Fig. 7.1
Heart failure and LVAD physiology. Ao aorta, PA pulmonary artery, LVAD left ventricular assist device, MR mitral regurgitation, TR tricuspid regurgitation, CO cardiac output, CVP central venous pressure, LAP left atrial pressure, PCWP pulmonary capillary wedge pressure, RAP right atrial pressure, LVEDP left ventricular end-diastolic pressure, LVEDV left ventricular end-diastolic volume, RVEDP right ventricular end-diastolic pressure, RVEDV right ventricular end-diastolic volume
Despite the positive effects of decreased RV afterload, the RV may also sustain negative effects influenced by the LVAD. RV function is related to contraction of its free wall and the position of the IVS. During LVAD support, RV contractility may be impaired by changes in IVS position and motion. When the LV is excessively unloaded, the IVS shifts markedly towards the LV and impairs efficient RV contraction. To compensate for the loss of IVS function, the workload of the free wall of the RV increases, causing exhaustion of the RV.
LVAD implantation causes an inconsistent change in the extent of perioperative TR. The severity of TR is worsened by the leftward shift of the IVS as well as increased pulmonary vascular resistance (PVR) caused by cardiopulmonary bypass (CPB), systemic inflammatory response syndrome (SIRS), and increased preload after blood transfusion. In addition, high flow of the LVAD may distort the tricuspid valve annulus.
7.3 Definition of Right Ventricular Failure
The preoperative condition of patients who require LVAD implantation includes a broad presentation of RV dysfunction, from asymptomatic to severe RV failure (RVF). In this patient population, RVF is defined as a clinical syndrome characterized by decreased ability of the RV to fill and eject appropriately or impaired function of the RV in providing adequate blood flow through the pulmonary circulation at a normal preload [20].
There has been no universal definition of RVF after LVAD implantation among authors because of the retrospective nature of the available studies. RVF occurs when the pulmonary circulation is unable to fill the LVAD despite maximal medical therapy. The need for a right ventricular assist device (RVAD) is accepted as an indicator of extreme RVF, and even without requiring RVAD, RVF is considered when conditions are such that hemodynamic instability requires pharmacological support, i.e., inotropes or pulmonary vasodilators, for >2 weeks. Patients with RV failure may also require a combination of the two [4, 6, 8, 21–23].
7.4 Evaluation of Right Ventricular Function
7.4.1 Modalities to Evaluate Right Ventricular Function
Right heart assessment is important for monitoring RV function and identifying possible causes of RV dysfunction. Assessment of the RV is sometimes challenging because of its anterior retrosternal position, complex geometry, and hemodynamic load dependence.
Echocardiography has been the most common method for RV evaluation. Three-dimensional echocardiography is a promising modality that can provide more accurate evaluation, although cardiac magnetic resonance imaging (MRI) has become a standard. Assessment of RV structure should include observations of the RV size, shape, volume, and wall thickness [25], and MRI is now considered the most reliable method for measuring RV volume [26, 27]. RVEF and RV fractional area change (RVFAC) are calculated on the basis of size and volume measurements determined by the imaging studies.
Tricuspid valve (TV) annular plane systolic excursion (TAPSE), which is an echocardiographic measurement of the longitudinal displacement of the TV annulus during systole, is used for evaluation of RV function. Displacement >15 mm in TAPSE is a quantitative measure of normal RV systolic function. However, TAPSE is less reliable in patients with focal RV dysfunction because it only measures the longitudinal movement of the lateral free wall. Evaluation of the TV annulus size, the presence and severity of TR, the inferior vena cava size, and the hepatic venous blood flow pattern are also important parts of the evaluation of RV function. Although there is good correlation among TAPSE, RVFAC, and RVEF, many cardiographers use a subjective assessment that classifies global RV function as good or as mildly, moderately, or severely reduced.
7.4.2 Evaluation After Left Ventricular Assist Device Implantation
Echocardiography is the primary imaging modality for evaluating cardiac function after LVAD implantation because MRI is not an option for patients with these devices [27]. Changes in RV size and the degree of TR are followed serially. When RV function is worsening, RV size and the extent of TR increase correspondingly.
In patients with LVAD, a decrease in the TAPSE is seen because RV afterload and RV contractile work to maintain cardiac output (CO) are reduced [17]. However, low TAPSE in the presence of increasing RV size and TR suggests worsening RV function [28].
An important structural feature in evaluation of heart function is the position the IVS, as it reflects various factors. A shift of the IVS may indicate RV dysfunction, inappropriate volume loading, incorrect LVAD setting, and LVAD device failure. Because of the space limitations imposed by the pericardium, potential right heart compression by fluid collection or thrombus should also be considered.
With continuous-flow LVAD support, preexisting RV dysfunction was not exacerbated during a median follow-up of 4.5 months [29]. Moreover, the size of RV chamber is reduced in parallel with that of the LV chamber, although the degree of the change in the RV is variable. In contrast, the pulsatile LVADs consistently decompressed the LV to a greater degree, thus impairing RV function by leftward shifting of the IVS.
7.5 Predictors of RV Failure
7.5.1 Risk Factors
Many studies have attempted to identify preoperative risk factors and develop risk scores in order to identify patients at the greatest risk for postoperative RV failure after LVAD implantation and to formulate the appropriate treatment strategies for them. Patients at high risk for postoperative RV failure would receive benefits from preoperative optimization of RV function and possibly even planned biventricular assist device (BiVAD) support. However, it is still difficult to predict the incidence of postoperative RV failure in the LVAD patients because RV failure includes multiple factors throughout the treatment.
Some studies have identified particular patient characteristics and hemodynamic parameters as risk factors for postoperative RV failure, but the significance of the findings is limited by small cohorts, single institution, retrospective nature, and inconsistent use of an LVAD type.
According to published studies, preoperative risk factors for postoperative RV failure in patients with LVAD are female gender; non-ischemic cardiomyopathy [30]; preoperative support, including mechanical ventilation, mechanical circulatory support [4, 8, 23], or intra-aortic balloon pumping (IABP) [6]; hemodynamic parameters; biochemical markers; and echocardiographic measurements.
The hemodynamic parameters that can indicate RV failure after LVAD implantation include elevated preoperative and intraoperative central venous pressure (CVP) and reduced RV stroke work index (RVSWI < 300 mmHg mL−1 m−2) [4, 8, 21, 31]. Although high PAP is an easily measured parameter, RV function, which may not always be related to high PAP, remains the most critical determinant of survival. In fact, patients without pulmonary hypertension are more likely to develop RV failure and have more serious morbidity after LVAD implantation. This situation indicates that decreased RV contractility is unable to overcome increased PVR. RVAD or BiVAD support should be considered for patients with poor RV function indicated by low PAP or RVSWI. Preoperative treatment, including a pulmonary vasodilator, is required for patients with chronic pulmonary hypertension associated with pulmonary disease.
Findings consistent with organ failure and hepatic congestion secondary to impaired preoperative RV function are also important risk factors for postoperative RV failure and BiVAD requirement. These signs include elevated serum creatinine (Cr) [4, 31], blood urea nitrogen [8], aspartate aminotransferase (AST), and bilirubin levels [4]. Increased nonspecific neurohumoral markers of heart failure, such as N-terminal pro-brain natriuretic peptide and neopterin, and inflammatory markers such as procalcitonin and big endothelin-1 are also counted as risk factors for RV failure after LVAD implantation [24].
Severe preoperative TR and a short/long axis ratio of >0.6 for RV have high specificity (87 % and 97 %, respectively) and good sensitivity (66 % and 37 %) to identify patients with high risk of postoperative RV failure [9]. Increasing severity of TR is also associated with the occurrence of postoperative RV failure. Puwanant et al. found that preoperative TAPSE <7.5 mm was better related to post-LVAD RV failure than RVFAC, with a specificity of 91 % and a sensitivity of 46 % [28].
7.5.2 Risk Scores
Other risk scoring systems have been developed to quantify the risk of RV failure after LVAD implantation. Rao et al. revised a previous screening scale to more accurately predict survival after LVAD implantation and to determine emerging risk factors for mortality (Table 7.1). In their study, mechanical ventilation and a previous LVAD were independent predictors of mortality after device insertion. The correlation between observed and predicted values was better in the revised score than in the old score, which overpredicted mortality in patients at low risk and underpredicted mortality in patients at high risk [32].
Table 7.1
Risk score for mortality after LVAD implantation
Rao et al. [32] | |
---|---|
Preoperative variables | Weighting |
Ventilated | 4 |
Postcardiotomy | 2 |
Pre-LVAD | 2 |
CVP >16 mmHg | 1 |
PT >16 s | 1 |
Total points | Mortality (%) |
Risk score | |
>5 | 6 |
≤5 | 12 |
Through multivariable regression analysis, Drakos et al. found seven preoperative variables that were correlated with postoperative RV failure, including destination therapy, IABP, and increased PVR [22] (Table 7.2). They reported that the incidence of postoperative RV failure was 44 %, although their study included various pulsatile and non-pulsatile LVAD systems. The authors defined RV failure as requirement for inotropic support for >14 days, iNO for >48 h, or RVAD implantation. Patients were stratified into four risk groups from a sum of all points assigned to each variable. The incidence of RV failure was 11 % in the lowest risk group, while in the highest group, it was 83 %. Destination therapy, elevated PVR, and preoperative IABP support were significant independent predictors for postoperative RV failure.
Table 7.2
Risk scores for RV failure after LVAD
Matthews et al. [4] | Drakos et al. [22] | ||
---|---|---|---|
Preoperative variables | Points | Preoperative variables | Points |
Vasopressor use | 4 | Destination therapy | 3.5 |
Cr ≥2.3 mg/dL | 3 | IABP | 4 |
Bilirubin ≥2 mg/dL | 2.5 | PVR | |
AST ≥80 IU/L | 2 | 1.7 | 1 |
1.8–2.7 | 2 | ||
2.8–4.2 | 3 | ||
>4.3 | 4 | ||
Inotrope dependency | 2.5 | ||
Obesity | 2 | ||
ACE or ARB | 2.5 | ||
β-blocker | 2 | ||
Total points | Odds ratio | Total points | Risk (%) |
Risk score for RV failure | |||
≤3.0 | 0.49 | <5 | 11 |
4.0–5.0 | 2.8 | 5.5–8.0 | 37 |
≥5.5 | 7.6 | 8.5–12 | 56 |
>12.5 | 83 |
In a study with 197 patients undergoing LVAD, Matthews et al. reported that 35 % of the patients developed RV failure and that the independent predictors were the use of vasopressors and increased AST, bilirubin, and Cr levels; using these predictors, they developed a risk score system [4] (Table 7.2).
Fitzpatrick et al. proposed a similar risk score from a cohort of 266 patients after implantation of various types of LVAD [31]. In their study, they defined RV failure as the need for RVAD, which was found in 34 % of the patients. Using preoperative risk factors associated with postoperative RV failure, they proposed a risk score for RV failure based on multivariate logistic regression analysis. According to the scoring system, a score >50 is an indication for a BiVAD and has good sensitivity (83 %) and specificity (80 %).
The National Institutes of Health-sponsored Interagency Registry for Mechanical Assisted Circulatory Support (INTERMACS) developed a systematic scoring system through the largest LVAD database in the USA [33]. Alba et al. compared INTERMACS level I and II (sicker and decompensating) to level III and IV patients, showing that the system is a good predictor of postoperative complications and mortality, but not sensitive for postoperative RV failure [34].
7.6 Prevention and Management of RV Dysfunction
It is crucial to pay meticulous attention to optimizing preload, afterload, and contractility in patients with preexisting RV dysfunction in order to prevent RV failure (Fig. 7.2). Even simple measures such as maintenance of sinus rhythm or AV synchronicity, adequate ventilation, temperature, and acid–base balance should be taken to prevent RV failure. Few studies have assessed the impact of preoperative management on post-LVAD RV failure.
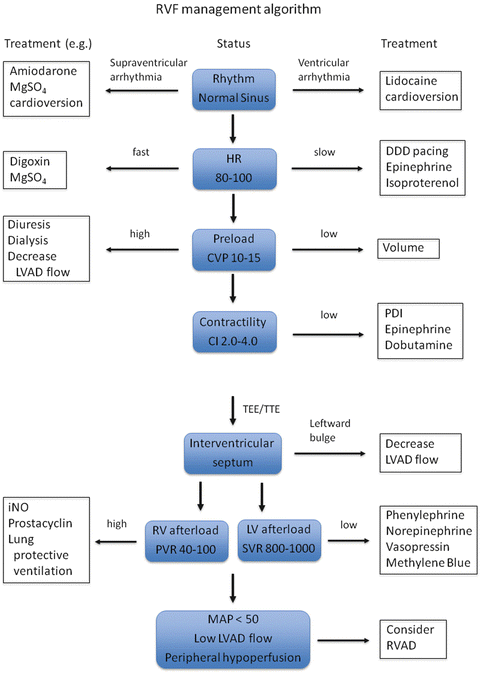
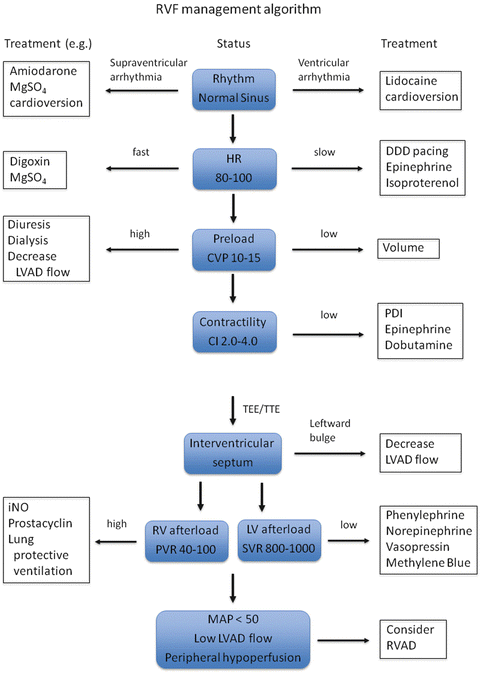
Fig. 7.2
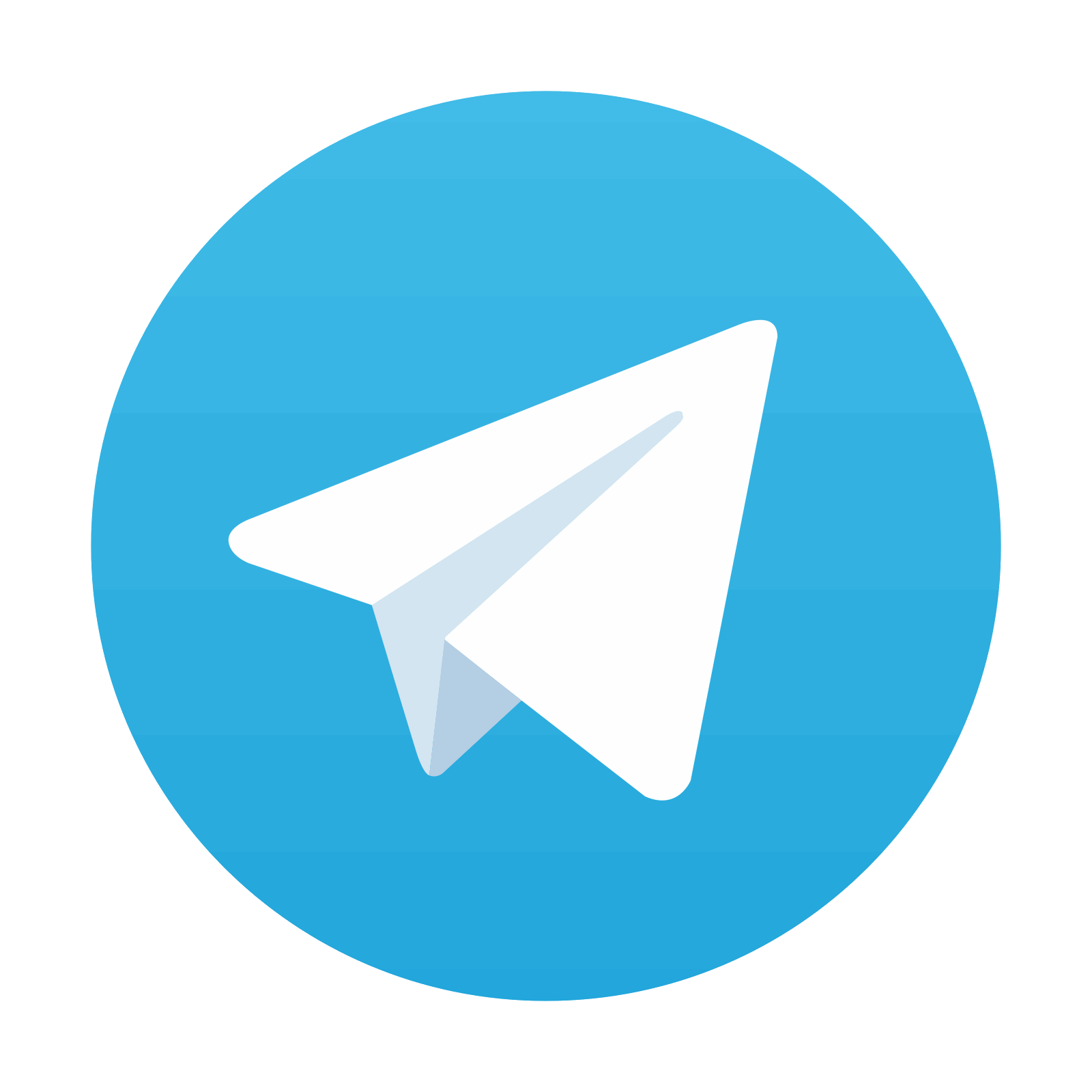
Management algorithm for RVF. RVF right ventricular failure, LVAD left ventricular assist device, MgSO 4 magnesium sulfate, HR heart rate (beat min−1), CVP central venous pressure (mmHg), CI cardiac index (L min−1 m−2), PDI phosphodiesterase inhibitor, TEE transesophageal echocardiography, TTE transthoracic echocardiography, RV right ventricle, LV left ventricle, iNO inhaled nitric oxide, PVR pulmonary vascular resistance (dyne s cm−5), SVR systemic vascular resistance (dyne s cm−5), MAP mean atrial pressure (mmHg), RVAD right ventricular assist device
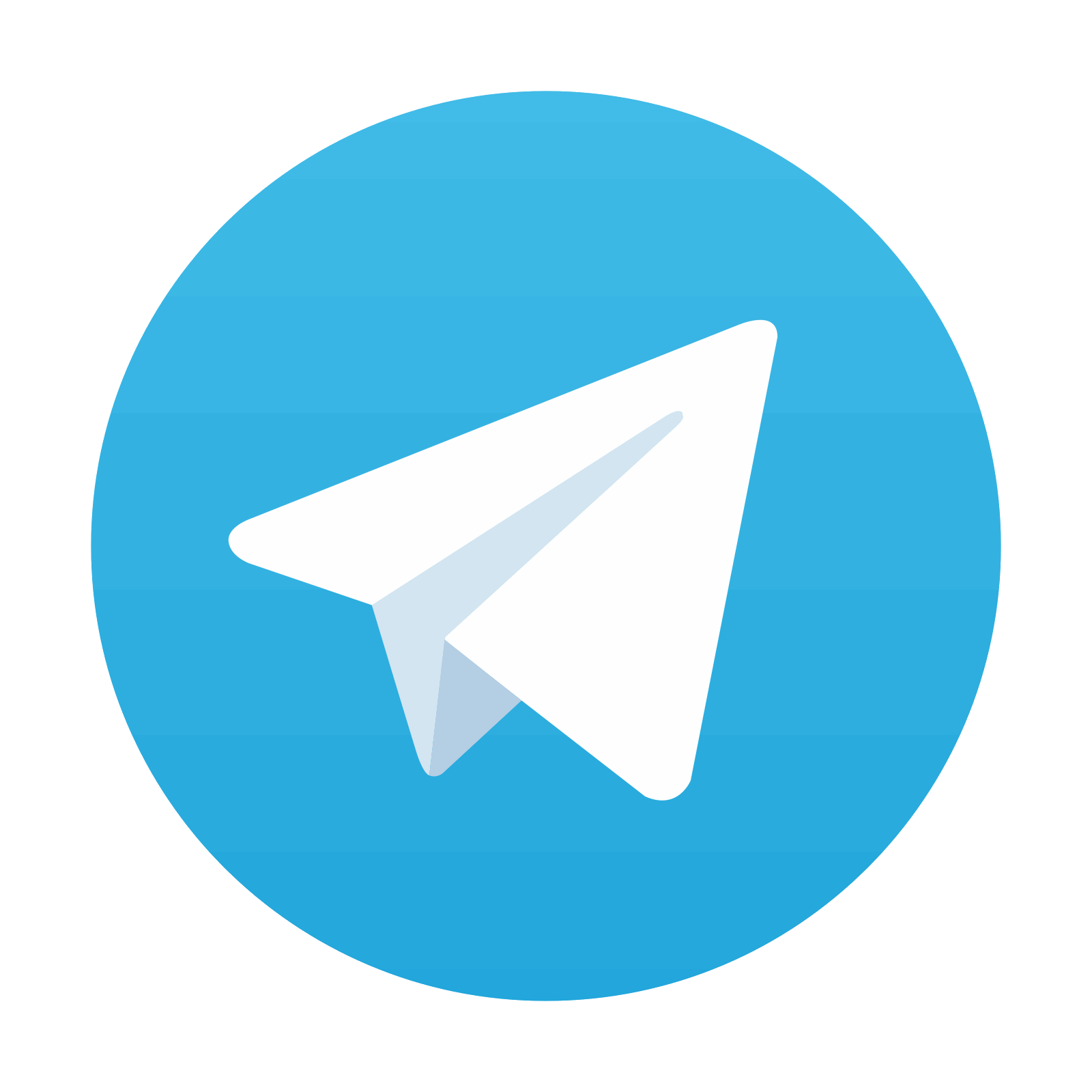
Stay updated, free articles. Join our Telegram channel

Full access? Get Clinical Tree
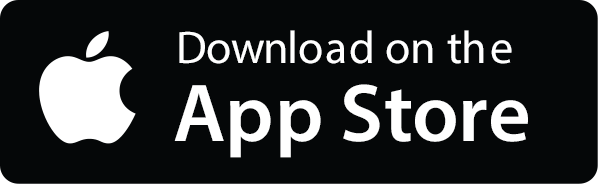
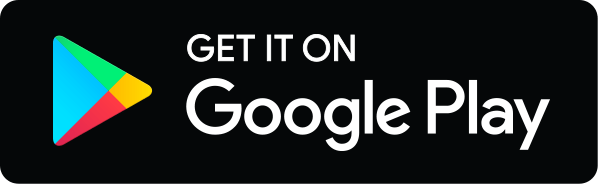
