Fig. 3.1
Illustration of potential stem/progenitor cell niches in the lung of the adult mouse. The lung can be divided into three major levels of conducting airways (the trachea, bronchi, and bronchioles) plus the gas-exchanging alveoli. Distinct region-specific stem/progenitor cell niches are thought to exist along the proximal-distal axis of the airway. These include: SMG ducts in the proximal trachea, basal cells within intercartilaginous zones of the trachea and primary bronchi, NEBs in the intralobar bronchi and bronchioles, and the BADJ and alveolar spaces within the alveoli. Progenitor/stem cells (marked in red and listed) reside in their respective local niches and these environments enable them to maintain their stem/progenitor properties and control their ability to differentiate into various progeny cell types. SMG submucosal gland, NEB neuroepithelial body, BADJ bronchioalveolar duct junction, BV blood vessel
Studies using murine models have revealed several region-specific stem cell niches along the proximal-distal axis of the airway that maintain distinct subpopulations of progenitors. Stem/progenitor cells are mobilized from these epithelial niches to maintain tissue homeostasis during injury repair and normal cellular turnover. The coordination of molecular and cellular events in the microenvironment of stem cell niches plays a pivotal role in maintaining the balance of stem/progenitors and differentiated cells that are needed for regeneration in the lung (Fig. 3.1). In this chapter, we review the diversity of cell types, including potential stem/progenitor cells, that have been identified in the adult lung, and discuss advances in our understanding of stem/progenitor cell niches and their roles in injury repair and lung cancer.
3.2 Cellular Diversity in the Adult Lung
Based on its anatomical and functional features, the lung epithelium can be divided into three domains: the proximal cartilaginous airways (trachea and bronchi), the bronchioles (bronchioles, terminal bronchioles, and respiratory bronchioles), and the alveoli. The epithelial cell types in each of these domains are distinguished by their morphology, cellular phenotype (i.e., proteins they express), and function. The proximal airway of the mouse is lined with a pseudostratified columnar epithelium composed mainly of basal, club, goblet, and ciliated cells; the secretory SMGs reside beneath this surface airway epithelium (SAE) and are limited to the proximal trachea in mice (Hansell and Moretti 1969; Pack et al. 1980; Widdicombe et al. 2001; Jeffery 1983; Liu et al. 2006). The major cell types in the human proximal airway differ slightly from those in mice and include basal, intermediate, goblet, non-ciliated columnar, and ciliated cells (Jeffery 1983; Liu et al. 2006; Mercer et al. 1994). Furthermore, in humans the SMGs are present throughout the cartilaginous airways, including the trachea and bronchi. These glands are composed of an interconnecting network of serous acini and mucus tubules, which secrete antibacterial factors, mucous, and fluid into the airway lumen (Wine and Joo 2004). In the distal mouse and human airways (i.e., bronchioles), club, ciliated, neuroendocrine, and goblet cells are the major cell types, and neuroendocrine cells are found both individually and in clusters within NEBs (Mercer et al. 1994; Van Lommel et al. 1999; Plopper et al. 1980; Liu et al. 2006). However, the bronchioles of human lungs have also been shown to contain basal cells, albeit at lower abundance than in the proximal regions (Tamai 1983; Rock et al. 2010). The alveolar epithelium is lined by surfactant-producing cuboidal alveolar type II epithelial cells (AECII) and squamous gas-exchanging alveolar type I epithelial cells (AECI) (Liu et al. 2006). The major epithelial cell types that are present at various locations throughout the airway are listed in Table 3.1.
Table 3.1
Major epithelial cell types in the lungs of adult mice and humans
Cell types | Cell-type markers | Stem/Progenitor cells | Lineage cell type(s) | Candidate niches | Reference(s) |
---|---|---|---|---|---|
Basal cells | Cytokeratin 5, Cytokeratin 14, P63, NGFR, ITGA6 | Yes | Basal, club, goblet, and ciliated cells | Intercartilaginous zones and SMGs | |
Intermediate cells | Cytokeratin 5, Cytokeratin 14, P63 | Yes | Goblet and ciliated cells | Undefined (transient amplifying progenitor) | |
Club cells (conducting airways) | CCSP (Scgb1a1) | Yes | Club, basala, goblet, and ciliated cells | NEB | |
Club cells (BADJ) | CCSP (Scgb1a1), SPC | Yes | Club, AECII, and AEC1 cells | BADJ | |
Ciliated cells | FoxJ1, Tubulin IV | No | Not applicable | Not applicable | |
Goblet cells | Mucin 5AC, Mucin 5B, Spdef | No | Not applicable | Not applicable | |
Non-ciliated columnar cells (non-goblet or non-club) | Undefined | Yes | Undefined | Undefined | Evans et al. (1986) |
AECI cells | Aquaporin 5, Podoplanin RAGE | No | Not applicable | Not applicable | |
AECII cells | SPB, SPC, Lamp3, Abca3, and integrin α6β4 | Yes | ATI and ATII cells | Alveoli space | |
PNEC | CGRP, Ascl1, Pgp9.5 | Yes | PNEC, clubb, and ciliated cellsb | NEB | |
MSC | CD73/90/105, Vimentin, Prolys-4-hydroxylase | Yes | Adipocyte, chondrocyte, osteocyte, myofibroblasts, and fibroblasts | Bone marrow, circulation system, and pulmonary interstitium |
3.3 Potential Stem Cells in the Adult Lung
Stem/progenitor cells are crucial for development, tissue homeostasis, and injury repair in the lung. Studies using epithelial reconstitution assays, murine injury models, and lineage tracing approaches have identified several region-specific stem/progenitor cell populations in the adult lung of mice and humans. Basal cells in the proximal airways, variant club cells in bronchioles, bronchoalveolar stem cells (BASCs) in BADJs, and a subset of AECII in alveolar spaces have all been identified as stem/progenitor cells (Table 3.1).
In the trachea and main-stem bronchi, basal cells are the principal stem cells involved in homeostasis and injury repair and have the capacity to generate all the major cell types found in the proximal airway, including basal, ciliated, goblet, and granular secretory cells (including club cells) (Hong et al. 2004a, b; Hajj et al. 2007; Rock et al. 2009; Schoch et al. 2004; Cole et al. 2010; Engelhardt et al. 1995). The intermediate cells in the human proximal airway are so named because they are generally thought to represent an intermediate state of differentiation from basal cells and to serve as a transient amplifying cell population with the capacity to differentiate into ciliated and goblet cells (Engelhardt et al. 1995; Mercer et al. 1994). Intermediate cells do not exist in the mouse proximal airway, potentially because of the less pseudostratified nature of their smaller diameter airways. Of note, studies of murine lung injury involving BrdU labeling demonstrated that label-retaining cells (LRCs) reside predominantly in the ducts of SMGs, suggesting that these glands serve as a stem cell niche in the proximal airway (Xie et al. 2011; Borthwick et al. 2001; Engelhardt et al. 1995; Engelhardt 2001; Rock et al. 2009). Importantly, the SMG-localized LRCs have the capacity to undergo sequential rounds of cell division despite their slowly cycling phenotype (Xie et al. 2011; Lynch and Engelhardt 2014). Nevertheless, because lineage tracing of glandular LRCs has not yet been possible, the ability of these stem cells to produce specific airway cell types remains unclear. Several cellular markers have been utilized to identify and isolate basal cells. These include cytokeratin 5 (CK5), cytokeratin 14 (CK14), and aquaporin 3 (Rock et al. 2009, 2010; Schoch et al. 2004). Using a CK5-CreERT2 transgenic mouse line, Rock et al. further demonstrated that basal cells are capable of differentiating into club and ciliated cells, both at steady state and during injury repair (Rock et al. 2009). In addition, they identified nerve growth factor receptor (NGFR) and integrin α6 (ITGA6, also called CD49f) as markers on the surfaces of isolated human basal stem cells (Rock et al. 2009). Similarly, Ghosh et al. identified a CD49fbright/Sca-1+/ALDH1+ (Aldehyde dehydrogenase 1) subset of tracheal basal cells as region-specific stem cells, and demonstrated that these cells could generate niches in vitro and contribute to tracheal epithelial maintenance and injury repair (Ghosh et al. 2011). These studies suggested that basal cells play key roles in both homeostasis and injury repair of the proximal airway.
In the intralobar bronchiolar airways, a subset of the variant club cells that express club cell secretory protein (CCSP, also called Scgb1a1) but not CyP450-2F2 (CCSP+, CyP450-2F2−) can self-renew and produce both club cells and ciliated cells (Hong et al. 2001; Rawlins et al. 2009b; Reynolds and Malkinson 2010; Xing et al. 2012; Guha et al. 2012). This CCSP+/CyP450-2F2− subset was also found at the BADJ of distal bronchioles, where it contributed to airway epithelial regeneration following naphthalene-mediated depletion of CyP450-2F2+ club cells (Giangreco et al. 2002). Kim et al. subsequently identified BASCs as a subpopulation of cells that express CCSP and pro-surfactant protein C (SPC) and serve as region-specific stem cell at the BADJ (Kim et al. 2005). Using naphthalene- and bleomycin-induced murine models of lung injury repair, Kim et al. further demonstrated that these cells possessed the capacity to self-renew and to produce differentiated epithelial cells in vivo, and that BASCs could differentiate into club cells and alveolar epithelial cells in an ex vivo clonogenic assay (Kim et al. 2005). Conversely, an in vivo lineage tracing experiment using a CCSP(Scgb1a1)-CreER™ knock-in mouse line revealed that club cells generated daughter club cells and ciliated cells but not alveolar cells following hypoxia-induced lung injury (Rawlins et al. 2009b). However, subsequent lineage tracing studies following alveolar injury by influenza infection and bleomycin exposure support the finding that CCSP-expressing stem/progenitors can give rise to AECI and AECII cells (Zheng et al. 2013; Rock et al. 2011). These injury-dependent influences on BASC-derived lineages suggest that either specific injury signals may invoke different responses and/or that multiple subsets of BASCs exist with different capacities for differentiation. The later hypothesis is consistent with findings suggesting that BASCs in the distal airways might include a heterogeneous population of progenitor cells (Teisanu et al. 2009, 2011; Chen et al. 2012). A study by Teisanu et al. classified club cells with the surface antigen profile CD45−/CD31−/CD34−/EpCAM+/Sca-1low into two subgroups based on their autofluorescence (AF) profiles and suggested that club cells in the AFlow population are naphthalene resistant, whereas their AFhigh counterparts were not (Teisanu et al. 2011). Indeed, mice that were exposed to naphthalene showed significantly greater proliferation in AFlow club cells compared to AFhigh club cells, and conversely, mice exposed to ozone showed significantly greater proliferation in the AFhigh club cell fraction compared to AFlow club cells (Teisanu et al. 2011). McQualter et al. demonstrated that an EpCAM+/Sca-1low/Integrin α6β4+/CD24low fraction of epithelial stem/progenitor cells was capable of self-renewing and differentiating into a variety of airway epithelial lineages, including alveolar epithelial cells (McQualter and Bertoncello 2012). These studies provide evidence that BASCs play key roles in the repair of injury to both bronchiolar and alveolar cells, as well as in homeostasis.
In the pulmonary alveolus, surfactant-producing AECII cells have long been recognized as stem/progenitor cells for the squamous AECI cells in the adult lung (Adamson and Bowden 1974; Evans et al. 1975). In vitro assays of cell proliferation and clonogenicity, as well as in vivo analyses following epithelial injury and lineage tracing, have produced mounting evidence that a subset of AECIIs have the capacity to proliferate and restore the alveolar epithelium by producing either new AECII cells or their squamous AECI counterparts (Reddy et al. 2004; Barkauskas et al. 2013; Fujino et al. 2011). Equally noteworthy were findings suggesting that integrin α6β4 is a biomarker for a subset of stem/progenitor cells in alveolar epithelia; SPC−/integrin α6β4+ cells were found resident in the alveolar epithelia, where they were able to regenerate SPC+ AECII cells (Chapman et al. 2011). The notion that AECII cells are region-specific stem/progenitors was recently confirmed by work from the Hogan laboratory, which employed a genetic SPC-labeled lineage tracing assay and an in vitro 3D culture model. This study produced convincing evidence that AECII cells were able to maintain the homeostasis of alveolar epithelia during both steady-state turnover and injury repair (Barkauskas et al. 2013). Several strategies that rely on biomarkers to identify and isolate adult lung stem/progenitor cells are listed in Table 3.2.
Table 3.2
Experimental strategies used to isolate and characterize progenitor cells based on phenotypic characteristics
Input | Phenotypic markersa,b | Assay | Differentiation/Proliferation potential | References |
---|---|---|---|---|
Human nasal polyps | CD151+/F3+ | In vitro ALI, ex vivo xenograft assays | Fully differentiated and functional airway epithelium including basal, columnar, goblet, and ciliated cells | Hajj et al. (2007) |
Human fetal airway xenografts | AQP3− | Ex vivo xenograft assay | Mature epithelia including ciliated, secretory, and basal cells within 4–6 weeks (compared to 6–20 weeks in AQP3hi cells) and by 20 weeks both AQP3− and AQP3hi cells generated mature epithelia including glands containing mucous and secretory cells | Avril-Delplanque et al. (2005) |
Human trachea | NGFR+/ITGA6+ | In vitro 3D colony-forming assay | Colonies containing basal and ciliated cells | Rock et al. (2009) |
Human trachea and bronchi | CD45−/H33342Side Pop | In vitro limiting dilution assay, ALI culture | Cobblestone colonies of basal cells on plastic and basal, columnar, and goblet cells in ALI cultures | Hackett et al. (2008) |
Human lung | CD45−/CD31−/CD73−/CD34−/CDH1+/LGR6+ | In vitro limiting dilution assay, in vivo kidney organoid assay | Colonies containing club, AECII, and AECI cells and single cells from both freshly isolated and clonally expanded cells generated clones in mouse kidney capsule grafts | Oeztuerk-Winder et al. (2012) |
Human lung | CD45−/CD31−/EpCAM+/HTII-280+ | In vitro 3D colony-forming assay | Colonies containing AECII cells | Barkauskas et al. (2013) |
Mouse trachea | GSI-A3B+/NGFR+ | In vitro 3D colony-forming assay | Colonies containing basal and ciliated cells | Rock et al. (2009) |
Mouse trachea | CD45−/CD31−/Ly76−/Ly6a+/Itga6hi/Aldefluor+ | In vitro colony forming assay(s) in vivo airway injury model | Rimmed clones in a co-culture system with irradiated feeder cells; basal, ciliated, and club cells in a mixed ALI culture system; proliferated in vivo following naphthalene injury | Ghosh et al. (2011) |
Mouse tracheal surface epithelium | Trop2+/Itga6+/Aldefluor+ | In vitro 3D colony-forming assay | Luminal colonies containing basal, ciliated, columnar, serous, and mucous cells in 3D cultures | |
Mouse tracheal glandular epithelium | Trop2+/Itga6+/Aldefluor+ | In vitro 3D colony-forming assay, in vivo fat pad assay | Dense colonies containing basal, columnar, serous, and mucous cells in 3D cultures, and basal, serous, mucous, and myoepithelial cells in an in vivo fat pad assay | |
Mouse lung | CD45−/CD31−/EpCAM+/Itgb4+/LysoTracker+ | In vitro 3D colony-forming assay | Colonies containing AECII cells | Van der Velden et al. (2013) |
Mouse lung | CD45−/CD16−/CD32−/EpCAMhi/Itgb4+ | In vitro 3D colony-forming assay, in vivo kidney organoid assay | Colonies containing AECII, club, and cell expressing both SPC and CCSP in a 3D colony-forming assay and within a mixed kidney capsule organiod assay | Chapman et al. (2011) |
Mouse lung | CD45−/CD31−/Ly6a+/CD34+ | In vitro limiting dilution assay | Mesenchymal colonies with osteogenic and chondrogenic potential (data not shown) | McQualter et al. (2009) |
Mouse lung | CD45−/CD31−/EpCAMhi/Itga6+/Itgb4+/CD24lo | in vitro 3D colony-forming assay | Cystic colonies containing ciliated, club, goblet, basal, and secretory cells, saccular colonies containing club, AECII cells, and mixed colonies containing mucous, ciliated, and AECII cells | McQualter et al. (2010) |
Transgenic mouse trachea | CK5-GFP+/GSI-A3B+ | In vitro 3D colony-forming assay, in vivo lineage tracing | Colonies containing ciliated and basal cells; lineage-labeled CK5-expressing cells generated basal, club, and ciliated cells both at steady state and following SO2 injury | Rock et al. (2009) |
Transgenic mouse lung | CD45−/CD31−/CD34−/EpCAM+/SFTPC-GFP−/lo/hi | In vitro 3D colony-forming assay | GFPneg cells generated spheroid colonies containing AECII, club, serous, ciliated, and basal cells, GFPlo cells generated irregularly shaped colonies containing AECII, club, and ciliated cells, and GFPhi cells generated dense colonies containing AECII cells | Chen et al. (2012) |
Transgenic mouse lung | CD45−/CD31−/EpCAM+/Sftpc-CreER; Rosa-Tm+ | In vitro 3D colony-forming assay, in vivo lineage tracing | Colonies containing AECII and AECI cells; lineage-labeled Sftpc-expressing cells proliferate at steady state and following injury | Barkauskas et al. (2013) |
3.4 Stem Cell Niches in the Adult Lung
As discussed above, a vast body of evidence has demonstrated that distinct stem/progenitor cell populations reside in specific anatomical niches (Fig. 3.1), where diverse cell types and signals coordinate the behavior of stem cells during homeostasis and following injury. Stem cell niches are discrete microenvironmental units within a tissue that can provide one or more of the following features important for stem cell control: a unique extracellular matrix; supporting cell types; unique innervation and nearby vasculature; and diffusible factors that allow stem cells to maintain a capacity to self-renewal and control their proliferation and differentiation in the setting of injury (Fuchs et al. 2004). The anatomical sites of airway stem cell niches are typically epithelial structures associated with these unique features described above (e.g., innervation, support cells). Although much remains to be learned about how components of airway niches coordinate stem/progenitor cell behavior and phenotype, data from organ systems that have been studied more extensively suggest that they are likely important in the lung as well.
In the following discussion of stem cell niches in the airway, we focus on the unique anatomic and biologic properties of each niche within a particular region of the lung, and on how these features may contribute to repair following injury. In particular, we concentrate on studies of slowly cycling stem/progenitor cells in the mouse lung, since nucleotide label retention has been one of the most commonly used methods for tracking the anatomic locations of stem cell niches in the lung.
3.4.1 The Tracheal Surface Airway Epithelium
Within the tracheal SAE, subsets of basal cells are thought to be the major stem/progenitor cells. Following injury, LRCs tend to cluster within intercartilaginous zones of the distal trachea and larger bronchi along the basal lamina of the surface epithelium (Borthwick et al. 2001). These intercartilaginous zones tend to be sites of high blood vessel concentration and nerve penetration to the epithelium (Baker et al. 1986; McDonald 1988). These features are likely important biologic components and may function to localize stem/progenitor cells within this niche at homeostasis; they could also mediate injury responses that direct changes in stem/progenitor cell behavior. It has been suggested that these intercartilaginous zones enable a subset of surface basal cells to maintain multipotency within the mouse proximal airway (Borthwick et al. 2001; Engelhardt 2001; Liu and Engelhardt 2008; Rock et al. 2009, 2010; Hong et al. 2004b). Subpopulations of basal cells with the capacity for self-renewal and differentiation have also been described by others, based on clonogenic assays and lineage tracing studies (Cole et al. 2010; Hajj et al. 2007; Hong et al. 2004a; Schoch et al. 2004; Rock et al. 2009). For example, Rock et al. recently identified a subset of basal cells that were marked with p63+/NGFR+/CK5+ that were able to self-renew and to generate luminal daughter cells within an in vitro 3D tracheosphere assay (Rock et al. 2009). Lineage tracing studies in mice expressing a CK5-promoter driven CreER transgene further demonstrated that CK5-expressing basal cells could give rise to ciliated and club cells in the tracheobronchial airways, both at steady state and following injury (Rock and Hogan 2011; Rock et al. 2010).
3.4.2 The Tracheal Submucosal Glands
A link between SMGs and stem/progenitor cells in the SAE was first discovered through retroviral lineage tracing experiments using human airway epithelial cells and a rat trachea xenograft model (Engelhardt et al. 1995). In these studies, retrovirally tagged human tracheobronchial epithelial cells were expanded in a denuded rat trachea that had been subcutaneously implanted into nu/nu mice. These cultures contained diverse populations of airway cells that were capable of clonal expansion within the xenografted airway. Phenotypic analysis of clones established a working model for progenitor/progeny relationships in the adult human proximal airway. Although seven clonal classes were discovered, the most abundant clone phenotype was multipotent and contained basal, intermediate, ciliated, and goblet cells. These multipotent clones were also the largest in size, supporting the hypothesis that they were derived from stem/progenitors with the largest capacity for expansion. Notably, SMGs also formed within these xenografts, and lineage tracing revealed that they were always associated with multipotent clones on the SAE. Expansion of basal cell progenitors in vitro prior to seeding into xenografts reduced the complexity of possible outcomes in clone phenotypes observed, giving rise to multipotent clones almost exclusively. These findings suggested that a small subset of basal cells are multipotent for SAE cell types and also have the capacity to form SMGs (Engelhardt et al. 1995). Additionally, these studies demonstrated with early passage primary human airway epithelial cells that a diverse range of progenitors exist in the human proximal airway with unipotent and bipotent capacities for differentiation. Later, clonal analysis in mice expressing a CK14-CreER transgene confirmed these findings and demonstrated that at least two subsets of basal cells exist with either unipotent or multipotent capacity for differentiation (Hong et al. 2004b). Consistent with the finding that a subset of adult airway stem cells have the capacity to generate SMGs, LRCs localized within glands or glandular ducts following tracheal epithelial regeneration following injury (in response to both SO2 and detergent treatment), suggesting that a subset of slowly cycling glandular epithelial cells are tissue-specific stem/progenitor cells and are capable of regenerating the airway epithelium after injury (Borthwick et al. 2001; Engelhardt 2001). Based on dual nucleotide sequential labeling experiments, these glandular LRCs retain the capacity to divide following repeated injury but remain slowly cycling (Xie et al. 2011; Lynch and Engelhardt 2014). Glandular LRCs make up a small fraction of total glandular cells (0.39 % ± 0.03 %) at 90 days after injury and only about 10 % of glandular LRCs reenter the cell cycle following a second injury and remain slowly cycling (Xie et al. 2011). Thus, if slowly cycling glandular LRCs are a stem cell, they represent approximately 0.04 % of total glandular cells.
Similar observations on glandular-derived stem cells were made in a murine model of hypoxic-ischemic injury (Hegab et al. 2011, 2012b). In these studies, Hegab et al. found that the SMG duct cell population included stem/progenitor cells that shared phenotypic features with surface airway basal cells and were resistant to epithelial injury in the context of tracheal hypoxic-ischemic injury. In vitro and ex vivo assays carried out with epithelial stem/progenitor cells isolated from the SMG duct have demonstrated that these cells are capable of self-renew and can generate several cell types found in the SAE and SMGs (Hegab et al. 2011, 2012a, 2014). Furthermore, in vitro colony forming assays using epithelia isolated from the gland-rich proximal region of the mouse trachea have revealed that these cells have a higher potential for proliferation than their counterparts from the gland-free distal trachea (Xie et al. 2011).
Cumulatively, these studies provide convincing evidence that the SMGs serve as a stem/progenitor cell niche for the proximal airway. The positioning of stem/progenitor cell niches within SMGs likely has biologic significance beyond simply the maintenance of glandular cell types. For example, SMGs are less exposed to the external environment and pathogens that threaten the lung, thus glandular stem cell niches are more protected. Additionally, SMGs are highly innervated (Nadel 1983; Wine 2007) and their secretions are regulated (i.e., enhanced) in response to injury of the SAE (Xie et al. 2011). Given that SMGs play an important role in airway innate immunity by producing secretions that regulate the composition of fluid, electrolytes, mucus, and antibacterial factors at the airway surface (Wine and Joo 2004; Wang et al. 2001; Dajani et al. 2005), it is not surprising that the regulation of glandular secretions following airway insults might be coordinated with the mobilization of glandular stem/progenitor cells that regenerate the airway surface. Interestingly, studies of cystic fibrosis suggest that defects in glandular secretions caused by the lack of the cystic fibrosis transmembrane conductance regulator (CFTR) chloride channel alter the SMG stem/progenitor cell niche by dysregulating the calcitonin gene-related peptide (CGRP) neuropeptide (Xie et al. 2011). Such studies have demonstrated that, in mice deficient for CFTR, slowly cycling LRCs relocate from SMGs to the SAE following naphthalene injury and that this is accompanied by a redistribution of highly proliferative stem/progenitor cells from proximal gland-rich regions of the trachea to regions of the SAE that lack glands. CGRP is induced in SMGs following airway injury and leads to the induction of gland secretions by activating CFTR, however, CGRP is constitutively upregulated in SMGs of cystic fibrosis humans, ferrets, pigs, and mice and this altered neuroendocrine signaling is thought to be the basis of stem cell niche dysfunction (Xie et al. 2011). Such findings emphasize the plastic nature of airway stem/progenitor cell niches.
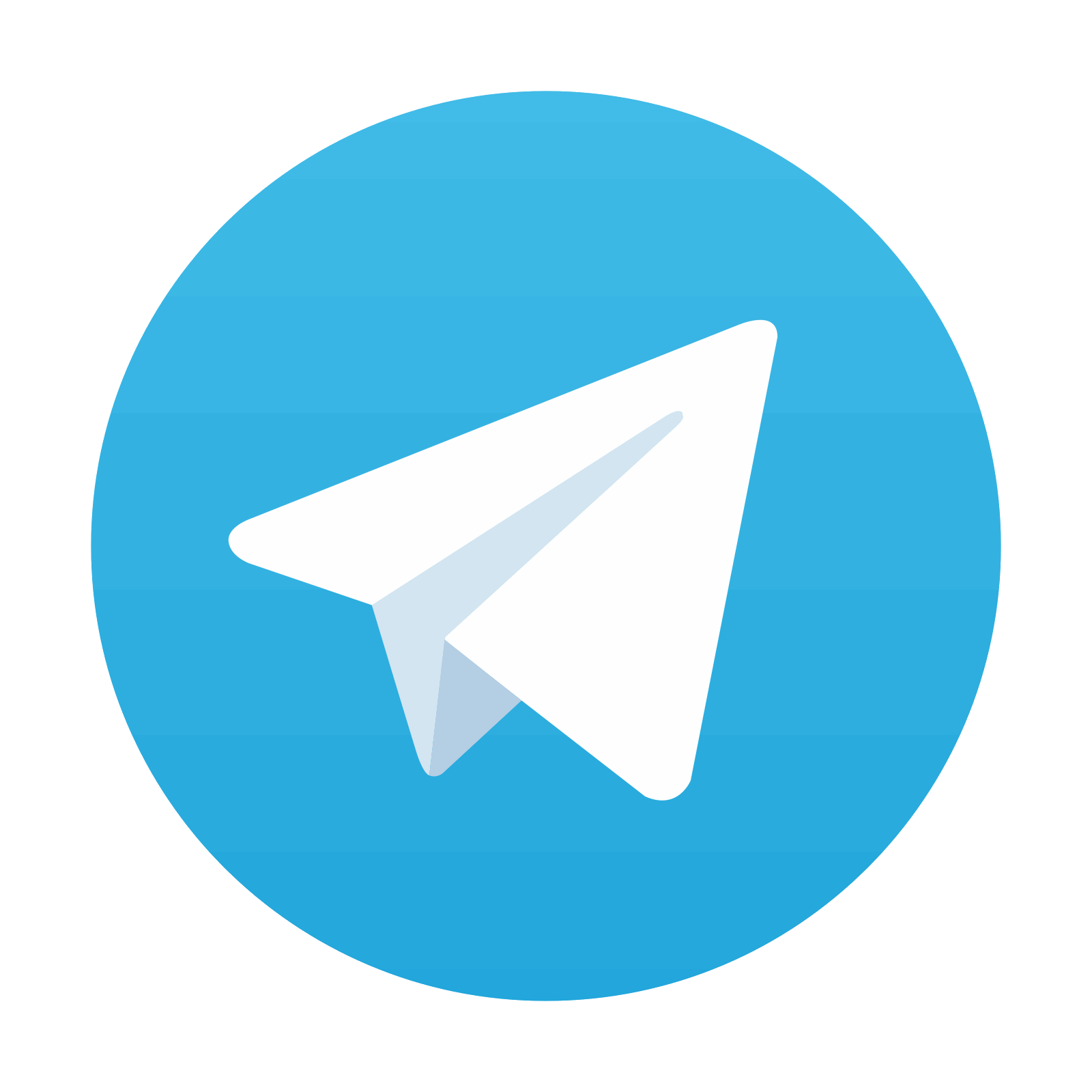
Stay updated, free articles. Join our Telegram channel

Full access? Get Clinical Tree
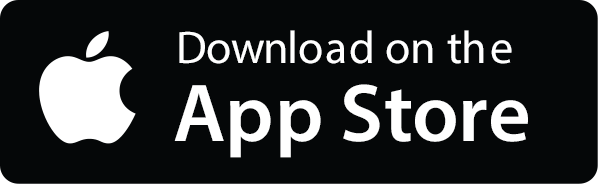
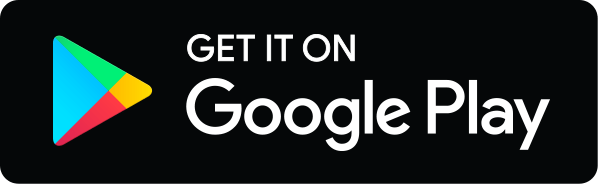