Dysfunction
Clinical example
Devices available
Concept
Benefits
Drawbacks
Respiratory failure
H1N1 infection
vvECMO
Oxygenation only
CO2 only
vv ECMO, NovaLung
A membrane with inflow from systemic artery (femoral) with return to a systemic vein
No systemic heparinization, allows lung rest as ventilation for CO2 removal is decreased
Of limited use for oxygenation
IVOX
Requires normal LV function
CO2 and O2
vv ECMO
Full pulmonary support when sufficient cardiac function to overcome resistance through the membrane (?)
Complete respiratory support, easy (?) to institute, widely available (?)
Requires full anticoagulation….
va ECMO
Combined resp and cardiac failure
v-a ECMO
Full pulmonary and right ventricular support using membrane oxygenator and pump
Full support …
Requires full anticoagulation, limits ability to ambulate and care for patient
The support system most frequently applied is extracorporeal membrane oxygenation (ECMO), but technologies developed include several conceptually different devices. ECMO allows for a “pump system” to pass blood extracorporeally through a membrane gas exchange device and returns it to the patient [24, 25]. The path of the blood flow is determined by the mode of ECMO used, either Veno-Venous (VV) or Veno-Arterial (VA) circulatory support. The designation refers to the site of vascular drainage and perfusion (in and out of the systemic circulation), i.e. the cannulation sites. Both approaches may provide very effective gas exchange support in end-stage respiratory failure, but only VA ECMO provides additional hemodynamic support. VV ECMO predominantly provides oxygenation and CO2 elimination whereas VA ECMO supports systemic perfusion at the same time. A standard circuit design includes a centrifugal driver or roller pump and a membrane oxygenator with a heparin bonded tubing perfusion system.
There have been significant technological refinements of pump design with centrifugal drive devices being the latest development. An increasingly frequent approach for support for respiratory failure utilizes a dual lumen cannula for institution of VV ECMO via single site percutaneous internal jugular vein insertion. This can greatly facilitate application of ECMO [25, 26].
ECMO systems require a “pump” generating the blood flow to perfuse the oxygenated blood. Alternative designs simplify the approach and have relied on the patient’s arterial perfusion pressure to provide extracorporeal gas exchange. The most widely established system in this category is the Novalung® which allows CO2 elimination via a veno-arterial shunt principle with a polymethylpentene diffusion membrane inter-positioned [14]. In essence this is the same approach as for arterio-venous hemofiltration. In addition to extracorporeal systems, there have been intravascular devices for oxygenation such as the diffusion limited oxygenator (IVOX®) [27]. This enables permissive hypercapnia with an indwelling catheter based application.
There are device specific advantages and limitations according to each approach. Few clinical guidelines for application exist and several devices are still in investigational phases and will not be discussed in this chapter. A third category, fully implantable artificial lung devices, are currently experimental in animal models and have not been established in clinical practice outside of such scientific trial protocols. These are not discussed in this review.
Indications for Lung Support Systems
The indications for lung support can be categorized according the reversibility of the lung injury and whether the lung is native or a transplant. The criteria leading to the implementation of lung assist systems may depend on a reversible etiology with a strategy to support to native pulmonary recovery. Non-reversible, i.e. end stage pulmonary disease indications may be present when the patient is considered a candidate for lung transplantation [28]. Essentially three categories exist.
Indications:
A.
Reversible injury to the native lungs, i.e. bridge to recovery
B.
Reversible injury in a transplanted lung: e.g. acute allograft failure
C.
Irreversible injury to native lung, i.e. bridge to transplant
The diagnostic spectrum for lung device support is broad and reflects the specific clinical scenario, few contraindications are generally considered applicable across these scenarios. These include: sepsis and acute sepsis syndromes, irreversible coagulopathy and irreversible injury to damage to other end organ systems without likely chance of recovery. This is particularly pertinent to severe neurological injury from anoxia or stroke.
Respiratory failure encompasses failure in ventilation, oxygenation and CO2 clearance. This is often combined with a variable degree of cardiac dysfunction, particularly right heart failure. The lung support technologies available address these different components of respiratory failure to a variable extent.
Respiratory Dysfunction (Oxygenation and CO2 Clearance) and Cardiac Dysfunction
VA ECMO is a modality which removes systemic venous blood and delivers into the central arterial system. It works in parallel to the native pulmonary circulation and is designed to optimize perfusion pressure and oxygen delivery. Driver devices help support mean arterial pressure in addition to improving oxygen saturation. This is measured at the capillary vascular bed with simultaneous monitoring of sufficient preload to maintain left ventricular output. This modality offers near compete cardiopulmonary support.
Respiratory Dysfunction (Oxygen and CO2) Without Hemodynamic Compromise
VV ECMO is typically used for primary respiratory failure when no significant haemodynamic support is required. Generally, for this approach to be effective, cardiac output is preserved in the patient and specific evaluation shows no likely acute cardiogenic deterioration. Due to the type of VV ECMO support, there are unique considerations for systemic monitoring and daily management when compared with VA ECMO. Blood flow rates for VV ECMO are typically sub-maximal blood flow rates (calculated in L/min for BSA or BMI). This allows for optimized oxygen delivery and CO2 elimination with reduced peripheral arterial embolic risk as no arterial return cannula is required. In patients with predominant failure of CO2 clearance but preservation of oxygenation and hemodynamics, the NovaLung® device provides a further alternative.
General Considerations Regarding Indications and Outcomes
Regardless of the primary cause of lung failure, respiratory decompensation is followed by either hypoxia, hypercarbia or both [29, 30]. As described, not all device systems are effective in ameliorating both components of respiratory failure and lung assist technology relies on uncoupling of oxygen delivery versus CO2 removal primarily to allow for the use of the ventilator with a maximal lung preservation strategy such as minimal lung volumes, ventilation pressures and lower FiO2 [7]. The ultimate goal with these devices is to allow for resolution of the primary lung injury or to allow time for a transplant (in the case of irreversible lung failure) to occur.
Lung replacement therapy in contrast aims to provide oxygenation and ventilation in a more complete and implantable option. Replacement of other physiological properties of lungs, including metabolic and vascular functions as well as immunologic properties do not feature in any of the devices currently available. These two aspects will not be discussed further.
The complexity of the clinical and logistic issues involved imply that ECMO is best provided in dedicated programs. It is reasonable to assume that this is likely associated with optimal outcomes. Resources and manpower requirements ought to be met with adequate protocol provision and procedural guidelines. Interventional lung supplement devices not requiring perfusion services such as the NovaLung® maybe more readily available to critical care units. They nonetheless require specific expertise discerning correct indications and planning destination care with respect to the primary etiology. A detailed evaluation has to rule out all contraindications and other irreversible end-organ failure in particular when patients are selectively considered as candidates to bridge to lung transplantation [11, 31].
The second indication in this context is to provide support to recovery after primary graft dysfunction (PGD) in the lung recipient [32, 33]. In this setting lung assist devices may significantly improve outcomes if commenced early after onset of PGD, ideally prior to onset of multi-organ impairment or sepsis [34]. The quality of evidence however is limited by the lack of prospective studies. For lung transplant indications, including primary graft dysfunction, it is essential that ECMO support is combined with protective lung ventilation strategies [7, 8]. It is clinically intuitive that the reduction of ventilator induced lung injury (VILI) with low tidal volumes and permissive hypercapnia contribute in these settings. Optimizing systemic and respiratory physiotherapy including ambulatory protocols have been introduced with some clinical success, but remain a highly individual indication rather than an evidence based recommendation at present [35].
Results of Use
Determining the quality of evidence supporting the use of lung assist devices is hampered by both the heterogeneous nature of the conditions which constitute the indications as well as the variety of devices available. A further complication is the very rapid development of these technologies which are continually under development and have not matured sufficiently to undergo trials which produce the high quality evidence which we seek. However, some assessment must still be made to guide clinical care.
Search Strategy
We evaluated the available literature by undertaking a systematic search of the following databases: PubMed, EMBASE and the Web of Science. An extensive search strategy was used that yielded 2,012 titles and abstracts. The full text of 157 relevant articles were retrieved and reviewed. To assess the value of these interventions we limited our considerations to mortality as the beneficial outcome and neurological injury as the harm outcome.
We included both observational studies and trials which provided data on mortality and neurological outcome in adult patients were evaluated. We excluded animal studies and studies in children. We considered the evidence according to the clinical indications for use. We have loosely applied the GRADE framework to judge the quality of the evidence supporting the use of these modalities to improve survival [36–38].
Results
Reversible Injury to the Native Lungs—Bridge to Recovery
There were a number of case series but only three clinical trials using ECMO for reversible lung failure. The two older studies published in 1979 (Zapol) and 1994 (Morris) have little applicability to current practice as they used now obsolete ECMO technology and conventional management techniques [21, 39]. The major study informing current practice is the CESAR trial [40]. This trial compared a strategy incorporating ECMO to one without. There were 766 patients screened from 148 centres in the United Kingdom. Of these 180 patients met the inclusion criteria (age 18–65, had Murray score of >3.0 or pH of <7.20 and potentially reversible respiratory failure). Patients were excluded if they had high ventilation pressures or high FiO2 for more than 7 days, intracranial bleeding, contraindication to heparinization or other contraindication for continued active treatment. The patients allocated to the ECMO arm (n = 90) were transported to a single ECMO centre, while those allocated to the conventional arm remained in their local hospital or were transferred to a regional centre without ECMO capabilities. Of those who were allocated to the ECMO arm, 68 (75 %) actually received ECMO (venovenous) and in these patients 43 (63 %) survived 6 months without disability. In the 17 patients who did not receive ECMO, 14 survived. The relative risk of death in the overall ECMO arm was 0.69; 95 % CI 0.05–0.97. The overall survival rates in the ECMO arm was 63 % and in the control arm it was 47 %, with an absolute risk reduction of 16 % with number needed to treat 6.25. No neurological injury was noted in either group in this trial. Although this study was a carefully done randomized controlled trial, there are concerns related to the study design such as no limitation on the treatment of the conventional arm and further that randomization necessitated transfer to a centre which not only provided ECMO, but also likely to provide expert non-ECMO ARDS care [41].
A recent systematic review was carried out which combined the results of the CESAR trial with two observational studies with matched patients. The authors concluded that the utility of ECMO is still unclear as their results were very sensitive to reasonable assumptions on the type of analysis and the differences in patient populations where the two recent observational studies were H1N1 patients who were on the whole younger and sicker, but could not be matched and therefore could not be adequately assessed within the study [42, 43].
Although the CESAR RCT could be considered as providing high quality evidence for the use of VV ECMO for reversible lung injury we have reservations about the study design and its applicability our question—does the provision of VV ECMO in itself improve outcome. When considering the inconsistency of results among the two high quality observational studies, we judge that the overall quality of evidence is moderate. Currently, there is an ongoing trial, Extracorporeal Membrane Oxygenation for Severe Acute Respiratory Distress Syndrome (EOLIA) NCT01470703, which may help to clarify this issue further [44].
Review of available Novalung® data revealed a small subset of reports. These studies describe various levels of success with the intervention, but given that they are all observational studies they are considered relatively low quality evidence. Further prospective studies are needed.
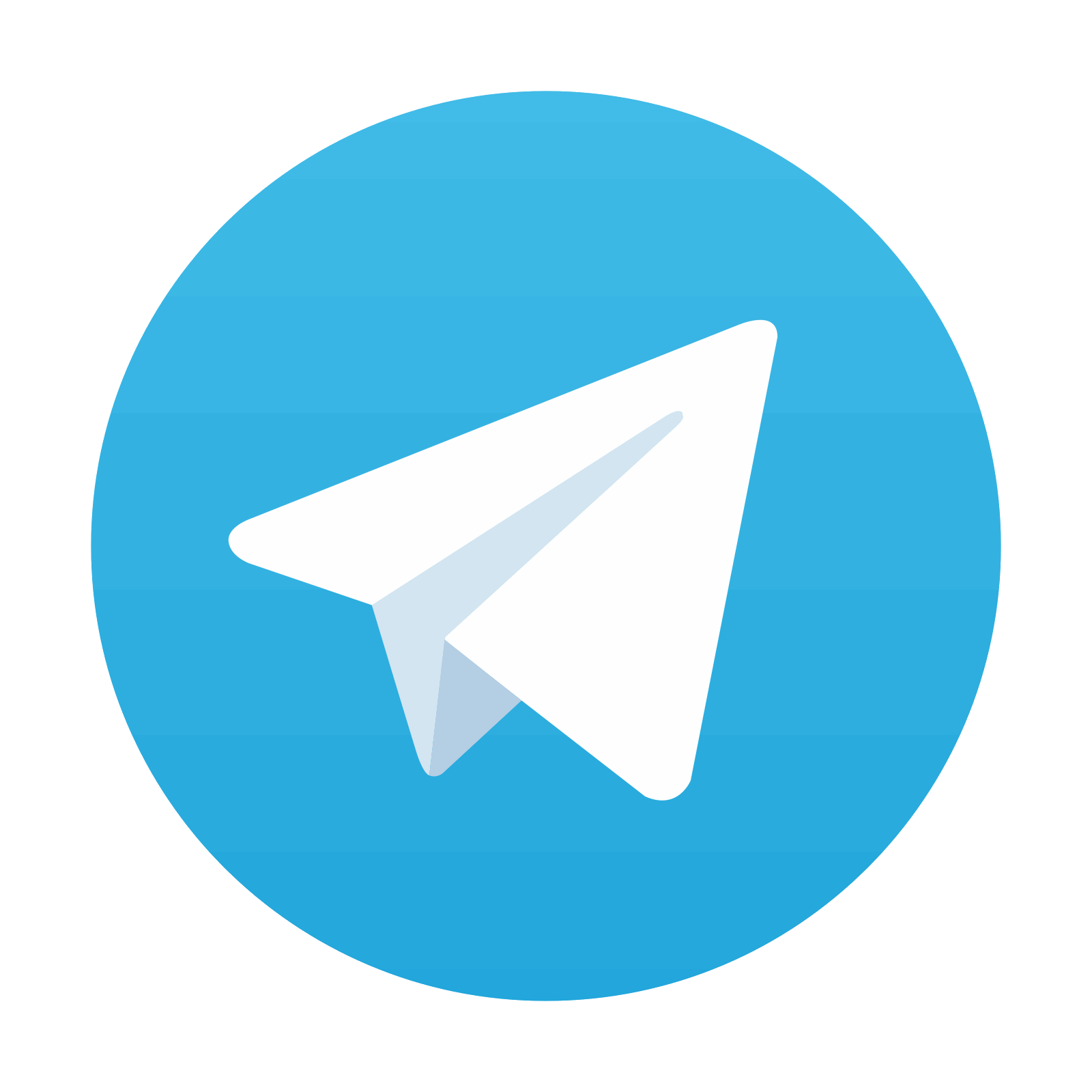
Stay updated, free articles. Join our Telegram channel

Full access? Get Clinical Tree
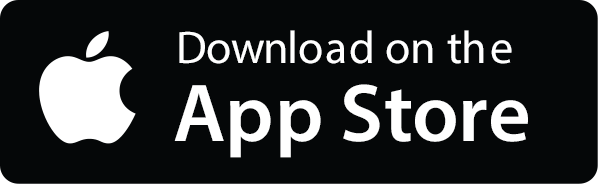
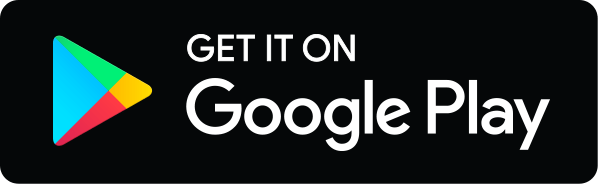