Background
The authors used semisupine cycle ergometry stress echocardiography to assess cardiac function and unmask baffle stenosis in patients with d-transposition of the great arteries after atrial redirection surgery.
Methods
This was a retrospective review of semisupine cycle ergometry stress echocardiography performed in 53 patients (64% male; mean age, 24.0 years; 90% Mustard procedure) and 56 healthy control subjects. Incremental exercise to volitional fatigue was performed. Hemodynamic data, echocardiographic cardiac dimensions, area change, tissue Doppler velocities, strain, ventricular synchronization, and superior vena cava flow velocities before and immediately after exercise are reported.
Results
Patients had lower exercise capacity (870 vs 1,854 J/kg, P < .001) and peak heart rates (132 vs 167 beats/min, P < .001). Stroke volume index did not increase with exercise (45 vs 47 mL/m 2 , P = .400). Cardiac index increased in both groups with exercise (3.0 vs 6.1 and 2.9 vs 7.0 L/min/m 2 , P < .001) and was higher in control subjects ( P = .006). Right ventricular diastolic and systolic areas decreased significantly with exercise in both the short-axis and four-chamber views. Right and left ventricular contraction time shortened with exercise (405 vs 247 and 338 vs 217 msec, P < .001) and remained synchronous (ratio of right ventricular to left ventricular contraction time = 0.080). Doppler velocities in patients with baffle obstruction were higher in the lower superior vena cava with exercise compared with nonobstructed patients (1.87 vs 1.46 m/sec, P = .020) and normalized after catheter intervention (1.49 vs 1.46 m/sec, P = .800).
Conclusions
Patients with d-transposition of the great arteries have lower exercise capacity and peak heart rates. The systemic right ventricle presents a lesser but qualitatively normal systolic response and decreased diastolic filling. Semisupine cycle ergometry stress echocardiography unmasked SVC obstruction.
Highlights
- •
d-TGA atrial switch patients have lower exercise capacity, heart rates, and cardiac index values.
- •
The systemic right ventricle has lesser but qualitatively normal systolic function.
- •
There is decreased RV diastolic filling.
- •
SVC obstruction can be unmasked using stress echocardiography.
D-transposition of the great arteries (d-TGA) is the second most common cyanotic heart lesion among congenital heart defects. In the 1960’s, atrial redirection with the Mustard and Senning procedures greatly enhanced the long-term prognosis of these patients. In the 1980’s, these procedures were replaced by a more physiologic repair, the arterial switch procedure. Currently, a significant number of patients who underwent Mustard or Senning operations have reached adulthood and are being followed in adult congenital heart disease clinics.
The atrial redirection operation is associated with long-term sequelae. Still, the survival rate at 20 years is up to 86%, and many of the patients are asymptomatic and report normal tolerance of regular daily activities. However, when challenged with exercise, they have limited exercise capacity compared with normal subjects.
A lack of adequate heart rate (HR) response to exercise, contraction dyssynchrony, decreased contractility and reduced ventricular filling rates have been suggested as factors compromising exercise capacity. An inadequate increase in stroke volume with exercise has raised the hypothesis of atrial baffle venous restriction, which may contribute to impaired exercise capacity in these patients. Cardiopulmonary exercise testing is a validated diagnostic and prognostic tool in patients with left ventricular (LV) failure and in selected forms of congenital heart disease. Graded maximal exercise testing provides an objective way to measure exercise capacity in these patients.
The forms of exercise testing used in many of these studies could not separate the components of cardiac function contributing to exercise tolerance. Semisupine cycle ergometry stress echocardiography (SSCE) is a technique that enables the measurement of echocardiographic and hemodynamic parameters and the assessment of load-dependent and load-independent indices of cardiac contractility and segmental wall motion during incremental exercise. Exercise, as performed in SSCE, is a more natural way of inducing cardiovascular responses compared with pharmacologic stress testing. The pattern of stroke volume response to exercise may reveal abnormalities that are not clinically apparent at rest and serve as a useful marker of myocardial function in children with heart disease.
Superior vena cava (SVC) baffle obstruction at the junction with the atrium is a well-described complication in patients following atrial redirection. The presence of a stenosis is not necessarily clinically significant. The diagnosis of a clinically significant stenosis may be difficult because patients may not present with classical symptoms, and blunting of the SVC Doppler pattern may not be a sensitive sign. Resting SVC Doppler velocities may not be increased and fail to raise the suspicion of baffle obstruction.
The purpose of this study was to document the exercise capacity and cardiac responses and, if possible, determine the mechanisms responsible for limiting exercise capacity in patients after the atrial redirection procedure using our stress echocardiographic protocol. In addition, we evaluated the use of SSCE to unmask SVC baffle obstruction in these patients.
Methods
This was a retrospective review of all semisupine cycle ergometry stress echocardiographic tests performed on patients with d-TGA between 1998 and 2013 at the Heart Centre at British Columbia Children’s Hospital. A total of 109 subjects, 53 patients who underwent atrial redirection operations and 56 healthy teenagers, were analyzed. All subjects had undergone SSCE at least once, 17 patients underwent two studies, and four patients underwent three studies during the study period, for a total of 130 studies. Demographic data were collected from medical records. Details of the primary procedure (Mustard or Senning), pacemaker insertion and pacemaker mode, previous diagnosis of venous obstruction, and determination of the relationship to catheter intervention (angioplasty with or without stenting) were collected. A total of 56 healthy teenagers underwent the same exercise testing protocol performed on the patients. They were evaluated as volunteers for control data or as patients with nonspecific symptoms and normal results on cardiac evaluation.
Ethics approval was obtained from the University of British Columbia Children’s and Women’s Health Centre’s Clinical Research Ethics Board.
Exercise Testing
Exercise testing was performed on an electronically braked semirecumbent cycle ergometer (Lode Angio Ergometer; Lode BV, Groningen, The Netherlands) ( Figure 1 ). The workload of the ergometer was increased in increments of 20 to 40 W every 3 min on the basis of the subject’s physical size. Patients were encouraged to exercise until volitional fatigue. A 12-lead electrocardiogram was monitored continuously (Case 8000 Stress System; GE Medical Systems, Wauwatosa, WI). Simultaneous blood pressure (BP), HR, and echocardiographic measurements were taken at rest, 90 sec into each stage of exercise, and immediately and 3 min after exercise. We report the results of the pre-exercise and immediate postexercise measurements. Exercise capacity was defined as cumulative work (joules per kilogram). HR was determined electrocardiographically. BP was recorded manually by sphygmomanometry.

Doppler Echocardiographic Protocol
Transthoracic echocardiography was performed by an experienced pediatric sonographer using a General Electric cardiac ultrasound system (GE Vingmed Ultrasound AS, Horten, Norway). A proprietary software program, EchoPAC version 112.1.3 (GE Medical Systems), was used for all offline analyses. All images and measurements were taken at rest and 90 sec into each stage of exercise. The immediate postexercise and 3-min postexercise images and measurements were obtained without delay and took between 60 and 90 sec to complete. We report the results of the pre-exercise and immediate postexercise measurements. Imaging started in the parasternal position with a long-axis view and an M mode through the ventricles, followed by modified long-axis and right parasternal views. Next, an apical four-chamber view was obtained, imaging the right and left ventricles for function, tissue Doppler sampling, pulsed-wave Doppler mitral and tricuspid valve velocities, and both outflow tract velocities. The upper SVC was imaged in a right suprasternal view or very high right parasternal view for pulsed-wave Doppler velocity, and a subcostal view was obtained to examine the lower SVC and pulmonary venous Doppler velocities ( Figure 2 ).

Aortic diameter was measured at the base of the valve in a modified long-axis view. Aortic cross-sectional area (CSA) was calculated in square centimeters as CSA = π × [(aortic diameter) 2 /4]. The velocity-time integral (centimeters) was sampled by pulsed-wave Doppler, with the sample volume placed at the level of the aortic annulus in a right parasternal view. Stroke volume index (SVI) (milliliters per square meter) was calculated from aortic CSA and velocity-time integral, normalized to body surface area. Cardiac index (CI) (liters per minute per square meter) was calculated as the product of SVI and HR.
Right ventricular (RV) internal dimensions in diastole and systole were measured from the M mode in a parasternal short-axis view. Because the geometry of the right ventricle makes volume measurements difficult, we used the areas in short-axis and four-chamber views as surrogates for RV volume, as reported by Shafer et al. The areas of the right ventricle during systole and diastole were determined in both the parasternal short-axis and four-chamber views ( Figures 3 and 4 ). The area change between systole and diastole was calculated in both views and averaged. Tissue Doppler was sampled in a four-chamber view for lateral wall, interventricular septum (IVS), and RV wall E-wave velocities, as well as RV wall S-wave velocity (centimeters per second). Circumferential strain (percentage) was calculated in the parasternal short-axis view, and longitudinal strain (percentage) was calculated in the apical four-chamber view. Speckle-tracking was used to calculate two-dimensional strain ( Figure 5 ). Evaluation of contraction synchronization was done in M mode in the short-axis view during systole. The times to IVS negative (toward the left ventricle) peak contraction, IVS positive (toward the right ventricle) peak contraction, and LV and RV peak contraction were measured. The ratio of each of these measurements divided by the R-R interval was calculated.



Venous flow velocities were measured by pulsed-wave Doppler using a suprasternal view for the upper SVC and a four-chamber view for the lower SVC and pulmonary veins. Tricuspid regurgitation was assessed by color Doppler imaging in a four-chamber view and subjectively by atrial color flow area and graded as 0 (no regurgitation), 1 (mild regurgitation), 2 (moderate regurgitation), or 3 (severe regurgitation). For analysis, they were combined to mild tricuspid regurgitation (grades 0 and 1) and moderate to severe tricuspid regurgitation (grades 2 and 3). Tricuspid and mitral annular plane systolic excursion was measured in a four-chamber view using M-mode echocardiography.
Statistical Analysis
Frequency tables were generated for all categorical data. The number and percentage are reported. Univariate analyses were used to analyze continuous variables. Mean values ± SD are reported. A repeated-measures analysis of variance was used to determine whether differences existed in our dependent variables over time (before and immediately after exercise). All tests were two sided, with P values < .05 considered to indicate statistical significance. All analyses were performed using SAS version 9.4 (SAS Institute, Cary, NC).
Results
Demographic Data
There were 53 patients (34 male [64%]) and 56 control subjects (21 male [38%]) enrolled in the study. The majority of the patients had undergone the Mustard procedure using pericardium in all ( n = 48 [90%]); the remainder had undergone the Senning procedure ( n = 5 [10%]). Twelve patients (23%) had pacemakers, and 15 patients (28%) had previous diagnoses of SVC obstruction. Patients were classified as having SVC obstruction by a combination of their clinical histories and the results of resting echocardiography, SSCE, and imaging by other modalities such as computed tomography, magnetic resonance imaging, and cardiac catheterization. Age (24.0 ± 6.9 vs 13.0 ± 3.0 years, P < .01), height (169.2 ± 9.6 vs 156.9 ± 13.0 cm, P < .01), weight (68.8 ± 15.4 vs 47.5 ± 11.5 kg, P < .01), body mass index (23.9 ± 5.0 vs 19.0 ± 2.8 kg/m 2 , P < .01), body surface area (1.78 ± 0.21 vs 1.44 ± 0.23 m 2 , P < .01), and aortic CSA (4.15 ± 1.07 vs 2.60 ± 0.44 cm 2 , P < .01) are reported for patients and control subjects, respectively.
Hemodynamic Data
A total of 130 studies were analyzed ( Table 1 ). The cumulative work of the patient group was significantly lower than among control subjects ( P < .001). Systolic BP increased significantly during exercise in both groups ( P < .001, P < .001) with a lower systolic BP immediately after exercise in the patient group ( P = .028). Diastolic BP remained unchanged. HR significantly increased during exercise in both groups ( P < .001, P < .001), but peak HR was significantly lower in the patient group ( P < .001). Baseline SVI was significantly higher in the patient group ( P < .001) but did not increase during exercise ( P = .400), unlike the control group, in which SVI significantly increased during exercise ( P = .009). CI increased significantly during exercise in both groups ( P < .001, P < .001), with a greater increase in the control group ( P = .006).
Parameter | Patients ( n = 53) | Control subjects ( n = 56) | Comparison between patients and control subjects | |||||
---|---|---|---|---|---|---|---|---|
Baseline | Immediately after exercise | P | Baseline | Immediately after exercise | P | P baseline | P immediately after exercise | |
Cumulative work (J/kg) | 870 ± 348 | 1,854 ± 560 | <.001 | |||||
Systolic BP (mm Hg) | 120 ± 12 | 163 ± 22 | <.001 | 118 ± 10 | 172 ± 21 | <.001 | .380 | .028 |
Diastolic BP (mm Hg) | 74 ± 9 | 73 ± 10 | .300 | 73 ± 8 | 72 ± 9 | .620 | .490 | .580 |
HR (beats/min) | 69 ± 15 | 132 ± 28 | <.001 | 78 ± 12 | 167 ± 20 | <.001 | <.001 | <.001 |
SVI (mL/m 2 ) | 45 ± 17 | 47 ± 19 | .400 | 37 ± 9 | 42 ± 10 | .009 | <.001 | .060 |
CI (L/min/m 2 ) | 3.0 ± 1.1 | 6.1 ± 2.4 | <.001 | 2.9 ± 1.0 | 7.0 ± 2.1 | <.001 | .550 | .006 |
Velocity-time integral (cm) | 15.8 ± 8.4 | 16.3 ± 8.8 | .650 | 20.0 ± 3.9 | 22.0 ± 3.47 | <.001 | .170 | <.001 |
Peak aortic velocity (m/sec) | 0.9 ± 0.2 | 1.4 ± 0.4 | <.001 | 1.0 ± 0.21 | 1.76 ± 0.29 | .007 | .080 | <.001 |
Cardiac Dimensions, Tissue Doppler, and Strain Data
The cardiac dimensions, tissue Doppler velocities, and strain results are presented in Table 2 . Statistical comparisons of these indexes for the patient and control groups was not performed, because the RV and LV architecture is so different that the results cannot be interpreted. There was a significant decrease with exercise in diastole and systole in M-mode RV internal dimensions ( P = .030, P = .006) and RV area in the short-axis ( P < .001, P < .001) and four-chamber ( P < .005, P < .001) views. Tissue Doppler RV E-wave and RV S-wave velocities increased significantly with exercise ( P = .001, P < .001), but lateral wall E-wave and IVS E-wave velocities remained unchanged. Radial and circumferential strain, tricuspid annular plane systolic excursion, and mitral annular plane systolic excursion did not change with exercise. There was no difference ( P = .800) in exercise capacity between patients with mild (grades 0 and 1) and moderate to severe (grades 2 and 3) tricuspid regurgitation.
Parameter | Baseline ( n = 53) | Immediately after exercise ( n = 53) | P |
---|---|---|---|
RV internal dimension in diastole, M-mode (cm) | 3.83 ± 1.1 | 3.42 ± 1.04 | .030 |
RV internal dimension in systole, M-mode (cm) | 2.69 ± 1.2 | 2.18 ± 1.18 | .006 |
RV area during diastole, SAX (cm 2 ) | 16.9 ± 5.8 | 12.1 ± 5.9 | <.001 |
RV area during systole, SAX (cm 2 ) | 10.2 ± 4.9 | 6.6 ± 4.7 | <.001 |
Average RV SAX area change (cm 2 ) | 0.41 ± 0.11 | 0.47 ± 0.15 | .020 |
RV four-chamber diastolic area (cm 2 ) | 25.6 ± 7.6 | 21.5 ± 6.6 | .005 |
RV four-chamber systolic area (cm 2 ) | 18 ± 6.6 | 13.9 ± 6.1 | .004 |
Average RV four-chamber area change (cm 2 ) | 0.29 ± 0.13 | 0.36 ± 0.14 | .009 |
TAPSE (cm) | 1.2 ± 0.6 | 1.1 ± 0.3 | .470 |
MAPSE (cm) | 1.5 ± 0.6 | 1.5 ± 0.6 | .620 |
Lateral wall E velocity (cm/sec) | 5.7 ± 3.4 | 6.1 ± 5.9 | .060 |
IVS E velocity (cm/sec) | 5.0 ± 3.2 | 6.1 ± 5.2 | .170 |
RV E-wave velocity (cm/sec) | 6.1 ± 3.9 | 9.4 ± 3.1 | <.001 |
RV S-wave velocity (cm/sec) | 4.8 ± 1.8 | 9.1 ± 3.4 | <.001 |
LV radial strain (%) | 29 ± 15 | 31 ± 13 | .260 |
RV radial strain (%) | 15 ± 9 | 20 ± 10 | .056 |
LV circumferential strain (%) | −18 ± 7 | −17 ± 8 | .370 |
RV circumferential strain (%) | −9 ± 3 | −10 ± 4 | .450 |
LV global strain (%) | −16 ± 7 | −17 ± 7 | .610 |
RV global strain (%) | −12 ± 4 | −10 ± 4 | .080 |
Contraction Synchronization Data
Synchronization data are presented in Table 3 . IVS time to negative peak, IVS time to positive peak, and time to peak LV and RV wall contraction all shortened with exercise ( P < .001). The ratio of each measured time (IVS time to negative peak [ P = .020], IVS time to positive peak [ P < .001], and time to peak LV [ P < .001] and RV [ P = .004] wall contraction) divided by the R-R interval prolonged with exercise. The ratio of time to peak RV/LV wall contraction showed no difference with exercise ( P = .08).
Parameter | Baseline ( n = 53) | Immediately after exercise ( n = 53) | P |
---|---|---|---|
IVS time to negative peak (msec) | 143 ± 38 | 95 ± 47 | <.001 |
IVS time to negative peak/R-R interval | 0.16 ± 0.05 | 0.2 ± 0.1 | .020 |
IVS time to positive peak (msec) | 374 ± 84 | 239 ± 90 | <.001 |
IVS time to positive peak/R-R interval | 0.41 ± 0.1 | 0.52 ± 0.17 | <.001 |
LV time to peak (msec) | 338 ± 44 | 217 ± 48 | <.001 |
LV time to peak/R-R interval | 0.37 ± 0.06 | 0.47 ± 0.09 | <.001 |
RV time to peak (msec) | 405 ± 81 | 247 ± 83 | <.001 |
RV time to peak/R-R interval | 0.45 ± 0.11 | 0.53 ± 0.16 | .004 |
RV time to peak/LV time to peak ratio | 1.20 ± 0.20 | 1.14 ± 0.26 | .080 |
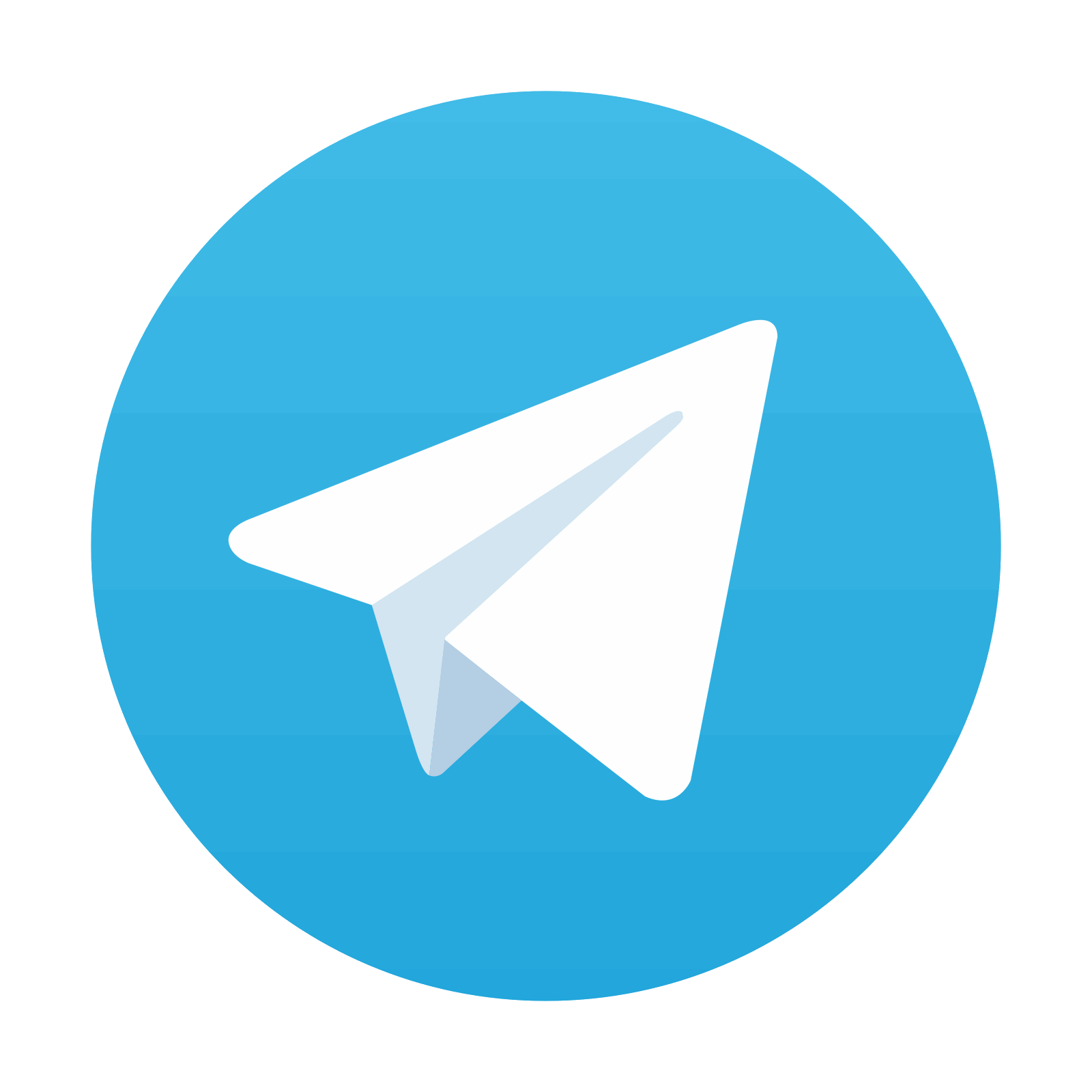
Stay updated, free articles. Join our Telegram channel

Full access? Get Clinical Tree
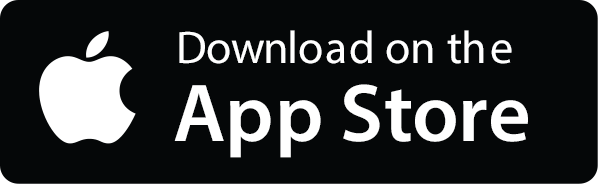
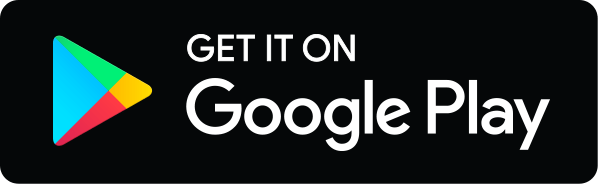
