



Fig. 11.5
Images suggestive of an exaggerated or “false” AV opening duration, as assessed by M-mode. This artifact should be suspected when the aortic cusp opening shape is fusiform (a). Although the apparent M-mode AV opening duration in this case appeared to be >200 ms (arrows), there was, in fact, little or no AV opening. (b) This error was due to several factors, including the semilunar shape of the AV cusps, placement of the interrogating cursor to the left of the cusp closure line (view b: red line), and translational motion of the aortic root. This pitfall could have negative implications when the examiner relies solely on M-mode for selecting the AV closing speed during an LVAD optimization protocol. M-mode should not be used in isolation. False M-mode AV opening can be identified by correlating M-mode findings with the 2D image and color M-mode (in the presence of AR) to validate the extent of AV opening

Fig. 11.6
Assessment of AR . (a) TEE shows at least moderate—and possibly severe—continuous AR during LVAD support. The AR vena contracta (VC) is clearly >3 mm, and the jet width/LVOT width is clearly >46%. Color-flow Doppler reveals inflow-cannula systolic entrainment of the AR jet (arrow). A closed MV and trace MR (Asterisk) areFig. 11.6 (continued) indicative of marked systolic AR. RVOT, right ventricular outflow tract. (b, c) During LVAD support, at least moderate continuous AR (arrow) is observed in the transthoracic parasternal long-axis view with color Doppler (b) and color M-mode imaging (c); the inflow cannula is denoted by an asterisk. In view c, note the variance in the early systolic (arrowhead) versus late systolic (arrow) AR VC width, as shown by M-mode. This finding is not consistent among different patients; it is likely influenced by several variables and by the fact that the AV cusps can exhibit augmented systolic opening, despite AR, at speeds close to (but less than) the AV “opening speed.” (d–e) The AR VC width may increase at higher pump speeds in the same patient, as seen here. This may partially be due to an increased systemic arterial pressure at higher pump speeds, which presumably increases the AR volume. At both speeds, the VC is >3 mm, indicating at least moderate—and possibly severe—AR. The VC width is 4.2 mm at 8600 rpm in view d and is 5.7 cm at 9600 rpm in view E (HM-II LVAD). (f) “Continuous” holosystolic and holodiastolic AR, as detected by continuous-wave Doppler (TTE apical 5-chamber view). (g) Continuous-wave Doppler (TTE apical 5-chamber view) reveals nearly continuous AR, which significantly extends into the electrical and mechanical systolic period with a brief period of AV systolic forward flow (arrows). (h) Color M-mode shows minimal AV opening, with a brief duration of low-velocity systolic forward flow (arrows). (i) TTE parasternal long-axis view of an AR jet on color-flow Doppler imaging (arrow). (j) The AV opens widely, with forward flow that interrupts AR. However, the AR period extends into the electrical and mechanical systolic period (arrows) during HVAD pumping at 2600 rpm
In patients whose AV remains closed, it is important to evaluate for aortic root thrombus, which may be transient or associated with commissural fusion. Continuously closed aortic cusps have been associated with the development of aortic root thrombosis and LVAD-associated AR [23], as discussed below. Fusion of the aortic cusps , either surgical or secondary to chronic aortic cusp closure, can be recognized on speed-change echocardiograms (discussed below).
New-onset (“de novo”) AR is found in approximately 25–33% of patients 12 months after LVAD implantation [24, 25] and is a key finding, given its adverse effects on LVAD performance and its association with morbidity and mortality [26–28]. Several studies suggest that persistent AV closure is a risk factor for de novo AR after LVAD implantation, even when no aortic root thrombus is present (Fig. 11.7) [24, 29, 30]. Standard methods for quantifying AR [31] may be challenging to use after LVAD implantation. In the absence of definitive cutoff criteria to define mild, moderate, and severe AR after LVAD implantation, one should perform an aggregate assessment based on duration (predominantly diastolic vs. continuous AR flow by spectral Doppler), AR jet vena contracta width (significant AR vena contracta ≥3 mm, see Table 11.1), the presence or absence of holodiastolic flow reversal in the descending thoracic aorta on suprasternal notch views, jet height relative to the LV outflow tract (LVOT) , comparative LVAD and native-circuit flow measures (Figs. 11.8 and 11.9) , and LV chamber size. Additionally, significant AR noted on LVAD surveillance echocardiography can be further evaluated with device controller data and the cardiac response during LVAD problem-focused echocardiography with speed changes, as described below.



Key Points
Recording multiple cardiac cycles with color M-mode at a sweep speed of 25–50 mm/s is recommended to accurately assess the frequency and duration of AV opening.
Persistent AV closure can be associated with aortic root thrombus and de novo AR.
If aortic root thrombus is suspected, decreasing the LVAD pump speed (e.g., during a planned speed-change exam) should be avoided, because it could result in sudden AV opening.
After LVAD implantation, AR is not uncommon. Assessment of AR severity is partly based on careful color Doppler analysis in the parasternal long-axis view.

Fig. 11.7
De novo AR after LVAD implantation . This condition progressed from no AR on the baseline surveillance study exam at 1 week (a) to trivial AR (arrow) at 1 month (b) to at least moderate AR (arrows, VC >3 mm) at 14 months (c). All images are transthoracic parasternal long-axis views with color Doppler. In this patient, the AV never opened at any pump speed during the LVAD support period; aortic root thrombus was not present

Fig. 11.8
Direct Doppler measurement of LVAD flow from the distal outflow graft, as evaluated by TTE (a). Flow (stroke volume and cardiac output) within the outflow graft (LVAD output) can be derived by measuring the graft’s diameter (arrow) and the pulsed Doppler VTI at the same location, proximal to the anastomosis site (b)

Fig. 11.9
The total cardiac output (combined LVAD flow output and native LVOT flow output [if any]) is the same as the RVOT cardiac output. The RVOT cardiac output is measured by using standard imaging techniques including (a) measurement of the RVOT (pulmonary annulus) diameter (d). Color-flow (b) and spectral Doppler (c) studies are performed to rule out significant pulmonary regurgitation and to measure the RVOT VTI. Note: In the case shown above, the RVOT VTI is low (7–9 cm) at a relatively high HM-II pump speed of 9600 rpm; this was consistent with a low cardiac output, which was due to an obstructed (kinked) outflow graft. It may be useful to average 3–5 VTIs, depending on their variability
Mitral Valve
As noted above, LV unloading generally leads to reduced mitral valve annular dilatation, improved leaflet coaptation, and, ultimately, reduced mitral regurgitation (MR) severity . Persistence of significant MR after LVAD support begins may indicate inadequate LV unloading or inflow-cannula malposition and interference with the submitral apparatus. If MR is present, it can be quantified by standard methods [32]. Incidental post-LVAD MR may also represent LVAD malfunction and should be discussed with the clinical team .
Tricuspid and Pulmonary Valves
Like MR, moderate or greater tricuspid regurgitation (TR) is an important finding on LVAD surveillance echocardiography, because this condition can be associated with insufficient LV unloading (functional TR), excessive LV unloading with a leftward shift of the interventricular septum (e.g., a suction event), elevated systolic pulmonary pressures, or intrinsic RV systolic dysfunction. Distinguishing among these causes by using echocardiographic parameters is discussed in further detail below. Regardless of the cause, TR after LVAD implantation can generally be assessed with standard methods [32]. Furthermore, the native pulmonary valve typically remains functionally normal after LVAD implantation and can be interrogated by using standard methods when significant stenosis or regurgitation is suspected [31, 32].
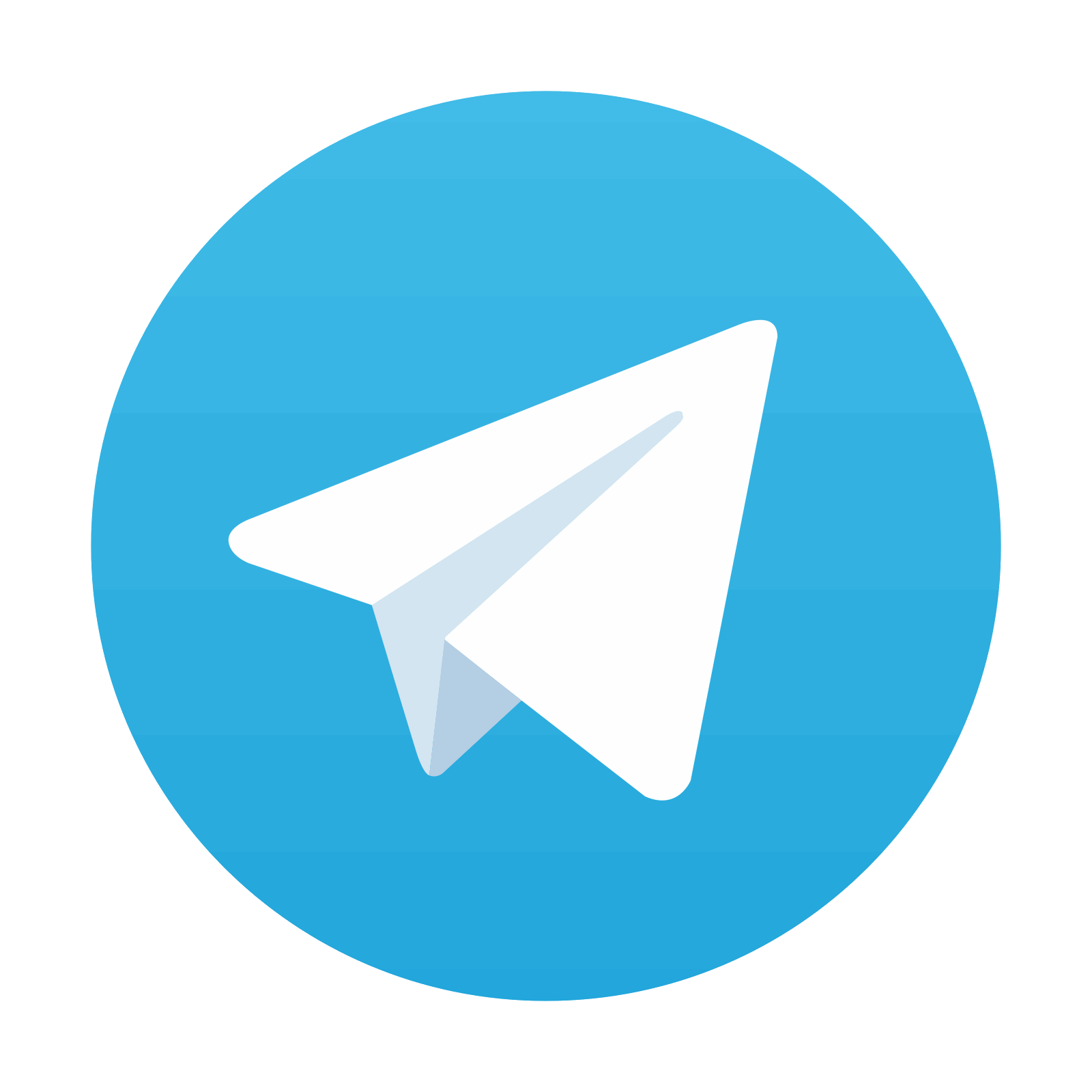
Stay updated, free articles. Join our Telegram channel

Full access? Get Clinical Tree
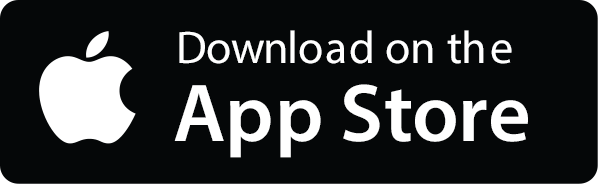
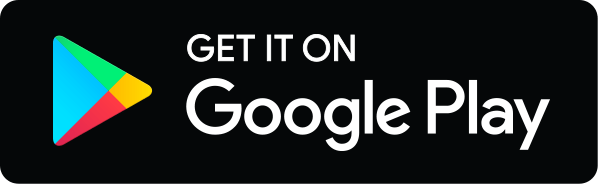