© Springer-Verlag London 2017
Howard Eisen (ed.)Heart Failure10.1007/978-1-4471-4219-5_3131. Stem Cell Therapy in Heart Failure
(1)
Washington Hospital Center/Georgetown University Hospital, Department of Cardiology, 110 Irving Street, Washington, DC, 20010, USA
(2)
Miami, FL, USA
Keywords
Stem CellsHeart FailureCardiac RegenerationCardiovascular DiseaseMyocardial InfarctionIntroduction
Despite advances in prevention and treatment, cardiovascular disease (CVD) is still the leading cause of morbidity and mortality in the United States. As mortality from CVD declines, and more of our patients survive acute myocardial infarction, revascularization procedures, arrhythmias, and treatments for congenital and acquired valvular and structural heart disorders, so the prevalence of heart failure (HF) continues to increase with more than 550,000 new cases annually [1]. Half of humans with HF will die within 5 years of onset; it was cited as a contributing cause in more than 280,000 deaths in 2008 [1]. HF is also well-known to be extremely costly, with health care services, medications, and lost productivity equating to a U.S. national burden of $34.4 billion each year [2]. This high clinical and economic cost provides great impetus for the development of new therapeutic approaches for HF.
While it was long taught that that the human heart had no capacity for regeneration because cardiac myocytes existed in a terminally differentiated state, a number of lines of evidence have overturned that idea. While the rate of cell turnover remains a topic of debate [3], demonstration in humans of chimerism of a sex mismatched transplanted heart [4], of mitotic figures in myocardium after infarction [5], and of differential integration of Carbon-14 (from Cold War nuclear bomb tests) [6] suggested that some turnover of myocytes does occur in humans. Demonstration of myocardial regeneration after infarction in animal models [7] initiated a new field for translational research.
The therapeutic cell types [8], mechanisms of action, routes of administration, and treatable conditions [9] are now beginning to be defined. This chapter will review briefly some of the significant clinical trials in the field of myocardial regeneration for the treatment of heart failure. We argue that a relatively rational progression from translational studies to clinically informative trials [10] is now underway.
Stem Cells
Toronto scientists Till and McCulloch described stem cells in 1963 in their work on the radiation sensitivity of mouse bone marrow cells [11]. Stem cells are cells that can continuously self-renew and undergo differentiation to a cell lineage [12]. Stem cells are described as pluripotent if they can form all the cell types of the adult organism and totipotent if in addition they can form embryonic tissues. Once a stem cell is confined to a certain tissue, its potency is generally limited to differentiation into cell types of that tissue. Besides heart failure [13], other clinical entities being studied for stem cell therapy include vascular disease, wounds, burns, hematological diseases, malignancies, Parkinson’s disease, Alzheimer’s disease, multiple sclerosis, spinal cord injury, diabetes, and other conditions [14]. Stem cells used in investigational studies could broadly be classified based on their differentiation potential: totipotent embryonic stem cells, adult multipotent stem cells, adult tissue specific stem cells, and ‘embryonic-like’ induced pluripotent stem cells. Both autologous (i.e., the patient’s own) and allogeneic (from a donor) cells are under investigation.
Stem Cells and Cardiac Regeneration
The potential for the human heart to regenerate constitutes a revolutionary paradigm shift in our understanding of myocardial pathophysiology; besides cellular hypertrophy, necrosis, apoptosis, and fibrosis, it appears that under the right conditions we can enable another myocardial response to injury – regeneration. While rate of cell turnover in the human heart is debated, the existence of the phenomenon is a) clearly established, and b) clearly inadequate to recoup the myocytes lost during myocardial infarction. One possible exception might be found in the syndrome of Anomalous Left Coronary Artery from the Pulmonary Artery (ALCAPA, or Bland-White-Garland syndrome); these infants have evidence of myocardial infarction, but if surgically corrected early may recover normal left ventricular function [15]. While the mechanism of this phenomenon is unknown, it seems likely that the regenerative potential of an infant’s myocardium might be substantially greater than that of adults’.
As a potential therapeutic intervention in adults, stem cell transplantation may be viewed as an attempt to augment the inadequate endogenous regenerative capacity of the heart, and to prevent the maladaptive sequelae. Mechanisms by which transplanted stem cells enhance cardiac regeneration include the formation of new cardiac myocytes, new blood vessels, modulation of inflammation, and attenuation of remodeling and fibrosis [16].
Stem Cells for Myocardial Disease
In this section we review broad categories of cell types under study for myocardial regeneration.
Skeletal Myoblasts
Skeletal myoblasts are stem cells located under the basal lamina in skeletal muscle; they were postulated to participate in repair following injury, and were among the first cells to be considered for myocardial regenerative therapy [17]. They can be obtained as an autologous product, have high scalability in culture, and appear to be resistant to ischemia. In animal models skeletal myoblasts appeared promising [18, 19]. Early phase clinical studies did encounter a safety concern and it was speculated that ventricular tachyarrhythmias might arise from failure of the graft electromechanically to couple with endogenous cardiomyocytes [20, 21].
Bone Marrow Derived Stem Cells
The adult bone marrow is home to a vast array of support cells and multipotent precursor lineage stem cells. Bone marrow derived stem cells (BMDSC) are the earliest and most investigated in all of stem cell research. In adult humans, BMDSC can be collected by iliac crest aspiration of marrow with expansion in culture, or obtained by cell sorting from peripheral blood after cytokine mobilization. BMDSC exhibit plasticity in vitro permitting differentiation into many cell types including those of cardiogenic lineage, such as contracting cardiomyocytes, coronary arterioles and capillaries [7]. BMDSCs appear to constitute many different cell types, and of these, preclinical studies [22] and clinical trials have examined several for their potential to promote favourable healing after myocardial infarction. Freshly prepared mononuclear cell fraction of BMDSCs have been the subject of the largest experience to date, but more specific fractions from the bone marrow include precursors of hematopoietic stem cells (HSCs), mesenchymal stem cells (MSCs) and endothelial progenitor cells (EPCs), which are found in varying quantities within the mononuclear population [23].
HSCs are negative for lineage markers (lin−) but express hematopoietic marker CD45 with Sca-1+, CD34+, CD133+ and c-kit+ (CD117) [24]. The transdifferentiation of HSC into cardiomyocytes and endothelial cells in the heart has been demonstrated in animal models. Experiments with the administration of c-kit+/lin− HSC in infarcted rat myocardium demonstrated significant cardiogenesis in conjunction with improved myocardial perfusion, angiogenesis and collateral vessel formation [25], translating into improved myocardial function, whether by transdifferentiation or another mechanism [26]. EPCs have the ability to differentiate into endothelial cells, and exist both in the bone marrow and in the peripheral circulation. EPCs share a common precursor with the HSCs and are CD34+ but are negative for CD45 [27]. Kawamoto et al. demonstrated engraftment, neovascularization and improved cardiac function with both intravenous and intramyocardial delivery of EPCs [28, 29].
MSC constitute a small percentage of the bone marrow; they express Stro-1, CD90, CD106, CD13 but no classic hematopoietic or endothelial cell markers [30, 31], and exhibit the capacity to differentiate into cells of several tissue types, including osteoblasts, chondroblasts and adipocytes. Our lab and others have demonstrated that bone marrow-derived MSCs transplanted into infarcted myocardium result in functional improvement with reduction of infarct scar area [32–34]. MSC also possess immunomodulatory characteristics, inhibiting immune responses during allogeneic transplantation in humans [35]. The use of allograft MSCs in animal models and in human subjects has followed; graft rejection has not been observed, and this approach reduced scar size and improved cardiac function in ischemic cardiomyopathy (ICM) [16, 36, 37].
Cardiac Stem Cells
Cells obtained from the heart with the Lin−/c-kit+ phenotype appear to have the potential to differentiate into functional cardiac myocytes, smooth muscle cells, and endothelial cells in vitro; these CSCs have been applied in animal models and in human subjects with the expectation of forming differentiated cardiac myocytes in vivo. CSC harvested from the adult heart (for example, from the right atrial appendage normally incised during many open heart surgeries) also exhibit other markers including Sca-1+ cells [38], side population (SP) cells [39], and ISL-1+ cells. Sca1-positive cells constitute 0.3 % of the myocyte compartment, and appear to have the potential for expressing cardiac transcription factors and transdifferentiating into cardiac myocytes in vivo [40]. Similarly, cellular and animal studies of Sca-1+/CD31cells [41], and SP cells [42] demonstrate cardiomyogenic potential. Together with Islet-1+ and c-kit+ CSCs , these and perhaps other cardiac stem cells exist in niches [43] from which proliferation, migration and regeneration may occur.
Reconstitution of the cardiac stem cell niche has been proposed to underlie the potential efficacy of “cardiospheres,” biologically engineered cultured multicellular structures containing a core of c-kit+ with supporting cells [44, 45], and cells derived from cultured cardiospheres have also advanced through the translational pathway [46].
Pluripotent Stem Cells
Pluripotent stem cells are those which may generate all three germ layers (ectoderm, mesoderm, and endoderm) and which self-renew indefinitely; these include embryonic stem cells (ESC) obtained from the developing embryo. ESC also have the potential for cardiac differentiation [47], but development of these cell types for therapy has been limited compared to other cells. Despite mechanistically feasible findings, both ethical issues and the potential of tumorgenicity [48] have led investigators to other approaches towards pluripotency, including nuclear reprogramming of differentiated adult cells [49, 50]. Like ESC, induced pluripotent stem cells (iPSC) have been shown in small animals to improve heart function after infarction [51].
Umbilical Cord Stem Cells
Human umbilical cord blood is a rich source of both HSC [52] and MSC [53] and these cells maintain proliferative potential which is neither embryonic nor adult, but probably in between [54]. Cord blood-derived cells have been used clinically for the treatment of hematologic diseases [55]. Transdifferentiation into cardiac myocytes and other lineages has been demonstrated [56], and transplantation into infarcted myocardium resulted in neoangiogenesis, reduced infarct size and improved ventricular function in animal models [57–59]. The availability of large quantities of cord blood may favour therapeutic development [60].
Adipose Derived Stem Cells
Paradoxically, as clinicians and epidemiologists bemoan the increasing trend towards obesity in industrialized societies, human adipose tissue also contains cells with multipotent potential [61]. Studies in vitro studies demonstrate ADSC can be persuaded to differentiate into cardiac myocyte-like cells [62, 63]. Showing similar characteristics as MSCs [64, 65], ADSC have been shown to regenerate damaged myocardium in animal studies [66], leading to translational studies with adipose tissue derived products in human subjects.
Cardiovascular Disease Targets for Stem Cell Therapy
Ischemic Cardiomyopathy
Most studies of cell therapy for myocardial disease have focused on that resulting from ischemic damage. Whether in the acute phase of myocardial infarction, or in the later stages of chronic ICM, this closely related set of disorders is well-understood after decades of clinical and preclinical investigation, and is particularly well-represented by small and large animal models [67]. Nonetheless, important non-ischemic heart diseases also have been subjected to translational investigation for stem cell therapies.
Non-ischemic Cardiomyopathies
In animal models, inherited non-ischemic cardiomyopathy [68–70], anthracycline chemotherapy-associated cardiomyopathy [71, 72], post-myocarditis cardiomyopathy [73, 74], and pacing tachycardia-induced cardiomyopathy [75] have been studied as potential targets for cell therapies. While clinical investigation and trials in these non-ischemic conditions remain fewer than those for acute and chronic ischemic myocardial diseases, translation into early phase human investigation for non-ischemic cardiomyopathies has begun.
Regenerative Mechanisms of Stem Cells
The hope that stem cells could replace damaged heart tissue [19, 22] by transdifferentiation into functioning cardiac myocytes and vascular cells (akin to re-populating a bare patch of lawn with grass seed) has proved a misleading oversimplification. Transdifferentiation may occur as a low-frequency event, but is unlikely to explain the magnitude and temporal course of myocardial regeneration; instead, the modulation and amplifaction of endogenous processes by the administered cell product appears to represent a major mechanism of action [76–78]. In a porcine myocardial infarct model, the administration of bone marrow derived MSCs resulted in 20-fold increase in endogenous c-kit+ CSCs; this principle was further supported by the finding that co-culture with MSCs enriched cardiopoetic cells obtained from heart biopsies [33]. Based on this finding of cell-cell interaction between niche-modulating cells and cardiotypic cells, co-administration of MSCs and CSCs in porcine chronic ICM was twice as effective as either cell type alone [79].
Cytokines which may be involved in this effect include vascular endothelial growth factor (VEGF), basic fibroblast growth factor (bFGF), insulin growth factor 1 (IGF-1), thymosin β4 (TB4), and stromal cell-derived factors 1 (SDF-1) [80], and it is likely that these cytokines are expressed with some temporal and spatial specificity including autocrine feedback, to modulate a local stem cell niche, influencing endogenous stem cells for proliferation, differentiation, cardiac remodelling and repair [81, 82]. Other paracrine effects may alter the metabolism [83] and recruitable contractility [84] of viable cardiac myocytes, and fusion of host cells with transplanted stem cells may occur, with the resulting cell exhibiting some of the characteristics of each contributor [85].
Techniques of Stem Cell Delivery
Cell administration in small animal models typically involved direct myocardial injection of cell suspensions through the epicardium. Large animal preclinical studies opened additional options, most of which have been applied in human subjects, including intracoronary infusion (either continuous or by stopping flow with an occlusive balloon), transendocardial injection (with purpose-built devices), transepicardial injection (typically adjunctive to open heart surgery), retrograde coronary perfusion, and intravenous administration. Each approach has its own logistical and technical advantages and disadvantages [86], and as clinical trials progress the outcomes related to the delivery technique may influence results as much as does the cell product [87].
Intracoronary administration has been frequently used following acute myocardial infarction reperfusion. If the target vessel is patent, the injured territory can accurately be targeted by infusing the culprit coronary, but engraftment will require cells to transmigrate across the endothelial lining, homing to sites of injured myocardium [88]. The potential for cell products to occlude the microvascular space is more than a theoretical concern [89].
Intramyocardial injection, by either transepicardial or transendocardial approaches, avoids these limitations, but increases the complexity of the cell administration procedure. Cells can be delivered directly to injured (or bordering) myocardium, without depending on the uncertainty of coronary transmigration. The spatial accuracy of these techniques has allowed subsequent analyses of the local influences of cells, revascularization, or the combination [90]. Transendocardial stem cell injection (TESI) into the border of an infarct zone appears to confer special benefit with concordant reduction in scar size and improvement in local contractility [91], so it makes sense for approaches to targeting the injection to be incorporated into trial designs [92]. Integration of CT or MR imaging of an infarction with ventriculographic assessment of wall motion permits directed delivery using fluoroscopy as the only real-time modality. Simultaneous biplane fluoroscopy is particularly advantageous when navigating a catheter in the LV chamber. Three-dimensional electromechanical mapping (EMM) [93] adds additional detail to the real-time assessment of potential targets for TESI, providing spatial assessment of myocardial viability and contractility [94].
Clinical Trials
Comprehensive reviews of clinical trials in the field are regularly published and updated [98, 99], and this chapter will not duplicate those efforts. In order that this chapter might be of more than transient relevance before becoming out of date, we have attempted here to connect the principles of cell biology, cardiac pathophysiology, and clinical trials design for only some of the more than 100 studies conducted or ongoing.
Acute Myocardial Infarction
Cell therapy studies for acute MI have included more than 1000 patients, mostly receiving BMDSC via intracoronary infusion. Results are mixed. For example, two studies can be compared. In a well-conducted, trial enrolling 101 patients with a first, anterior MI and receiving reperfusion by PCI, Lunde et al. randomized patients to receive bone marrow mononuclear cells (median cell number 68 × 106) by intracoronary infusion with stop-flow balloon occlusion. Control patients were not subjected to sham/placebo interventions. At 6 month cardiac MRI, no benefit was detected from cell infusion [100].
In contrast, the REPAIR-AMI trial randomized 204 patients with an acute MI to receive a ~200 × 106 bone marrow mononuclear cells or placebo 3–7 days after initial coronary intervention, again by stop flow intracoronary infusion. In this case, both LV ejection fraction changes and clinical endpoints seemed to be improved by cell therapy [101].
Among the many potential confounders for these trials, a few might be particularly significant. Important variability exists even among patients meeting the most tightly controlled inclusion criteria; differences in the severity and extent of coronary disease, pre-infarction angina, time to treatment, and others will influence the extent of myocardial injury, the efficacy of its salvage, the rate of recovery of myocardial contractility after reperfusion, and the net effect on global LV function. Ejection fraction, though undoubtedly a powerful marker of future clinical risk, is determined by the estimation of end-diastolic LV volume and end-systolic volume; it may vary substantially both on a beat to beat basis and as loading conditions change. Meta-analyses have been attempted and suggest that bone marrow cell infusion after acute MI may result in improved LVEF, reduced left ventricular end systolic volume (LVESV), and reduce infarct size [102]. Trends suggesting greater benefit in patients with more severely reduced EF are seen in these and other [103] trials of cell therapy for acute MI, including studies based on intravenous administration [104]. However, only large trials, such as the ongoing BAMI (The Effect of Intracoronary Reinfusion of BM-MNC on All Cause Mortality in Acute Myocardial Infarction) trial can approach balancing all these variables.
Chronic Ischemic Cardiomyopathy
In comparison with trials for acute MI, those enrolling patients with ICM include a broader range of cell types and delivery strategies. There is also significant heterogeneity among the outcome measures studied and the techniques used to characterize changes after cell therapy. Cardiac MRI is particularly useful in this regard, allowing quantification of both global and regional myocardial function, and measurement of the mass of viable myocardium and scar. While MRI remains the most complete technique, CT now approaches MRI in many of these measures. Many patients with ICM have implanted pacemakers and defibrillators, which create artifacts partially obscuring the heart with either MRI or CT. SPECT, PET, and echocardiography have been used as well.
Clinical assessments included in some trials have included the highly subjective (NYHA functional class) and more quantitative (MVO2 max) measures. The potential for placebo effect is particularly great when highly motivated patients with serious conditions undergo procedures with novel therapies [105], reinforcing the value of randomized blinded placebo controlled trials in this arena.
Bone Marrow-Derived Cells for Ischemic Cardiomyopathy
A number of cell products have been derived from adult bone marrow for preclinical and clinical testing. Bone marrow includes a heterogeneous population of cell types, among which there may be significant overlapping features. Whether the study product was a fresh preparation of bone marrow, was characterized by selecting from bone marrow cells for specific surface markers, or was culture expanded, clinical trials of bone marrow-derived cells for chronic ICM are reviewed here.
In 2003 Perin and colleagues published a prospective, nonrandomized, open-label safety and feasibility study of bone marrow derived CD34 marker (+) cells delivered by transendocardial injection, in 14 patients with severe LV dysfunction and ICM versus seven controls [106]. They reported that cell injection was associated with reduction in myocardial ischemia, improvement in global left ventricular function, and mechanical improvement of the injected segments as assessed by single-photon emission computed tomography (SPECT) analysis and EMM respectively. Treated patients also had significant improvements in New York Heart Association (NYHA) functional capacity, Canadian Cardiovascular Society Angina Score (CCSAS), achieved metabolic equivalents (METs) and maximum oxygen consumption (VO2 max); results also supported the safety of transendocardial stem cell delivery with the NOGA® system.
Randomized trials of bone marrow derived cells have not settled the question of whether this approach is effective. The FOCUS-CCTRN trial [107] randomized 92 patients with ischemic heart disease and LVEF ≤ 0.45 to receive 100 × 106 autologous bone marrow mononuclear cells, or placebo by transendocardial injection. Injections were targeted to 15 sites of viable myocardium as assessed by NOGA® electromechanical mapping. At 6 months, echocardiographic LV volume and EF, exercise capacity, and SPECT perfusion were not different between groups.
Different endpoints were assessed when our group studied 65 patients with ICM and LVEF < 0.50. The TAC-HFT trial [108] compared autologous bone marrow mononuclear cells (n = 19) vs placebo (n = 10), and autologous culture-expanded bone marrow-derived mesenchymal stem cells (n = 19) vs placebo (n = 11). Cells were injected at ten sites encompassing an infarct scar as assessed by CT or MRI, and biplane left ventriculography, using the Biocardia Helical Infusion Catheter®, which differs from the NOGA Myostar® in important respects; lacking the electromechanical mapping function of the NOGA system, the Biocardia catheter is navigated to sites selected based on prior cardiac imaging and real-time fluoroscopy. Its needle tip is helical, as compared to the straight needle of the NOGA Myostar. In this study, the transendocardial injection of cultured mesenchymal stem cells was associated with decreasing scar, increasing viable myocardial mass, and improved quality of life and 6 min walk distance.
Again, whether discrepant results relate to different patient populations, different cell types, different delivery strategies, different endpoint measures and techniques, or to the vicissitudes of early phase clinical trials will only begin to be answered when additional trial results come available.
The transepicardial approach to cell delivery has also been studied in patients with ICM undergoing CABG [109, 110]. Patel randomized 20 patients with ICM to undergo off-pump CABG with direct injection of autologous CD34+ cells vs off-pump CABG alone [111]. The treatment group had greater improvement in LVEF compared to controls at 6 months. Stamm and colleagues randomized patients with chronic ICM to receive direct injection of freshly prepared autologous CD133+ bone marrow cells along with CABG (n = 20) vs CABG alone (control, n = 20), and reported improvement in LVEF and myocardial perfusion at 6 months in the cell therapy group [112].
As described above, the intracoronary delivery of cells for acute MI has been studied in a large number of patients, but this approach has also been used for delivery of bone marrow-derived cells in patients with ICM. Small feasibility studies [113–116] suggested this might be safe. Strauer et al. compared 191 patients with ICM who received autologous bone marrow derived mononuclear cells vs 200 patients who chose not to receive cell therapy. Efficacy findings were reported including improved ejection fraction and long-term survival after cell therapy, but the trial design limits certainty of conclusions [117].
Skeletal Myoblasts
Menasche and colleagues reported in 2001 the transfer of autologous skeletal myoblasts into the myocardium by transepicardial injection during CABG in a single patient with ICM [118]. Subsequent work by the same group conducted in a non-randomized open label fashion suggested improvement in regional systolic contractility, but with the new onset of sustained ventricular tachycardia [17]. When randomized placebo-controlled design was employed for 97 patients undergoing coronary bypass with transfer of skeletal myoblasts (400 × 106 or 800 × 106 million) or placebo, there was no improvement in regional or global LV contractility associated with cell treatment; those receiving myoblasts had more arrhythmia events, though total major adverse cardiac events did not differ among groups [119].
Dib administered skeletal myoblasts via transendocardial injection in 12 patients with ICM in an open label unblinded randomized fashion, and compared to 11 control subjects [120]. This trial reported improvement in NYHA class and quality of life with myoblasts; left ventricular volume changes were not statistically different between groups. Arrhythmia events were not increased with cell treatment. While the meaning of changes in serial electromechanical mapping are not known, it is interesting that 3 month follow-up mapping in the patients treated with cells showed increased unipolar electrocardiographic voltage in the treated segments and the hearts as a whole, compared with baseline.
Cardiac Stem Cells
In the SCIPIO trial, autologous cardiac stem cells identified by the c-kit+ marker were obtained from the right atrial appendage of patients undergoing coronary bypass surgery, were expanded in culture, then were returned to the patient a mean of 113 days later. One-half million to one million cells were administered by intracoronary infusion with a stop-flow technique into an infarct territory in a randomized, open-label trial of 16 patients. Among nine patients who had baseline and 4 month cardiac MRI scans, the ejection fraction increased significantly from 27.5 % at baseline (i.e., 4 months after CABG, and before CSC infusion), to 35.1 % after 4 months and to 41.2 % after 12 months, and this seemed to correlate with improved NYHA class and quality of life [121].
Cardiosphere-Derived Cells
In the CADUCEUS trial, heart tissue was obtained by endomyocardial biopsy, a minimally invasive transcatheter procedure. Cardiospheres were prepared by culture from this material, and dispersed cells from these structures were returned to the patient by intracoronary infusion. One important difference from some other ischemic cardiomyopathy trials is that these patients had very recent infarctions; they were enrolled 2–4 weeks after acute MI and were treated (n = 17) 1 ½–3 months after infarction in a randomized 2:1 scheme against control treatment (n = 8). Six months after treatment, MRI showed reduced scar mass, increased viable myocardium, and improved regional contractility. Chamber volume and EF did not differ between groups. Similar findings were reported after 12 month MRI [122].
Non-Ischemic Cardiomyopathy
While the myocardial disease related to coronary ischemia has been the subject of much more preclinical, translational, and clinical study, other forms of cardiomyopathy are important and have motivated investigation as well. Idiopathic dilated cardiomyopathy (DCM), and specific entities such as familial cardiomyopathies or chemotherapy-associated cardiomyopathy are beginning to be studied with the hope that cell therapy may regenerate or repair damaged myocardium.
Arguero et al. conducted the first clinical trial including bone marrow-derived cells delivery by surgical transepicardial injection, including in five patients with DCM [123]. Similarly, Arom et al. administered peripherally circulating cells by thoracoscopic injection in 20 patients with ischemic cardiomyopathy and 21 patients with DCM [124]. Both studies reported improved LVEF and functional status.
Fischer-Rasokat et al. conducted a study in 33 patients with DCM using an intracoronary infusion of BMDSC. After 3 months, cardiac function improved significantly and at 12 months N-terminal prohormone brain natriuretic peptide (NT-proBNP) serum levels were also decreased [125]. Improvements in quality of life, clinical symptoms, exercise capacity and cardiac function were reported in a similar study conducted by Martino et al. [126].
In the first randomized, open label, controlled trial performed by Seth et al., patients with DCM (n = 24) were administered autologous bone marrow mononuclear cells, and compared to 20 control patients. Cell administration was by intracoronary infusion, during balloon occlusion of the coronary sinus effluent. At 6 months, the treatment group demonstrated significant improvements in the LVEF, NYHA scale, and cardiac volumes compared to controls [127]. A later report from the same group continued to show improved cardiac function and clinical symptoms with treatment (n = 41) compared to control (n = 40) [128].
Vrtovec has reported the long-term follow-up of 110 patients = DCM who were randomized to receive open-label intracoronary infusion of CD34+ cells obtained by GM-CSF mobilization and apheresis, or control. LVEF increased up to 3 years, after which improvement declined, but at 5 years, the patients receiving cells still had higher LVEF and 6-min walk distance, and lower N-terminal B-type natriuretic peptide (a blood biomarker of heart failure.) [129].
Taken in context, these results do suggest that the treatment of non-ischemic cardiomyopathies is feasible and can be considered; randomized blinded placebo controlled trials will be required before firm conclusions can be drawn about efficacy.
Autologous Vs Allogeneic
As surveyed herein, a variety of autologous cell products have been subjected to early stage testing with a variety of strategies. While autologous cells would be expected to avoid the potential of host rejection, compared with the possibility of donor-derived allogeneic products, autologous cells do have important practical and clinical limitations. Depending on the cell type being prepared, tissue acquisition and processing, and culture expansion impose logistical obstacles to treatment when it might be needed. Furthermore, it has been suggested that stem cells from older, sicker patients are marked by smaller number or less potency [130]. Finally, the requirement to prepare (and perform pre-release quality testing for) a cell product for each individual patient is an expensive undertaking.
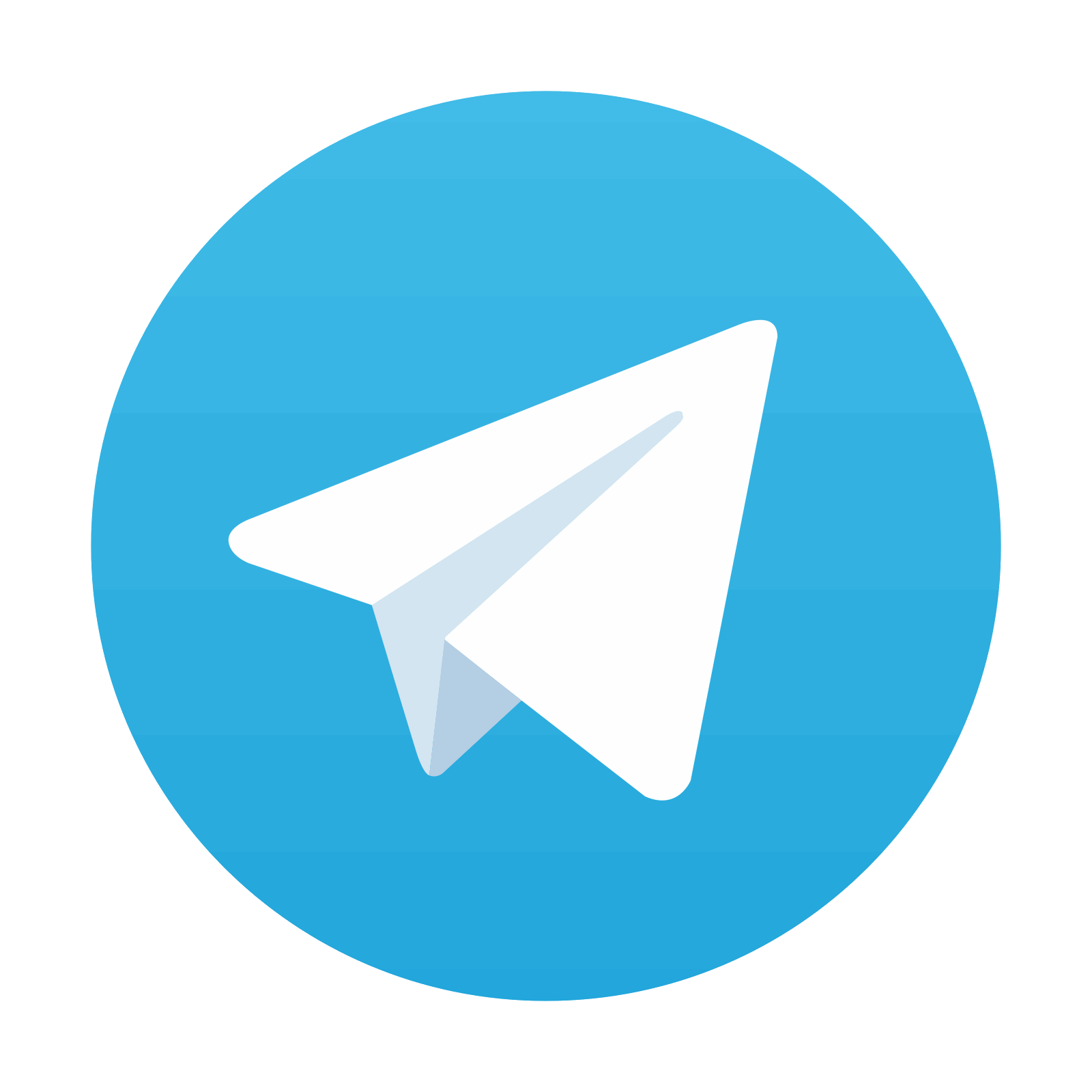
Stay updated, free articles. Join our Telegram channel

Full access? Get Clinical Tree
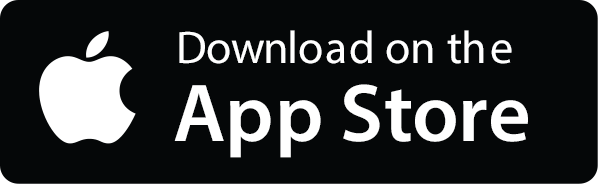
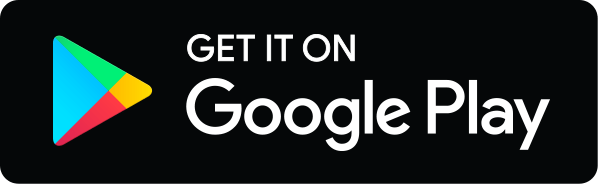