(1)
Project-team INRIA-UPMC-CNRS REO Laboratoire Jacques-Louis Lions, CNRS UMR 7598, Université Pierre et Marie Curie, Place Jussieu 4, 75252 Paris Cedex 05, France
Abstract
Surfactant is an interfacial material of respiratory conduits and its annexes. It modulates the surface tension and innate immune defense of the lung. The alveolar surface film stretches as the lung expands, raising the surface tension, then molecules are packed as the lung deflates, lowering the surface tension.
“ …; omne oleo tranquillari, et ob id urinantes ore spargere, quoniam mitiget naturam asperam lucemque deportet;… […; the whole sea is made still with oil and therefore the divers under the water scatter it with their mouths, because it allaies the rough nature thereof, and carries a light with it;…] ”
(Caius Plinius Secundus [Pliny the Elder; 23–79], Naturalis Historiae, Book II)
Surfactant is an interfacial material of respiratory conduits and its annexes. It modulates the surface tension and innate immune defense of the lung. The alveolar surface film stretches as the lung expands, raising the surface tension, then molecules are packed as the lung deflates, lowering the surface tension.
The substances that lower the surface tension in small amounts are called surface-active agents or surfactants. They reduce the energy required for the surface production.
13.1 Surface Tension
The free surface of a liquid at rest is not necessarily flat and horizontal, especially in the neighborhood of a vertical solid wall. The surface effects are indeed not always negligible with respect to the surface (pressure) and body (gravity) forces. The free surface of a liquid behaves like an elastic membrane bearing a uniform force per unit length.
The surface tension is the force acting on the surface of a liquid that tends to minimize the surface area.1 The smaller the surface area of any interface between a liquid and another medium, the greater the stability of the interface, and the lower the energy involved in the formation of the interface.
Surface tension is the manifestation of intermolecular forces in the interface, the surface shrinking to the smallest possible area. The surface tension is the force of attraction between adjacent molecules of a liquid. Therefore, this molecular cohesive force in the surface film tends to contract the surface.
The pressure–volume curves obtained in air-filled lungs and after filling lungs with saline show that: (1) surface tension forces are greater during inflation than deflation and (2) a large portion of transpulmonary pressure can be attributed to surface tension (Fig. 13.1).2
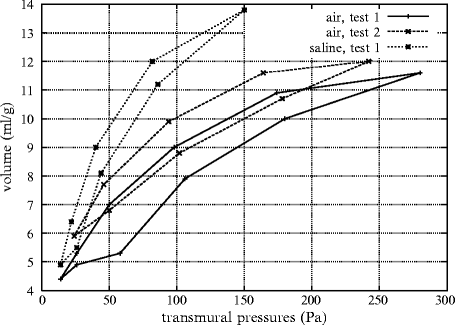
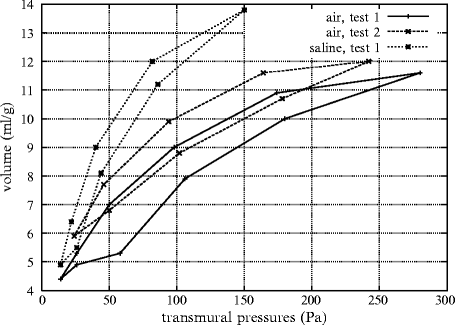
Fig. 13.1
Relationships between volume (ml per g of tissue) and pressure (Pa) in air-filled and saline-filled lungs (Source: [1595]). In the case of air-filled lung, the effect of loading history is demonstrated with the upper shift of the loop during the second cyclic loading with respect to the first. When the lung is filled with saline, only the first cyclic loading is displayed, owing to a quasi-superimposition of the loops of the following successive cyclic loadings with the first. The loop is displaced leftward and upward with respect to curves in air-filled lung.
The superficial tension changes with the radius of curvature of the alveolar surface. The Laplace law states that the transmural pressure increases as the curvature radius decreases, when the surface tension is constant.3 In a bialveolar model with 2 communicating alveoli of different curvature radii, the pressure of the small alveolus is higher than the pressure of the large one. Such a bialveolar model is not synchronized. The force exerted on a very thin curved shell is equal to twice the tension divided by the surface curvature radius. For a surface tension of 5 N/m2 (physiological magnitude) and a radius of 50 μm, the force is equal to 200 kPa. The superficial tension is then minimized in proportion to the alveolar volume by the secretion of a wetting agent.
The interface forces govern adhesion, friction, wettability (wetting or non-wetting)4 of solids by liquids (Fig. 13.2), capillarity of liquids in small-bore tubes5 and the curvature of gas–liquid or solid–liquid interfaces.
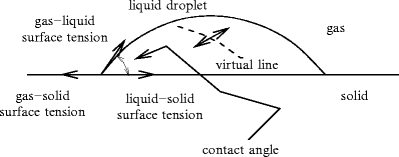
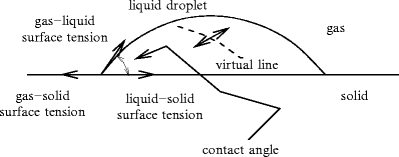
Fig. 13.2
Forces acting on a liquid droplet in a gas wetting a solid. Small droplets (size of
[1 mm]) are spherical whereas larger ones are ellipsoidal under the effect of gravity.

In the liquid domain, the time and space-averaged attraction force (resultant) exerted on any liquid molecule by its neighbors is equal to zero, even though the molecules diffuse and undergo random collisions. But at the interface of the liquid with the surrounding medium, no molecule can balance the effects of the interior liquid molecules (symmetry rupture). Cohesion forces are hence responsible for surface phenomena.6 The surface molecules then bear a net attraction toward the liquid body. The centrally directed forces minimize both the free energy7 and the surface area. The surface tension expresses cohesion internal forces that hinder the liquid molecules from occupying the available space.
Two fluids in contact, such as a liquid and its vapor, are separated by an interface. The interface properties differ from those of 2 uniform phases. The interface is modeled by a zero-thickness membrane undergoing an uniform tension. Let us define 2 surface regions separated by a virtual line on the surface (Fig. 13.2). In any point of this line, the length unit ds is subjected to a force from one of the 2 regions, normal to the line and tangent to the surface at the selected point, of magnitude:

where T s is the surface tension (M. T − 2; N/m).8 The surface tension decays when the temperature rises and becomes zero at the critical temperature. A surface energy (W) corresponds to the surface force on the surface area (A): W = T s A.

(13.1)
At the beginning of the nineteenth century (1805–1806), T. Young and P.S. de Laplace found the equation that describes the relationship between the curvature radius of a surface and the pressure. At the interface, a pressure difference exists between the concave and the convex sides of the surface. When a stretched zero-thickness membrane is crossed, an increment of pressure is observed from the convex side to the concave side that is given by the Laplace law. The excess pressure p on the concave side over the convex side is indeed expressed by the principal radii of curvature of the surface R c1 and R c2:


(13.2)
Using this principle, the vapor pressure p is greater for small bubbles in a liquid:

where p 0 is the vapor pressure over a liquid plane surface (infinite curvature radius) and M the molecular weight.

(13.3)
Any hollow bubble is characterized by 2 interfaces, an inner (convex with respect to the liquid domain) between the liquid and the internal gas (curvature radius R i ), and an outer (concave with respect to the liquid domain) between the liquid and the external gas (curvature radius
). The Laplace law (
) applied to both interfaces then leads to:
(Fig. 13.3).
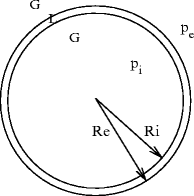
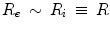

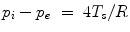
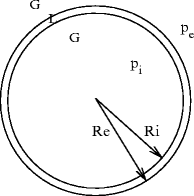
Fig. 13.3
Soap bubble in air.
. A spherical bubble provides the smallest surface containing a given volume. The higher the surface area, the greater the associated energy. The equilibrium is reached when the energy, hence the area, is minimal

In Eustachian tubes, surfactant slightly lowers surface tension and reduces opening pressure for better aeration and middle ear drainage. Eustachian tube surfactant is a much weaker surface tension lowering agent than pulmonary surfactant. Eustachian tube surfactant primarily acts as an anti-adhesive agent.
Alveolus surface tension is associated with neonatal respiratory distress syndrome [1596]. A greater pressure is required to inflate lungs filled with air than with aqueous solution. Surfactant effects were studied in 1950s by various teams of investigators [1597–1601]. The surface tension hampers transudation from capillaries to alveoli through the alveolocapillary membrane. Furthermore, the absence of a lung lining substance can cause neonatal atelectasis [1602]. Surface tension varies with lung compression and expansion, i.e., with changes in the surface area of the lung parenchyma. Surface tension rises with lung expansion to prevent the lung from overexpanding and helps lungs to deflate. Conversely, surface tension falls with lung deflation, maintains the alveoli in an inflation state, and helps them to expand again rather than to collapse. The surfactant, thereby, prevents atelectasis. Surfactant deficiency in respiratory distress syndrome was demonstrated from lung extracts of babies in 1959 [1603]. Surface tension in compressed lung extracts of babies with respiratory distress syndrome remains high. Surface tension increases in expended lung extracts, but to much greater values compared to normal lung extracts.
13.2 Alveolar Surfactant
Alveolar epithelium produces a liquid film over its surface in contact with air, generating a fluid–air interface. Surfactant is made intype-2 alveolar cells. The functional surfactant adsorbs very rapidly into the liquid interface to form a tensoactive monolayer. After secretion, the surfactant causes less densely packed arrangements in the structure of the air–liquid interface.
Surfactant is a material that contains phospholipids, predominantly dipalmitoyl lecithin, and apoproteins. It is included in a fluid layer (thickness ∼ 70 nm). The surfactant reduces surface tension throughout the major part of the lung parenchyma, thereby contributing to lung compliance. It also stabilizes the alveoli.
13.2.1 Formation of the Alveolar Surface Film
Surfactant is formed relatively late in fetal life. In utero, the fetal lung secretes fluid into the alveolar spaces to promote lung development. Shortly before birth, the fetal lung switches from fluid secretion to resorption to clear alveoli of excess fluid and establish a proper air–blood barrier for gas exchange. After birth, gas exchange depends on adequate regulation of the amount of fluid in the very thin liquid layer (thickness ∼ 0.2 mm) that lines the alveolar epithelium. Premature infants born without adequate amounts experience respiratory distress and can die in the absence of clearance of the excess alveolar fluid and restoration of alveolar fluid homeostasis to reinstate normal gas exchange. The half-life of the surfactant is equal to about 18 h.
The formation of the alveolar surface film encompasses 3 successive steps: (1) the transport stage during which surfactant aggregates move from the subphase toward the interface; (2) the attachment stage during which surfactant bilayers associate with the pre-existing monolayer; and (3) the transfer stage during which the structure of the surfactant-associated phospholipid bilayers in the aqueous subphase open in order to enable surfactant transfer to the interfacial monolayer.
Once secreted, surfactant forms tubular myelin, a lung-specific lipid transport molecule for insertion into the air–liquid interface. Tubular myelin, an ordered array of tubules, is formed from secreted lamellar bodies.9 Tubular myelin is a precursor of the interfacial film.
Phase 1 is characterized by unpacking of lamellar bodies, their transformation into tubular myelin, and the transfer of molecules toward the interface. Phase 1 is affected by the local physicochemical conditions (hydration and clcium levels, pH, etc.).
13.2.2 Alveolar Liquid Film Homeostasis
Alveolar epithelium (99% of the lung internal surface area) produces a liquid film and maintains its homeostasis for effective gas exchange. The fluid balance results from osmotic gradients created by active solute transport. In lungs, the alveolar epithelium maintains air spaces free of excess liquid via Na + influx through highly selective, apicalepithelial Na + channels in response to an electrochemical gradient created by basolateralNa + –K + ATPases. The resulting osmotic gradient leads to fluid reabsorption from alveolar to interstitial spaces.
13.2.2.1 Ion Carriers of Type-1 Pneumocyte
Type-1 and -2 alveolar epithelial cells, or pneumocyte-1 and -2, that cover the alveolar wall possess many types of ion channels. Alveolar epithelial type-1 cells (length ∼ 50–100 μm) have epithelial sodium, cyclic nucleotide-gated, and potassium channels, as well ascAMP-stimulated cystic fibrosis transmembrane conductance regulators [1604]. Potassium channels prevent cell depolarization during Cl − efflux and Na + influx. Chloride uptake across CFTR contributes to fluid absorption. In addition, pneumocyte-1 expressesaquaporin-5.
Pneumocyte-1 has the highest known osmotic water permeability of any mammalian cell type. The function of ENaC channel can be modulated by catecholamines. Alveolar type-1 cells indeed possessdopamine type-1 and -2 receptors that can raise ENaC activity [1604]. Moreover, they also containγ-aminobutyric acid receptor, a ligand-gated chloride channel.
Both type-1 and -2 pneumocytes have subunit A1 of Na + –K + ATPase, but only type-1 pneumocyte possesses subunit A2 [1604]. Using this difference with associated distinct antagonist sensitivity, it can be demonstrated that type-1 pneumocyte is responsible for at least 60% of the total alveolar fluid clearance in rats.
13.2.2.2 Ion Carriers of Type-2 Pneumocyte
Alveolar type-2 cells (size ∼ 10 μm) cover 2 to 5% of the alveolar surface area. They produce, secrete, and recycle alveolar surfactant. They also participate in active ion transport, as they have Na + –K + ATPases, K + and epithelial Na + channels, and cystic fibrosis transmembrane conductance regulators [1604]. However, they contain fewer Na + channels than alveolar type-1 cells, at least in rodents.
13.2.2.3 Adenosine Receptors and Ion Transport
Adenosine operates via G-protein-coupled receptors to activate or inhibit adenylate cyclases. Messenger cAMP regulates active Na + transport in alveolar epithelium. Adenosine hence regulates alveolar ion transport via elevated activity of ENaC and CFTR and airspace fluid content (para- and autocrine regulation of the alveolar fluid clearance). At least in rodents, type-1 and -2 alveolar epithelial cells possess all 4 types ofadenosine receptors (A1, A2A , A2B , and A3) [1605]. Receptors A1 and A3 inhibit adenylate cyclase and signal via inositol 3-phosphate and phospholipase-C.
Type-2 adenosine receptor activates adenylate cyclase via Gαs subunit. Receptor A2A is expressed 5- to 9-fold more than the 3 other types in alveolar cells.
At low doses, adenosine increases alveolar fluid clearance owing to A2A and A3 receptors. At physiological doses (micromolar), adenosine reduces alveolar fluid clearance via A1 receptor that causes Cl − efflux through CFTR, which counterbalances active Na + absorption.
13.2.3 Secretion and Role of Alveolar Surfactant
The surfactant that covers the alveolar wetted surface is a phospholipid, mainly lecithin and dipalmitoyl lecithin. Lecithin consists in a long chain of fatty acids bound to glycerol and to electrically charged phosphate and choline groups that are attracted by polar water molecules. Pure oxygen and atmospheric pollutants affect the alveolar surface.
The surfactant production is controlled by the vagus nerve. A decline in surfactant activity indeed results from a cut in vagus nerve. Therefore, the release of surfactant depends on the local blood flow to match ventilation to perfusion.
The surfactant is secreted by the type-2 granular pneumocytes of the alveolar wall. It induces variable surface tension according to the lung volume, i.e., the degree of alveolar expansion. It contributes to the alveolar stability, preserving the alveoli from full collapse during expiration and hyperinflation during inspiration.
Alveoli expand more easily due to the presence of surfactant. This surface-active agent also varies the surface tension with changes in the alveolus surface area. The surfactant layer becomes thinner in inflated alveoli, thereby increasing the surface tension. This augmentation is balanced by the alveolus size elevation. Conversely, rising concentration of surfactant balances the effect of reduction in alveolar size during exhalation.
The surfactant is thus very important because: (1) it promotes alveolar stability, alveoli of varying radii coexisting without ventilation between them, and (2) it reduces thetranspulmonary pressure. Furthermore, the surfactant hinders fluid exudation from the alveolar septa within the alveolar space. The surface tension, the tissue osmotic pressure difference in the alveolar septum, and the capillary blood pressure work to move fluid out of the alveolar capillaries. The capillary osmotic pressure opposes this force combination. A favorable balance can be maintained by a reduction in the surface tension.
The absence of surfactant can induce atelectasis (lung collapse). A reduction in surfactant can occur during prolonged inhalation of 100% oxygen. Premature newborns often have a reduction in or lack of surfactant (respiratory distress syndrome).
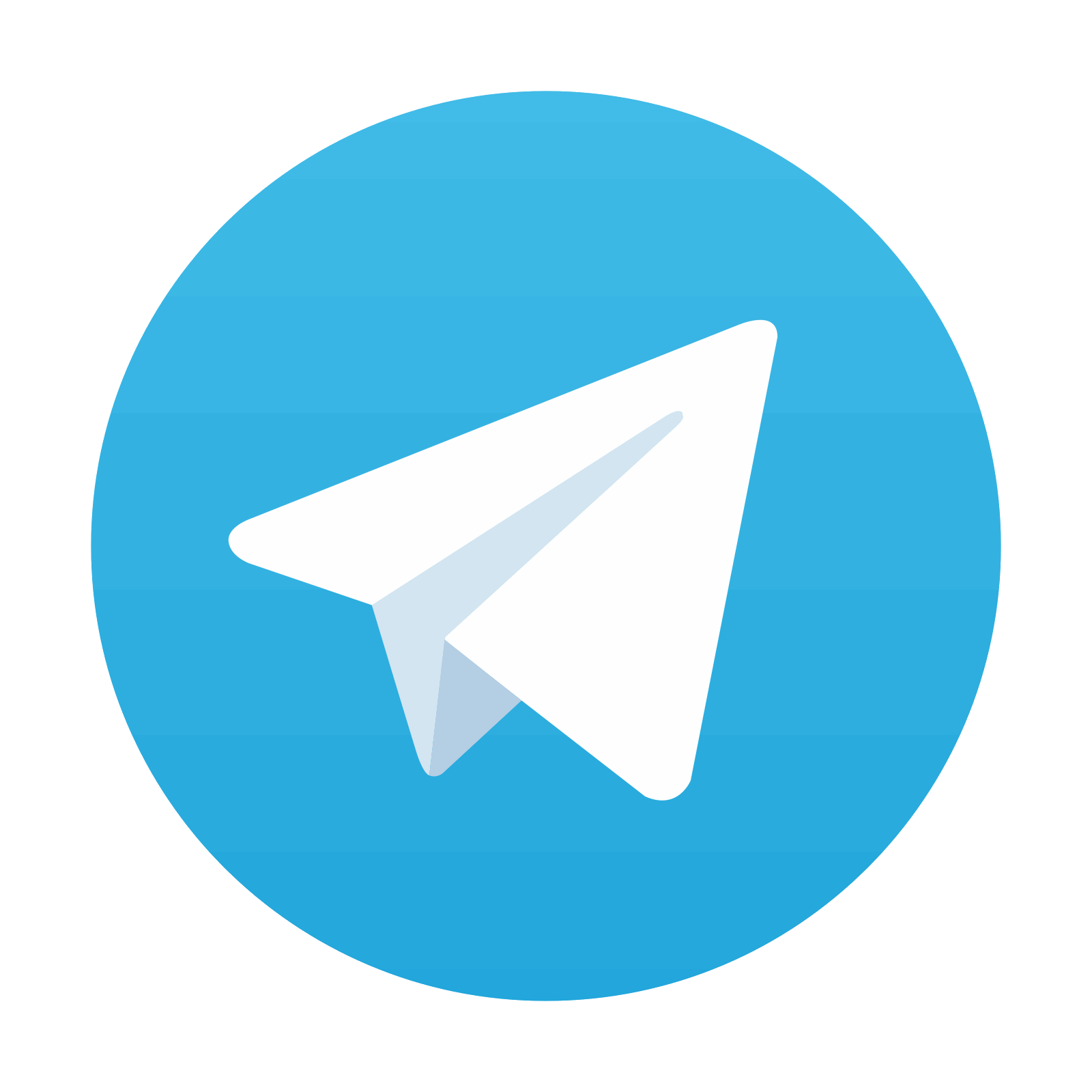
Stay updated, free articles. Join our Telegram channel

Full access? Get Clinical Tree
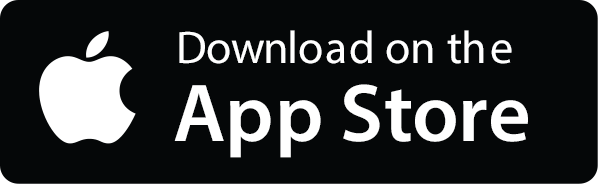
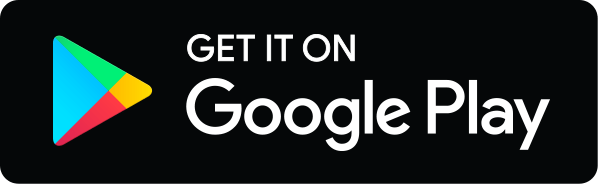