Empyema and bronchopleural fistula (BPF) are two distinct yet intimately related entities. They may occur together or independently, and they share similar etiologies. The management of each process has proved, over several centuries, to be a daunting task that requires sound clinical judgment and a resilient patient.
Hippocrates provided the first clinical description of empyema approximately 2400 years ago. In 229 bc he described the clinical presentation and physical examination findings in patients with empyema. Hippocrates is also credited with the first drainage procedure for empyema. This entailed partial rib resection, drainage, and daily packing.1 Despite Hippocrates’ detailing of the clinical presentation, natural history, and treatment of empyema, it was not until the nineteenth century that significant work on the subject was presented.
In 1843, Trosseau advanced thoracentesis for the treatment of empyema. French surgeon Sedillot described thoracotomy and empyema drainage. More extensive procedures, including thoracoplasty and decortication, were introduced by Estlander (1879) and Fowler (1893), respectively.2 At the start of the twentieth century, most treatment strategies for acute empyema involved early rib resection and open drainage. Mortality rates with this approach averaged 30%. Graham and Bell, of the United States Army Empyema Commission, made a major advance in the treatment of early empyema. They recommended closed-tube drainage to manage early empyema. This strategy decreased the mortality rate from 30% to 4.3%.3
In 1935, Eloesser4 described an open thoracotomy technique that would permit skin and soft tissue to behave as a valve to allow lung expansion. In 1963, Clagett and Geraci5 introduced open window drainage for 6 to 8 weeks, followed by empyema cavity obliteration with antibiotic solution and window closure. The Clagett window remains useful in the treatment of chronic empyema. Muscle flap closure of BPF and the postresectional space has become increasingly popular.6,7 Video-assisted thoracoscopy and fibrinolytic therapy also have roles in the management of empyema.8,9
Empyema is defined as a purulent pleural collection (Fig. 107-1). Causes include bacterial pneumonia, tuberculosis, postresectional, posttraumatic, and intra-abdominal processes. Approximately 50% of empyemas are caused by bacterial infection. Postresectional causes account for 25%, and an additional 8% to 11% are caused by extension of an intra-abdominal process.10
The presenting signs and symptoms of empyema are nonspecific. The most common symptoms are shortness of breath and fever. Patients also may complain of cough and chest pain. Sputum production may or may not be present. These symptoms are also present in patients with pneumonia. Empyema should be considered when a patient manifests these symptoms after a prolonged respiratory illness or a lung resection. Laboratory analysis is also relatively nonspecific and usually includes leukocytosis. C-reactive protein levels that exceed 100 mg/L may be useful as a diagnostic indicator of postpneumonectomy empyema, as described by Icard and colleagues.11 Physical examination findings may be underwhelming, although in empyema necessitatis an undrained empyema may track through the soft tissues, causing cellulitis in the overlying skin.
The radiographic workup of postpneumonic empyema should begin with a posteroanterior and lateral chest x-ray (Fig. 107-2). Hsu and colleagues12 showed that an empyema appears as a wide air–fluid level on posteroanterior view and has a narrow anteroposterior width on lateral projection. Bilateral decubitus films may provide information regarding a freely flowing or loculated empyema. Radiographs also may differentiate among empyema, BPF, and lung abscess. In BPF, the air–fluid level is most commonly located in the posterior costophrenic sulcus.13 There is little difference in air–fluid level size between posteroanterior and lateral projections in the case of lung abscess. Schachter and colleagues14 presented the following qualities that distinguish empyema from lung abscess on plain film: (1) The air–fluid level extends to the chest wall; (2) its border tapers near the mediastinum or chest wall; and (3) the air–fluid level crosses the fissure.
Ultrasound also may be used to evaluate empyemic spaces. Major advantages include portability and identification of loculations or pleural fibrosis. This modality also may differentiate transudates from exudates.15,16 Ultrasound also may guide catheter-based drainage of effusions, although a major limitation is the operator-dependent nature of this modality.
Chest CT scan is the mainstay in the diagnosis and management of empyema and BPF. CT characteristics specific to empyema include a thin, uniform, smooth wall along the exterior surfaces, in contrast with the irregular walls seen in lung abscesses (Fig. 107-3). The “split pleura” sign, which distinguishes the separated visceral and parietal pleural surfaces, can be seen in nearly 70% of empyemas (Fig. 107-4).17 The presence of empyema fluid separates the two hypervascular surfaces, which are readily identified on contrast-enhanced scans. One major pitfall is the difficulty of differentiating atelectasis or effusion from the diaphragm. Intra-abdominal ascites and subdiaphragmatic abscess also may make proper diagnosis of empyema challenging.
MRI may be applied to the diagnosis of parapneumonic effusions and empyema. The multiplanar imagery provides details specific to the location and relationship of the empyema to pleural structures. In addition, the pleural surfaces can be visualized adequately to identify a split-pleura sign. Even so, given the availability and current use of CT, as well as the cost of MRI, widespread use of MRI is limited as applied to empyema diagnosis.
As mentioned earlier, parapneumonic effusions account for the majority of empyemas. The approach should include plain films. Antibiotic therapy is the treatment of choice if the fluid thickness on decubitus studies is less than 1 cm.18 Diagnostic and therapeutic thoracentesis is indicated for larger collections and those that persist or enlarge despite medical therapy.
The evolution from parapneumonic effusion to empyema involves three stages. Stage 1, the exudative stage, is characterized by freely flowing fluid. At this point, the pleural surfaces are inflamed and quite permeable. This stage corresponds to the uncomplicated parapneumonic effusion described by Light and colleagues.18 Stage 2, the fibrinopurulent stage, is characterized by bacterial infection and fibrin deposition. The fluid color may progress from clear yellow to purulent. Biochemical fluid analysis can guide management of these fluid collections. A pleural fluid pH of less than 7.00, pleural fluid glucose concentration less than 40 mg/dL, or a positive culture suggests a complicated parapneumonic effusion, and tube thoracostomy is indicated. Frank pus is an indication for tube thoracostomy. The organized phase, stage 3, occurs approximately 1 week after the initial infection. Fibroblastic ingrowth and collagen deposition occur. Progression of this phase over a period of 3 to 4 weeks may result in a thickened membrane, or “peel,” that results in trapped lung and potentially restricts pulmonary function (Fig. 107-5).
After chest tube placement and drainage, the patient should undergo surveillance chest x-ray and chest CT scan to assess (1) completeness of drainage and (2) lung expansion. If multiple loculations are present on the chest CT scan, intrapleural fibrinolytics or thoracoscopy should be performed. Daily intrapleural streptokinase 250,000 units/100 mL of saline may be administered via the chest tube, followed by chest tube clamping for 4 hours. This therapy can be discontinued when the volume of drainage is low or after lung re-expansion has been demonstrated.9 Fibrinolytic therapy is most applicable to the fibrinopurulent phase of parapneumonic effusions. Despite the reported success of fibrinolytic therapy, we favor video-assisted thoracic surgery (VATS) over lytic therapy. Thoracoscopy permits adhesiolysis and chest tube positioning under direct vision. In addition, the coagulum may be thoroughly removed, and if necessary, a limited decortication can be performed. The overall condition of the lung also may be assessed and the need for thoracotomy and complete decortication determined.8 During the fibrinopurulent stage of empyema, VATS offers decreased hospital stay, reduced cost, and improved cosmesis in comparison with thoracotomy.19
In the organized phase, a thick fibrous “peel” overlies the visceral pleura. Patients may have a restrictive pattern on pulmonary function testing. Findings suggestive of this phase include the persistence of an empyema cavity after 7 to 10 days of chest tube drainage, failure of full lung expansion on radiographs, and a thick “peel” on chest CT scan or at thoracoscopy.
In cases of empyema complicated by trapped lung, thoracotomy and decortication are indicated. Preoperative workup should include a chest CT scan and pulmonary function testing. Ventilation/perfusion lung scanning may be useful in selected patients when poor ipsilateral lung function may indicate the need for pneumonectomy.20 Contraindications to this procedure include a debilitated, moribund patient, adequate drainage and expansion by lesser procedures, severe cardiopulmonary disease, and little or no perfusion to the affected lung.
The technique of decortication requires general anesthesia, usually through a double-lumen endobronchial tube. Packed red blood cells should be available perioperatively. The patient is placed in a full lateral decubitus position, and a serratus-sparing posterolateral or vertical axillary thoracotomy incision is made. The pleural cavity is entered through the fifth or sixth interspace after removing a portion of the overlying rib. The peel is incised initially with a no. 15 scalpel blade (Fig. 107-6). The peel is grasped, and a meticulous excision is performed using a combination of blunt and sharp separation of the peel and underlying visceral pleura. Some degree of ventilation to the affected lung will assist with identifying the proper dissection plane. The presence of air leaks indicates an incorrect dissection level. As the fibrinous covering is removed, the underlying lung will readily expand when ventilated. The dissection should be thorough and extend into the fissure and diaphragmatic lung surfaces. This extremely tedious procedure requires patience on the part of the surgeon and anesthesiologist.
Postresectional empyema may occur after pneumonectomy and lesser resections, including wedge biopsy, segmentectomy, and lobectomy. The risk of occurrence is low at 0.01% for wedge resections and up to 2% for lobectomies.21 Postpneumonectomy empyema is more common, with an incidence of 5% after standard pneumonectomy.22 The mortality rate is as high as 50%.23 Empyema from lesser resections usually occurs secondary to persistent parenchymal leak, with a resulting residual space that becomes secondarily infected. Chest tube drainage and directed antibiotic therapy usually are effective management. More extensive procedures, including VATS with drainage, modified Eloesser drainage, and myoplasty, may be needed in refractory cases. Airspace location may determine the muscle type used during myoplasty. Apical airspaces may be obliterated using the pectoralis major, serratus anterior, or latissimus dorsi muscle. The serratus and latissimus also may be used for posterior spaces. The omentum may be mobilized and passed along the anterior diaphragm for inferior spaces.21
Postpneumonectomy empyema (PPE) represents a serious complication that may occur early or late after pneumonectomy. PPE is accompanied by BPF in up to 80% of cases. Several risk factors that cause BPF may ultimately lead to PPE, as shown in Table 107-1. Preventive measures should include aggressive preoperative treatment of pleural sepsis with antibiotics and optimization of nutritional status. Perioperative measures include careful dissection around the bronchial stump with preservation of bronchial blood supply, appropriate stump length, minimization of intraoperative spillage, and liberal use of autologous flaps for stump coverage. Right pneumonectomy is associated with a higher rate of stump dehiscence, empyema, and BPF. This is likely because the stump is exposed to the pleural space on the right. The left bronchial stump usually retracts medially and subaortically, providing some degree of protection.
Preoperative risk factors Radiation and/or chemotherapy Immunocompromised host Systemic steroids Diabetes Inflammatory diseased lung/destroyed lung |
Intraoperative factors Surgical inexperience Pneumonectomy Long stump Right-sided resections Right pneumonectomy Devascularization of stump Residual cancer at stump Failure of recognition of BPF before closure Tension on bronchial closure |
Postoperative factors Prolonged ventilation Systemic steroids Reintubation |
PPE may be insidious in onset. Symptoms of fatigue, cough, weight loss, and pain may be present. Radiographs may be helpful in cases of PPE with concomitant BPF. A decrease in the air–fluid level on plain film in a patient with the preceding complaints and risk factors should prompt further investigation.
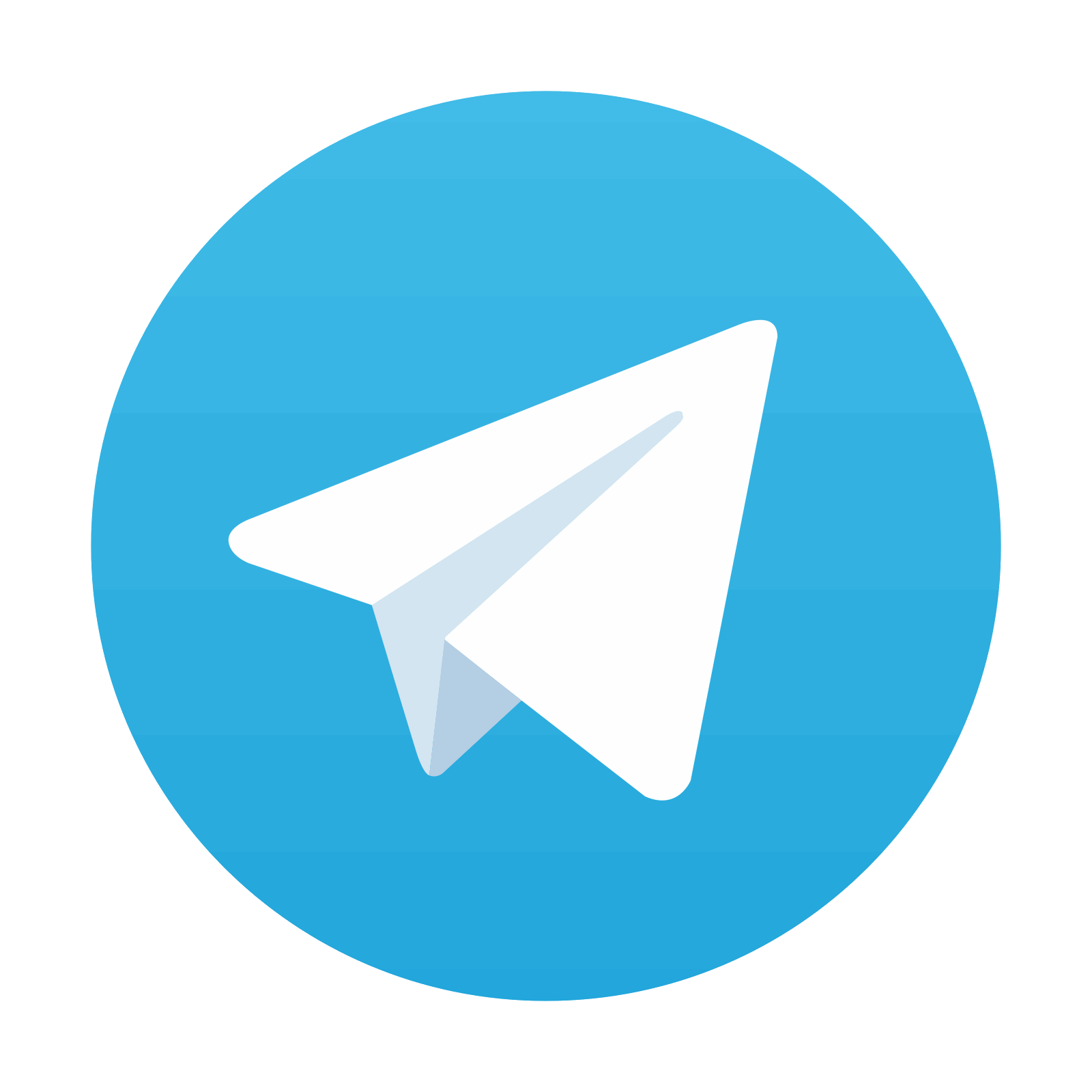
Stay updated, free articles. Join our Telegram channel

Full access? Get Clinical Tree
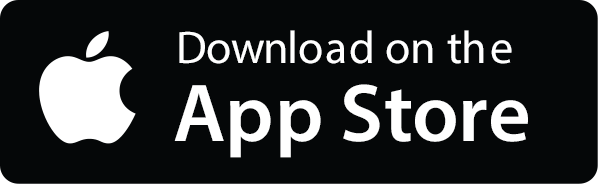
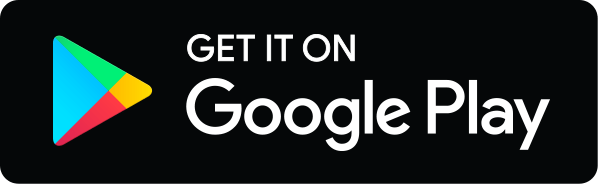