16 Stress Echocardiography with Nonexercise Techniques
Principles, Protocols, Interpretation, and Clinical Applications
Background
Indications
Although the inability of a patient to exercise maximally constitutes the main indication for the use of nonexercise stressors, such stressors are also indicated in other situations (Box 16-1). The most important of these situations include circumstances in which pharmacologic stress may offer diagnostic dimensions that are not available from exercise stress, for example, the diagnosis of coronary spasm using ergonovine testing. Although newer and simpler methods such as contrast-enhanced magnetic resonance imaging (MRI) have assumed a major role in viability assessment (see later discussion), stress echocardiography may be helpful in selected patients early or late after myocardial infarction, after which left ventricular (LV) dysfunction may not be permanent. The diagnostic challenge in this situation is that although stunned myocardium improves spontaneously, the revascularization of hibernating myocardium has implications for functional capacity and prognosis. Echocardiography can be used with dobutamine or dipyridamole stress, or both, for the identification of viable myocardium. A more historical role relates to the use of pharmacologic stress echocardiography in the cardiac catheterization laboratory—the functional evaluation of coronary stenoses having evolved to the evaluation of fractional flow reserve. Likewise, although use in the operating room was a legitimate reason to select pharmacologic stress, this is rarely performed in practice.
Box 16-1 Indications for Nonexercise Stressors
Unable to Exercise | Able to Exercise |
---|---|
CK, Color kinesis; TDI, tissue-Doppler imaging.
Finally, because of their relative technical ease, nonexercise methods have been used in preference to exercise echocardiography even in patients who are able to exercise. The incremental nature of these stressors means that the time course of ischemia can be identified; these data are difficult to gather using treadmill stress1 or bicycle exercise testing, although it is possible. The decision to use a nonexercise stress still may not be justifiable with routine echocardiographic imaging at present. However, the facilitation of adjunctive techniques (which may be less feasible during exercise than nonexercise stress)—such as strain and contrast perfusion echocardiography—could in the future justify the use of nonexercise techniques in patients who are able to exercise.
Choice and Mechanism of Action of Nonexercise Stressors
Exercise-Simulating Agents
The exercise simulators include dobutamine and other sympathomimetic agents, pacing, and atropine (usually used as an adjunct to the other approaches), all of which increase cardiac work and myocardial oxygen requirement. The induction of ischemia and regional dysfunction reflects the inability of the diseased coronary circulation to respond to this increased oxygen demand, a process that parallels the response to exercise. Because increased cardiac workload and oxygen demand is the usual mechanism underlying ischemia in most ambulatory situations, the exercise-simulating agents are often considered to be a more physiologic means of stressing the heart. However, additional mechanisms include effects on cardiac metabolism producing an “oxygen-wasting” effect2 and concurrent “supply” ischemia due to reduction of subendocardial perfusion and maldistribution of coronary flow.
Vasoactive Agents
The use of dipyridamole in combination with myocardial perfusion imaging is based on the induction of maximal coronary vasodilation in all territories. Flow heterogeneities (and therefore apparent perfusion defects) develop if a coronary stenosis limits the regional hyperemic response.3 Of note, this process may not involve the provocation of ischemia in a functional or metabolic sense. In contrast, the development of regional wall motion abnormalities at stress echocardiography necessitates the induction of ischemia.
The mechanism of vasodilator-induced wall motion abnormalities has been attributed to coronary steal.3 Traditionally, horizontal steal involves blood flowing to nonstenosed from stenosed territory, owing to reduced collateral flow into the stenosed territory because of depressurization of vessels supplying the collaterals due to increased runoff. Vertical steal occurs because vasodilator-induced depressurization of the microcirculation causes subendocardial vessels to collapse under the greater extravascular pressure in this region and “steal” flow to the subepicardium from the subendocardium. However, in experimental studies, steal appears less important than previously proposed, and vasodilator-induced ischemia has been attributed to the combination of reduced endocardial myocardial blood flow reserve with increased myocardial oxygen demand caused by hypotension and reflex tachycardia.4 The administration of atropine or increased sympathetic activity secondary to angina may augment this process. Systemic hypotension may certainly accentuate hypoperfusion, as driving pressure is the main determinant of myocardial perfusion when vasodilator reserve is exhausted. Stenosis “collapse” may be provoked if profound microcirculatory vasodilation causes a reduction of lateral pressure induced by increased flow.
Basic Principles
Pharmacology and Physiopathology
Exercise-Simulating Agents
Dobutamine is predominantly a beta-1 agonist; normal areas of myocardium become hyperkinetic in response to its inotropic effect. Segments supplied by a stenosed coronary artery may become akinetic or dyskinetic, but more subtly, and are unable to augment their thickening and excursion. Vasodilation and chronotropy appear at higher doses, usually at the 20 mcg/kg/min level of the routine dobutamine stress protocols, reflecting the stimulation of other receptors to a lesser degree. This chronotropic response appears to be the most important factor in terms of precipitating ischemia. Most dobutamine stress protocols cause a mean heart rate increment of 40 to 50 beats per minute and mean peak heart rates of 110 to 120 beats per minute.5 However, reflex bradycardia may occur in response to hypertension. Blood pressure normally rises in response to the inotropic effect of the drug, but high doses cause vasodilation and therefore cause blood pressure to fall at peak doses. Increases in myocardial oxygen demand due to increased cardiac work, as well as a weak vasodilator effect, lead to the development of coronary hyperemia in territories supplied by normal coronary arteries. Although this response is probably less than that induced by coronary vasodilators, the coronary hyperemic response to dobutamine stress permits its use with perfusion imaging, both scintigraphic and with microbubbles.6 The detection of myocardial perfusion defects in the presence of coronary disease may be augmented by partial volume effects due to thinning of the ischemic wall.
Vasoactive Agents
The principal coronary vasodilators are dipyridamole, adenosine, and regadenoson, which differ with respect to the onset and duration of their effects. Dipyridamole increases endogenous adenosine levels by reducing cellular reuptake and metabolism, and thereby acts indirectly.3 This indirect mechanism causes some delay between the administration of this agent and the timing of peak vasodilation, and this indirect action may account for interindividual variations in the magnitude of its effects. Adenosine acts directly on the vasculature, its vasodilator efficacy being equivalent to that of papaverine. The potency and speed of onset of adenosine cause its side effects to be more intense but also more short-lived than those of dipyridamole. By comparison to adenosine, the new A(2A)-specific receptor agonists have fewer complications but a similar mechanism of action.7 As with adenosine, however, their primary vasodilator role makes them more attractive for perfusion imaging than for wall motion assessment.
Combined Approaches
Atropine reduces the depressant effect of vagal stimulation on heart rate and may be particularly useful when reflex reduction of the heart rate occurs in response to blood pressure elevation. Atropine is combined with dobutamine in patients who fail to develop an adequate degree of tachycardia in response to dobutamine, the most common situation involving patients taking beta receptor antagonists. Tachycardia (and therefore ischemia due to increased oxygen demand) may be obtained by combining atropine with dipyridamole.8,9 In both circumstances, the addition of atropine to other stressors has been associated with an increment in sensitivity. Indeed, there is a trend toward earlier use of atropine during the dobutamine protocol, especially in beta-blocked patients. Early administration of atropine should not be allowed to interfere with the assessment of the low-dose response, so we do not administer this agent before the end of the 20 mcg/kg/min dose. A simple guide is that patients who fail to attain a heart rate of 70 beats per minute, or fail to increase heart rate by 5 beats per minute at this dose, rarely achieve target heart rate and might as well have the atropine early so as to avoid a prolonged test.
Echocardiographic Approach
Image Acquisition
The technical challenges of pharmacologic and pacing stress, although less extreme than those posed by exercise echocardiography, mandate the use of the best available equipment. The development of harmonic imaging has had a major impact on endocardial visualization10 and therefore on the feasibility of stress echocardiography.11 Although very few patients (less than 2%) have completely uninterpretable images, failure to detect the endocardium in every myocardial segment is very common, and contrast is indicated if two segments are obscured (Fig. 16-1).
Typically, transthoracic images are obtained in the standard views (parasternal long- and short-axis and apical four- and two-chamber). Two additional views are helpful—an apical long-axis view, which may overcome technical problems with parasternal imaging, and an apical short-axis view. Of the complementary imaging techniques, including contrast, strain, and three-dimensional (3D) imaging (see later section), the evaluation of coronary flow reserve in the left anterior descending artery is the simplest addition, although this seems to be most feasible in combination with adenosine.12
Although the constraints of a busy stress echocardiography schedule may preclude the performance of a detailed examination in every patient, a “screening” M-mode, pulsed wave (especially for LV inflow), and color Doppler echocardiographic examination should be performed at the beginning of every study. According to the setting, tissue Doppler images maybe helpful at baseline for the assessment of filling pressure13 and dyssynchrony.14 Time should also be taken to optimize two-dimensional (2D) echocardiographic images and gain settings (for endocardial detection) and to zoom on the ventricles (to obtain the best spatial resolution and frame rate). During the stress, the patient is imaged continuously, and the images are stored in digital clips. When the study is performed for the diagnosis of coronary disease, we favor a multiscreen display to show as many stages as possible, as changes may be transient. Many types of viewing software do not permit this. If so, the minimum default quad-screen display should include a resting image, a low-dose image (10 mcg/kg/min dobutamine, 0.56 mg/kg dipyridamole, or a small heart rate increment with pacing), a high-dose image (40 mcg/kg/min dobutamine or 0.84 mg/kg dipyridamole with or without atropine), and an early poststress image. The ability to save images at multiple stages enables easy alteration of this display. For example, when the clinical question pertains to the possibility of viable myocardium, the display could include two low-dose images (5 and 10 mcg/kg/min dobutamine) and one high-dose image.
Qualitative Interpretation
The interpretation of stress echocardiograms for clinical purposes is based on a qualitative evaluation of regional function at rest and stress.15 Some standardization is obtained by scoring regional wall motion and thickening in a number of segments. The American Heart Association has proposed a unified 17-segment model that includes the apical cap, to facilitate comparison between imaging modalities.16 Although this template ignores the small but important detail that the true apex is often not identified at echocardiography, its role as the standard should make its use preferred to the older 16-segment model (anterior, septal, lateral, and inferior at the apex, with these segments as well as anteroseptal and posterior segments at the base and midpapillary muscle level). Despite this standardization, however, the expertise of the physician interpreting the test is critical, and even accomplished echocardiographers require a significant learning period. In a study of echocardiographers learning dipyridamole stress echocardiography, Picano and colleagues reported that 100 supervised studies were required to bring the accuracy of “novices” to the level of “experts.” Despite many technical advances in stress echocardiography in the past two decades, this learning curve remains important.
The standard qualitative algorithm for interpretation of pharmacologic stress echocardiography is summarized in Table 16-1. With the exception of the low-dose responses, which denote myocardial viability, it may also be applied to pacing or other stressors.
Regional wall motion at rest may be classified as normal, hypokinetic, akinetic, or dyskinetic. Severe hypokinesia may be difficult to distinguish from akinesia; a useful guide is based on endocardial excursion, with less than 2 mm identifying akinesia and less than 5 mm indicating hypokinesia. Regions that fail to thicken or that move only in late systole (after movement of the adjacent myocardium) may be moving passively and should be considered akinetic, irrespective of endocardial excursion. Segments with resting akinesis or dyskinesis are most likely composed of infarcted myocardium if the wall is thinned and dense, but in the absence of thinning are likely to consist of viable tissue. Indeed, hypokinetic segments are not identified as being infarcted, on the basis that residual contraction implies the presence of residual viability. Because the process of thinning and fibrosis takes some time after infarction, myocardium of normal thickness is commonly seen after recent or nontransmural infarction. Improvement of abnormal function in response to low doses of dobutamine (5 or 10 mcg/kg/min) or dipyridamole (0.28 or 0.56 mg/kg) suggests the presence of viable myocardium (Fig. 16-2). This finding is more reliable if the segment subsequently deteriorates, which indicates ischemia.
The global LV responses to dobutamine stress include a reduction of cavity size and an increase in cardiac output—mainly due to increased heart rate rather than stroke volume after intermediate dose (e.g., 20 mcg/kg/min), a point that is pertinent to the LV strain response (see later discussion). LV dilation implies multivessel or left main coronary artery disease (see Fig. 16-2), but this is less commonly seen with pharmacologic than with exercise stress, probably because of reduction of loading. Together with an extreme inotropic response, this afterload reduction may produce cavity obliteration (Fig. 16-3) and even outflow obstruction—which is not meaningful. Although the LV cavity obliteration response is favorable prognostically (presumably reflecting a low probability of multivessel disease),17 it may impair sensitivity both because of reduced transmural wall stress and because endocardial excursion is reduced and small areas of wall motion are unrecognized.18 Use of intravenous beta-blockade allows the heart rate to slow rapidly after stress, filling the LV cavity and revealing wall motion abnormalities that were unidentified at peak stress.19 A similar outcome can be obtained by poststress imaging.
The normal response to inotropic stress is to increase endocardial excursion, speed of contraction, and degree of myocardial thickening. A deterioration of function from rest, or after an initial enhancement of function, indicates ischemia (Fig. 16-4). Variants of overt deterioration include delayed contraction (“tardokinesis”) and reduced myocardial thickening. Caution should be applied in the assessment of periinfarct zones, which may fail to improve function with stress, or even appear dyskinetic if the infarct bulges due to tethering by the infarcted zone. Examination of wall thickening may assist in distinguishing periinfarct ischemia and tethering.
The greatest limitations of the current application of stress echocardiography are concerns related to subjectivity and reproducibility. Although single-center studies have suggested that the interobserver variation in interpretation is small, interinstitution variability (although similar to that of other imaging techniques) is significant.20 Interinstitution variability is particularly a problem in studies of poor image quality and in patients with mild ischemia. This discordance has been progressively reduced by definition of standard reading criteria21 and the adoption of harmonic imaging.22 The following reading guidelines are useful for controlling variation: Minor degrees of hypokinesia are not identified as ischemia (especially if only apparent at peak and not at poststress readings); focal abnormalities that do not follow angiographic territories are ignored; abnormalities are corroborated whenever possible with another view; basal inferior and septal segments are not identified as abnormal in the absence of a neighboring abnormal segment; studies are read by multiple observers whenever possible; and reading is blinded to all other data.
Finally, a standard sequence is of value in the interpretation of stress echocardiograms. We first review the resting data or previous transthoracic echocardiography (TTE) for non–wall-motion data that may color our interpretation, including M-mode and Doppler components. On the digital images, we first check that images are triggered correctly and that the prestress, peak stress, and poststress views are comparable. We then briefly review all of the side-by-side displays to see if there are new wall motion abnormalities or obvious changes in cavity size (suggesting multivessel disease) or cavity shape. Segmental analysis is carried out by careful comparison of regional function in each of the 16 segments, comparing the same site at rest and at stress. Both wall motion and thickening are compared, using both cineloop and frame-by-frame review of the digital images. Freezing the images and stepping through one frame at a time is invaluable—delayed contraction is a hallmark of ischemic tissue that is not apparent to the unaided eye, but is often recognizable when images are reviewed frame by frame. The reader should think twice about identifying resting- or stress-induced wall motion abnormalities in segments that move rapidly and smoothly in early systole. Focusing on the first part of systole may minimize the contribution of rotational or translational movement, and assessing the timing of contraction may also avoid false positive interpretations provoked by early relaxation (Fig. 16-5). The ability to save multiple cycles in each view has reduced the need to review continuous capture stress images, but these remain of value for nonstandard views and to check on uncertainties arising from the digitized images. The site, extent (number of abnormal segments), severity (segmental wall motion score), and time of onset and offset of ischemia, as well as the effect of ischemia on global LV function, should be recorded.
Technical Aspects
Exercise-Simulating Agents
Protocols for Administration of Sympathomimetic Agents
Dobutamine has been administered using various empiric regimens, but none is clearly superior to the others. The most widely used is an incremental administration from 5 to 40 mcg/kg/min in 2- or 3-minute stages.5 Variants include maximal doses of up to 50 mcg/kg/min; lower dose-rates administered over longer periods are able to attain hemodynamic effects similar to those achieved by the high-dose protocols. New accelerated dobutamine protocols that have been used to reduce the duration of the test appear to be safe23 but may sacrifice the ability to identify a biphasic response. Whichever regimen is applied, a reasonable (i.e., 2- to 3-minute) period at peak heart rate is desirable, as ischemic wall motion abnormalities may take a short time to become apparent. If atropine is added to induce an increase in heart rate, the usual dose is 1 to 2 mg total in 0.25-mg increments.
Hemodynamic Responses to Sympathomimetics
Low doses of dobutamine enhance LV contractility, usually without the development of tachycardia. At doses greater than 20 mcg/kg/min, systolic blood pressure increases and heart rate augments to more than 100 beats per minute. Heart rate can be expected to increase to about 120 beats per minute in most patients, reflecting a increment of 40 to 50 beats per minute. In normotensive patients, systolic blood pressure usually increases to about 170 mm Hg (i.e., by 30 to 40 mm Hg)—less during dobutamine than during exercise. The systolic pressure response may be marked in hypertensive patients and blunted in patients with LV dysfunction or multivessel coronary disease. The peak rate-pressure product is usually about 20,000—less than with exercise,24–29 which has important implications for the relative sensitivity of dobutamine and exercise echocardiography. Although the development of ischemia at lower dobutamine doses and cardiac workloads indicates the presence of more extensive coronary disease, there is too much overlap for the hemodynamic response to be a reliable predictor of one-, two-, or three-vessel disease. The hemodynamic response to dobutamine shows significant variability between patients. Beta blockade may attenuate the physiologic response, with a significantly lower heart rate response (usually about 100 beats per minute), and a lower double-product (to levels of around 14,000), although this may be avoided by use of atropine.
Side Effects of Stress Testing with Sympathomimetic Agents
Other than the development of myocardial ischemia, dose-limiting side effects generally reflect intense adrenergic stimulation. Consequently, dobutamine stress is contraindicated in patients with severe hypertension and serious arrhythmias. Serious side effects are rare during DSE, with death, myocardial infarction, or sustained tachyarrhythmias in about 3 per 1000.8,30 Nonetheless, case reports of fatalities have been reported from ventricular fibrillation and cardiac rupture,31 and safety in patients at highest risk—those with severe LV dysfunction—has been described only in small populations.32 Nonetheless, considering that the sickest patients (those unable to exercise and with questions of myocardial viability) undergo the test, this safety profile is reasonable. This may reflect the detection of ischemia by online imaging, permitting termination before serious problems arise.
Less serious complications include palpitations (atrial and ventricular extra systoles), hypertension, anxiety (sometimes manifested as dyspnea or vagal reactions), tremor, and urinary urgency. Hypotension may arise from the vasodilator effect of high-dose dobutamine, the development of outflow tract obstruction, or the development of severe ischemia.33 Unlike hypotension during exercise testing, it does not denote the presence of serious coronary disease or LV dysfunction. In particular, the finding of an LV outflow tract gradient does not appear to be prognostically meaningful. The frequency of these side effects has varied from 5%, if only serious, dose-limiting side effects are considered, to 82%, if all side effects are included. Variability also relates to the type of stress protocol, the incidence being lower if the test is terminated for attainment of a target heart rate or at detection of ischemia or if submaximal test responses are excluded.
Vasodilator Stress Protocols
Stress Protocols
Because exogenous adenosine is able to act directly, it rapidly achieves steady state, and its biologic effects are of rapid onset. A fixed dose schedule of 0.14 or 0.17 mg/kg/min or incremental protocols (3-minute stages starting at 0.10 mg/kg/min, increasing to 0.14 and 0.18 mg/kg/ minute) been used for echocardiographic studies.34,35 The use of aminophylline is rarely required for side effects, as these resolve within 1 minute of stopping the infusion.
Hemodynamic Responses to Vasodilators
The mechanism of ischemia with of vasodilator agents is chiefly through coronary steal. There is a small contribution of increasing oxygen demand from tachycardia, which may be a response to angina and side effects rather than arising from the agents themselves. Similarly, blood pressure is usually little changed by these stressors, which may cause hypotension. The addition of atropine leads to an augmentation of heart rate and cardiac work,9 effectively combining reduced coronary supply with increased oxygen demand.
Side Effects of Vasodilators
Side effects during dipyridamole stress usually follow completion of the infusion, rarely preclude completion of the study, and usually resolve spontaneously. Minor side effects, including flushing and headache, occur in about two thirds of patients studied with high-dose dipyridamole. Severe or prolonged myocardial ischemia is infrequent but can be treated with nitrates or aminophylline. Serious side effects are very rare—probably less frequent than with dobutamine8,36—but severe myocardial ischemia and infarction, bronchospasm, complete heart block, and even death have been reported. Dipyridamole stress is contraindicated with atrioventricular block and bronchospasm, although patients with chronic obstructive airway disease with no or minimal airway reactivity may undergo the test.
The side-effect profile of adenosine is similar to that of dipyridamole, and although the intensity and frequency of side effects with adenosine is greater, they are of shorter duration. Some form of side effect occurs in most patients undergoing adenosine stress.37 In a high-dose protocol,35 side effects prevented about one third of patients from achieving peak dose, a comparable frequency to that found with dobutamine. Because the effect of adenosine is very transient, cessation of the infusion is usually the only treatment required for side effects. The use of regadenoson minimizes the risk of bronchospasm and heart block, is convenient because of a single dose, and has the same coronary vasodilator effect as dipyridamole,38 but regadenoson is suited to study of perfusion rather than wall motion responses.
Pacing Stress
Stress Protocols
The use of an implanted pacemaker to alter the heart rate during testing is often necessary to achieve target heart rate in the presence of atrioventricular block, even if pharmacologic stress is being used. Ventricular stimulation produces dyssynchronous contraction, but interpretable images are obtainable if image quality is sufficient to evaluate thickening, and atrial pacing poses no problems for interpretation.39 The test endpoints are the same as those for other nonexercise stressors; in the absence of these endpoints, pacing at peak heart rate is continued for 3 minutes. No protocol has been uniformly accepted. We use pacing in combination with dobutamine in order to obtain the inotropic response to stress, and therefore maintain a 3-minute incremental protocol when pacing is added, usually starting at 100 beats per minute.
Accuracy for Diagnosis of Coronary Artery Disease
Dobutamine Stress Echocardiography
The comparative sensitivity and specificity of DSE for the detection of coronary artery disease has been well summarized in two meta-analyses. Kim and colleagues40 reported an 80% (95% confidence interval [CI], 77% to 83%) sensitivity and 84% (95% CI, 80% to 86%) specificity in 82 pharmacologic stress studies between 1974 and 1999. In nearly 7000 patients from 62 DSE studies between 1991 to 2006, reported by Geleijnse and co-workers,41 the sensitivity was 81% and specificity was 82%. No single feature accounts for false-positive results, although the most frequent site of false-positive results is the basal inferior wall. The correlates of false negative results are a matter of discussion. The meta-analysis just mentioned did not show an effect from a variety of features conventionally associated with enhanced sensitivity—use of a coronary stenosis cutoff of 70% (rather than 50%), higher achieved heart rate, cessation of beta-blockers at the time of testing, the use of atropine, and the incorporation of a biphasic response. Armstrong42 has argued that these observations likely reflect the heterogeneity of testing in the meta-analysis, ranging from diagnostic testing for coronary artery disease, through evaluation of ischemia on therapy, to functional evaluation of coronary stenoses.
The use of a 50% or 70% stenosis cutoff is arbitrary and does not take into account stenosis location or vessel size, both of which clearly influence the likelihood of an abnormal response. This concern has been addressed in several studies that have compared the results of DSE with stenosis diameter at quantitative coronary angiography.43 The angiographic cutoff value with the best predictive value for a positive dobutamine test was a luminal diameter of 1.07 mm, percent diameter stenosis of 52%, and percent area stenosis of 75%, of which minimal lumen diameter was found to have the best predictive value for a positive dobutamine stress test (odds ratio, 51; sensitivity, 94%; specificity, 75%). Stenoses less than 1 mm in diameter can be identified with a sensitivity of 86%.43
The existence of a regional wall motion abnormality identifies the presence of coronary disease, so DSE demonstrates higher sensitivities in patients with myocardial infarction. In patients with single-vessel disease, the sensitivity of DSE (40% to 70%) is lower than in multivessel disease. Because dobutamine produces a less potent stress on the heart than exercise (see earlier discussion), DSE is probably more susceptible to external influences than exercise echocardiography or myocardial perfusion scintigraphy. This may be pertinent to the extrapolation of results from clinical trials (in which patients were carefully supervised and tested under optimal conditions, off therapy), to routine clinical practice, in which patients are often tested on therapy and are more likely to have a “suboptimal” test result because of inability to complete the protocol or interference by beta-blocker therapy.5
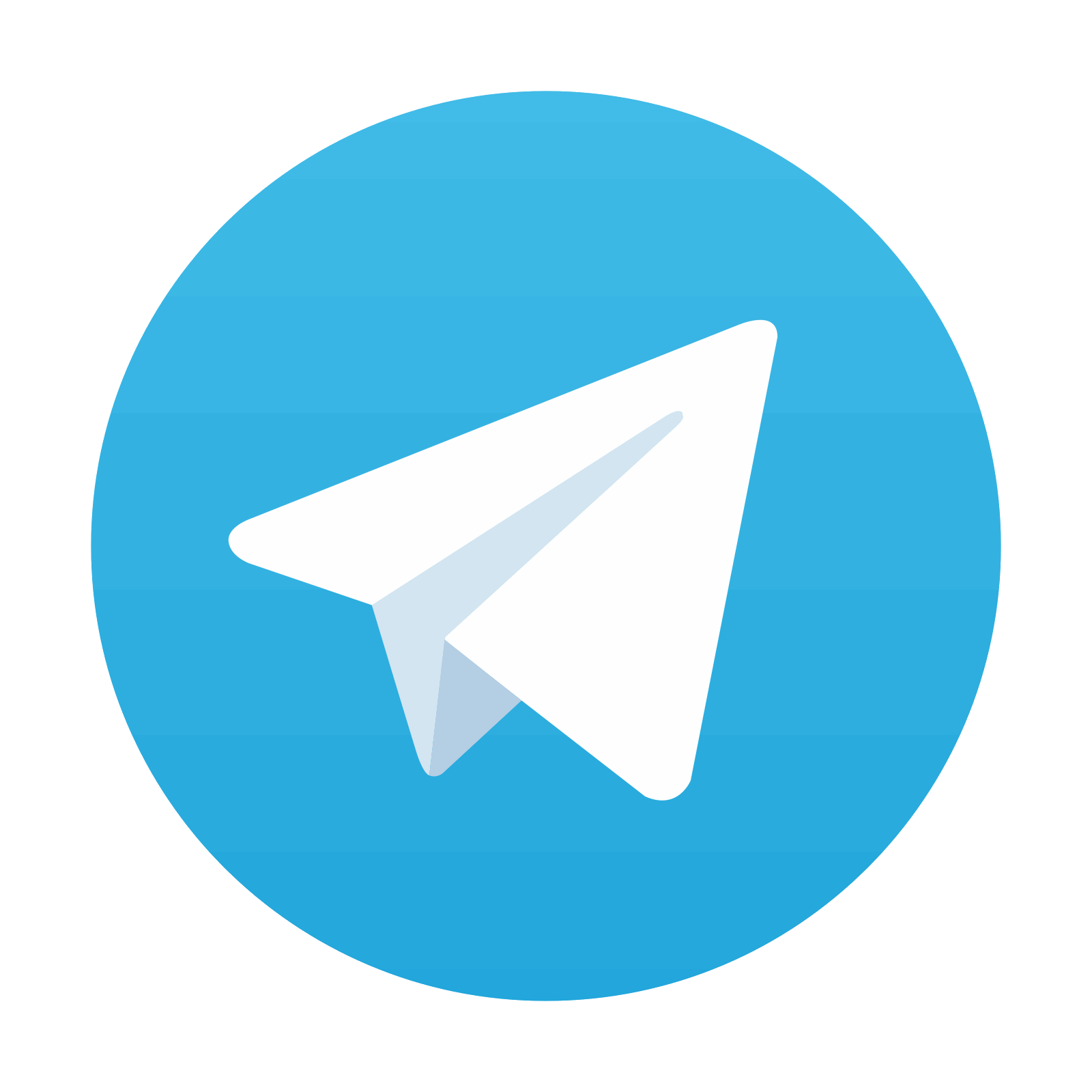
Stay updated, free articles. Join our Telegram channel

Full access? Get Clinical Tree
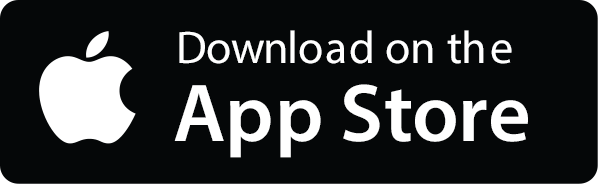
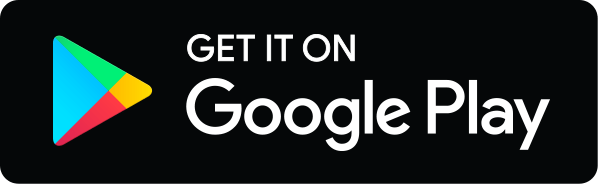