Outline
Cardiac Cell Therapy, 599
Mechanisms of Action for Cardiac Cell Therapy, 599
Clinical Trials of Cardiac Cell Therapy, 603
Bone Marrow–Derived Mononuclear Cells, 603
Bone Marrow–Derived Mesenchymal Stem Cells, 604
Cardiac Stem/Progenitor Cells, 604
Cardiosphere-Derived Cells, 604
Open Questions in Optimizing Cardiac Cell Therapy, 604
Gene Therapies in Heart Failure, 605
Gene Therapy Enhancing Contractility, 605
Gene Therapy Enhancing Stem Cell Homing, 606
Gene Therapy for Cardiac Regeneration, 606
Summary and Future Directions, 607
Cardiac Cell Therapy
Myocardial infarction (MI), despite significant advances in acute reperfusion and chronic pharmacotherapy, remains a major cause of heart failure in the United States. The lost myocardium is replaced by fibrotic scar leading to progressive left ventricular (LV) remodeling and further dysfunction. Although limited myocyte turnover in the adult mammalian heart has been reported to occur at baseline, this intrinsic regenerative capacity is limited and inadequate to restore function following injury. Thus healing following loss of myocardium is achieved through myocyte hypertrophy and scar formation. In contrast, lower vertebrates and even mammals in the early postnatal period have robust regenerative capacity, suggesting regenerative therapies are a viable approach to restore function, enhance quality of life, and improve mortality.
Although pharmacologic approaches have been responsible for significant reductions in mortality and slow the progression of ventricular remodeling, they do not address the underlying etiology of heart failure, namely, the loss of contractile myocardium. Despite the compelling need for regenerative therapies in heart failure and two decades of concerted effort, such treatments have yet to transform contemporary cardiovascular practice. A multitude of cell-based (adult and progenitor cells, pluripotent and multipotent cell-derived cardiomyocytes, stromal cells, engineered tissues), gene-based (noncoding RNA, episomes), and cell-free (secretomes, growth factors, biomaterials) therapies have been proposed ( Fig. 41.1 ). The most promising of the cell- and gene-based investigational therapies will be reviewed in this chapter.

Mechanisms of Action for Cardiac Cell Therapy
Cell therapy broadly aims to achieve two distinct but complementary goals: (1) direct cell replacement through implantation of cells or grafting of tissues into the injured myocardium and (2) stimulation of endogenous repair processes such as angiogenesis, inflammation, apoptosis, and fibrosis ( see Fig. 48.1 ). Direct cell or tissue replacement offers the most intuitive strategy to replace cardiac myocytes (“remuscularization”) and restore function following acute MI; however, it has also been the most elusive. Remuscularization may be the only effective strategy for irreversible fibrosis or remodeling associated with chronic injury, a larger albeit more complex indication than acute injury. However, replacement therapies remain exploratory at this stage in development. Whether adult stem cells are even capable of forming new cardiac muscle is an area of great debate ( see also Chapter 3 ). In contrast, replacement strategies based on pluripotent stem cells can clearly remuscularize infarcted myocardium in preclinical models, but have their own set of challenges that will need to be overcome before they are a viable clinical strategy.
The first cell type investigated for cardiac cell replacement therapy was adult skeletal myoblasts over two decades ago. Although initially thought to transdifferentiate into cardiomyocytes, this has been conclusively shown not to occur, and the cells themselves do not couple electrically with the host myocardium. Clinical trials of autologous skeletal myoblast transplantation in patients with heart failure did not improve regional or global LV function and caused ventricular arrhythmias, prompting abandonment of this cell type for therapy. More recently, efforts have shifted to other adult sources of cells purporting regenerative benefit through cell-cell and paracrine mechanisms, activating and stimulating endogenous regeneration and modulating repair mechanisms. Numerous autologous and allogeneic adult cell types have been investigated clinically, including adult stem cells of cardiac origin such as cardiac stem/progenitor cells (CSCs/CPCs) and noncardiac origin such as cardiosphere-derived cells (CDCs), as well as various bone marrow (BM)-derived cells (e.g., BM-derived mononuclear stem cells [BM-MNCs], and BM-derived mesenchymal stem cells [BM-MSCs]) ( Table 41.1 ). These so-called first-generation cell types have been further refined as “second-generation” cells composed of highly purified subpopulations or modified to potentiate their regenerative capacity. Development of these adult cell types has been accelerated to numerous phase II/III clinical trials within the past decade. With the realization that cell therapy has multiple effects in addition to regeneration, including cell-cell interaction and paracrine secretion of cardioactive cytokines and growth factors, investigators have expanded the indications for cell therapy from acute MI to ischemic and nonischemic cardiomyopathy to refractory angina, peripheral artery disease, and stroke. Recently, it has been recognize that cell-free preparations are capable of replicating the beneficial effects of cell therapy; these secretomes consist of extracellular vesicles/exosomes and growth factor-enriched myocardial matrixes. Whether they account for all of the benefits of cellular therapy and whether they are equally efficacious will need to await further clinical development.
Study | Design | NUMBER OF SUBJECTS | Cell Type | Route | Cell Number (×10 6 ) | Timing (Post-AMI) | Follow-up (Months) | Primary Outcome | Result | |
---|---|---|---|---|---|---|---|---|---|---|
T | C | |||||||||
Acute Myocardial Infarction | ||||||||||
Wollert et al. (BOOST) | SC, OL | 30 | 30 | Allo BM-MNC | IC | 2460 | 5–7 days | 6 | Global LVEF | Positive |
Schachinger et al. (REPAIR–AMI) | MC, DB | 95 | 92 | Auto BM-MNC | IC | 236 ± 174 | 3–6 days | 4 | Global LVEF | Positive |
Janssens et al. (Leuven-AMI) | SC, DB | 33 | 34 | Auto BM-MNC | IC | 172 ± 72 | 24 hours | 4 | Global LVEF | Negative |
Huikuri et al. (FINCELL) | MC, DB | 39 | 38 | Auto BM-MNC | IC | 402 ± 196 | 3 days | 6 | Global LVEF | Positive |
Tendera et al. (REGENT) | SC, OL | 97 | 20 | Auto BM-MNC or CD34+CXCR4+ BM-MNC | IC | 178 (BMMNC) 1.90 (CD34+CXCR4+ BMCs) | 3–12 days | 6 | Global LVEF | Negative |
Roncalli et al. (BONAMI) | MC, OL | 52 | 49 | Auto BM-MNC | IC | 98.3 ± 8.7 | 9 days | 3 | Myocardial viability | Negative |
Hirsch et al. (HEBE) | MC, OL | 69 | 65 | BM-MNC/PB-MNC | IC | 296 ± 164 (BM) 287 ± 137 (PB) | 5–7 days | 4 | Global or regional LVEF | Negative |
Traverse et al. (LateTIME Trial) | MC, DB | 58 | 29 | Auto BM-MNC | IC | 150 | 2–3 weeks | 6 | Global LVEF | Negative |
Traverse et al. (TIME) | MC, DB | 79 | 41 | Auto BM-MNC | IC | 147 ± 17 | 3 or 7 days | 6 | Global or regional LVEF | Negative |
Choudry et al. (REGENERATE-AMI) | MC, DB | 55 | 45 | Auto BM-MNC | IC | 60 | <24 hours | 12 | Global LVEF | Negative |
Sürder et al. (SWISS-AMI) | MC, DB | 95 | 55 | Auto BM-MNC | IC | 152 | 5–7 days or 3–4 weeks | 12 | Global LVEF | Negative |
Quyyumi et al. (PreSERVE-AMI) | MC, DB | 78 | 83 | Auto BM-MSC | IC | 10 ± 2 | 9 days | 6 | Resting myocardial perfusion | Negative |
Wollert et al. (BOOST-2) | SC, OL | 151 | 37 | Allo BM-MNC | IC | 700–2080 | 8.1 ± 2.6 days | 6 | Global LVEF | Negative |
Mathur et al. (BAMI, NCT01569178 ) | MC, DB | ∼175 | ∼175 | Auto BM-MNC | IC | 2–8 days | 24 | All-cause mortality | Recruiting, estimated completion 2019 | |
Ischemic Cardiomyopathy | ||||||||||
Menasche et al. (MAGIC) | MC, DB | 67 | 30 | Skeletal myoblasts | TEP | 400 or 800 | >1 month | Global or regional LVEF | Negative | |
Perin et al. (FOCUS-HF) | SC, OL | 20 | 10 | Auto BM-MNC | TEN | 178 | >3 months | 12 | QOL, MLHFQ | Positive |
Perin et al. (FOCUS-CCTRN) | MC, DB | 61 | 31 | Auto BM-MNC | TEN | 100 | >1 month | 6 | LVESV, VO 2 max, SPECT reversibility | Negative |
Assmus et al. (CELLWAVE) | SC, DB | 82 | 40 | Auto BM-MNC | IC | 205 ± 110 | >3 months | 4 | Global LVEF | Positive |
Patel et al. (ixCell-DCM) | MC, DB, sham control | 58 | 51 | Proprietary auto BM-MSC and M2 macrophages | TEN | >3 months | 12 | Composite (all-cause death, cardiovascular hospitalizations, and worsening HF) | Positive | |
Bartunek et al. (CHART-1) | MC, DB, sham control | 120 | 151 | Auto BM-CpSC | TEN | 24 | >3 months | 40 | Composite (all-cause death, worsening HF, MLHFQ, 6MWT, LVESV, and LVEF) | Negative |
Choudhury et al. (REGENERATE-IHD) | SC, DB | 70 | 35 | G-CSF/auto BM-MNC | IC or TEN | 115.1 | >3 months | 12 | Global LVEF | Positive for TEN |
CONCERT-HF ( NCT02501811 ) | MC, DB | ∼72 | ∼72 | Auto BM-MSC/c-kit + CSC | TEN | 12 | Global LVEF, VO 2 max, 6MWT, etc. | Recruiting, estimated completion 2018 | ||
DREAM HF-1 ( NCT02032004 ) | MC, DB | ∼300 | ∼300 | Auto BM-MPCs | TEN | 12 | Time to HF exacerbation | Recruiting, estimated completion 2018 | ||
CHART-2 ( NCT02317458 ) | MC, DB, sham control | ∼200 | ∼200 | Auto BM-CpSC | TEN | 52 | Composite (CV death, worsening HF, MLHFQ) | Recruiting, estimated completion 2020 | ||
Assmus et al. (REPEAT, NCT01693042 ) | MC, OL | ∼334 | ∼334 | Auto BM-MNC | Repeated IC | 24 | All-cause mortality | Recruiting, estimated completion 2022 |
Given their proven potential to remuscularize infarcted tissue, there is great interest in pluripotent stem cells such as human embryonic stem cells (ESCs) as a renewable source of differentiated cardiomyocytes. First isolated in 1998, human ESCs are isolated from the inner cell mass of the blastocyst in the early stages of embryogenesis. These cells retain the potential to differentiate into any somatic cell type given the appropriate stimulation. Initially, there was hope that the heart milieu itself could provide either critical cell-cell cues or growth factors to guide ESCs to a cardiac phenotype and integrate into host myocardium. This notion was quickly dispelled as injected ESCs into mouse myocardium formed teratomas rather than mature cardiomyocytes, in addition to eliciting immunogenicity and graft rejection. However, cardiomyocytes derived from ESCs can be transplanted and survive in normal rodent hearts and electrically couple with existing cardiomyocytes in porcine models. When transplanted into recipient rodent models after MI, there was a reproducible and durable improvement in LV function and electrical coupling with the host myocardium. Efficient methods for high-purity, clinical-grade cardiomyocyte production from ESCs now allow extension of replacement cell strategies into preclinical large animal studies. Upward of one billion human ESC-derived human cardiomyocytes (hESC-CMs), approximating the cell loss during anterior MI, have been successfully implanted in postinfarction, nonhuman primates. In this seminal study by Chong et al. and Murry et al., hESC-CMs were engrafted into infarcted primate hearts via epicardial injection, resulting in significant remuscularization. The human graft became vascularized and electromechanically coupled with the host myocardium within 2 weeks posttransplant and remained durable up to 2 months, albeit with significant immunosuppression to prevent xenograft rejection. A more recent example demonstrated the effectiveness of allogenic transplantation of 400 million primate induced pluripotent stem cell (iPSC)-derived cardiomyocytes into major histocompatibility complex (MHC) haplotype homozygous matched primates. As expected with significant engraftment of ectopic ventricular tissue, ventricular arrhythmias were observed in both studies, albeit transient and hemodynamically tolerated. Arrhythmogenicity of such grafts appears be proportional to graft size and the size of the host heart (no ventricular arrhythmias were noted in preceding small-model studies) and can be expected to pose a challenge in large animal studies. Although these preclinical proof-of-concept studies show promise and are grounded in more than three decades of basic science scholarship transitioning from in vitro and small animal models to more relevant large animal studies, significant challenges to clinical translation remain. Efficient and reproducible cell production and processing, avoidance or suppression of ventricular arrhythmias, graft survival without prohibitive immunosuppression, and currently surgical epicardial delivery must be addressed prior to broad clinical acceptance. To circumvent many of these issues, an alternative strategy using a surgically placed epicardial patch seeded with ESC-derived CPCs is already enrolling a first-in-human trial, despite recent evidence suggesting that cardiac progenitors do not durably engraft and any benefit is mediated through transient paracrine mechanisms.
The discovery of an alternative pluripotent stem cell, iPSCs, by Takahashi and Yamanaka et al. has markedly accelerated pluripotent stem cell research and translation into potential therapies. Overexpression of four genes (c-Myc, Oct3/4, SOX2, and Klf4) known to maintain pluripotency in stem cells reprogramed somatic cells back to a state of pluripotency. The process has been validated using a full spectrum of somatic cells, including cells isolated from a single hair follicle or a sample of blood. iPSCs offer benefits over ESCs such as autologous source, allowing patient-specific human leukocyte antigen (HLA) compatibility, potentially obviating the need for immunosuppression, and avoiding the societal issues surrounding blastocyst and embryo research. However, the reprograming process has been reported to result in genomic abnormalities and incomplete reprograming, leaving residual epigenetic marks that are of uncertain clinical significance. However, cardiomyocytes generated from iPSCs appear functionally indistinguishable from cardiomyocytes derived from ESCs and native cardiomyocytes, although they are phenotypically immature. Engineered sheets of iPSC-derived cardiomyocytes have been ectopically transplanted onto failing postinfarction hearts in pig with benefit, although the clinical relevance of such an approach is unclear given that such grafts failed to couple electromechanically or vascularize with the host myocardium.
Clinical Trials of Cardiac Cell Therapy
The first cardiac cell therapy trials were performed more than 20 years ago, with intracoronary delivery of BM-MNCs. There have now been more than 100 clinical trials of cell therapy for MI, more than 90 for chronic ischemic cardiomyopathy, and 25 for nonischemic cardiomyopathy. Trials to date have generally used heterogeneous populations of adult cell types and have consistently shown safety regardless of the specific investigational cell product, delivery approach, dosing protocol, or patient characteristics. Individual trials initially suggested efficacy, but these early trials were small without randomization, standardized enrollment criteria, or end points. More recent trials with larger cohorts and superior study design have generally failed to convincingly show benefit over guideline-directed medical therapy (see Table 41.1 ). A recent Cochrane meta-analysis of 38 randomized control trials capturing 1907 post-MI patients concluded the current body of evidence for cell therapy to be low quality and lacking evidence for benefit by composite end point of mortality, nonfatal MI, and/or heart failure readmission. Long-term mortality greater than 12 months and incidence of nonfatal MI were individually reduced with cell therapy but confounded by relatively low event rates, small study cohorts, and nonstandardized trial designs and adjudication. Numerous challenges remain for the clinical translation of cardiac cell therapy ( Fig. 41.2 ). Several representative trials of cell therapy are presented here to highlight these challenges facing cardiac cell therapies.

Bone Marrow–Derived Mononuclear Cells
BM-derived stem cells encompass a diverse cell population, of which BM-MNCs and MSCs have been studied the most clinically. In one of the original and most controversial papers in the field, Orlic et al. reported myocardial regeneration with new myocyte formation following acute MI with BM-MNC therapy in mice, which was rapidly extended into large animal and human studies. To date, this study has not been reproduced, and there is no direct evidence that any BM cell derivative can differentiate into a mature cardiomyocytes. Nevertheless, given the proposed alternative beneficial effect through cell-cell or paracrine mechanisms of action, clinical trials of BM-MNCs particularly in post-MI patients have continued to increase. The trials have generally yielded inconclusive or conflicting results likely due to the small size of phase I/II studies, heterogeneity of cell isolation, preparation, dosing, timing of therapy, baseline patient characteristics, end point adjudication, and study design. Routinely, modest and inconsistent but promising results in pilot and single-center phase I trials have not been reproduced in larger, multicenter phase II trials. For example, the original BOne marrOw transfer to enhance ST elevation infarct regeneration (BOOST, NCT00224536 ) trial of autologous intracoronary BM-MNCs randomized 60 ST-segment elevation myocardial infarction (STEMI) patients to therapy or placebo and showed improvement in LV systolic function but was not supported in the follow-up BOOST-2 trial (ISRCTN17457407) of 153 STEMI patients randomized to cell therapy or placebo. To definitively answer the role of BM-MNCs as cardiac cell therapy, the Bone marrow–derived mononuclear cells on all-cause mortality in Acute MI (BAMI, NCT01569178 ) trial represents the culmination of more than a decade of investigation in BM-MNCs for acute MI and LV dysfunction. BAMI is the first phase III trial designed to show benefit in all-cause mortality; however, enrollment has been significantly reduced from the initial 3000 to 350 patients due to challenges with recruiting patients. Given the significant reduction in enrollment, it is unclear whether the trial will have sufficient statistical power to demonstrate the beneficial effects of adjunctive intracoronary infusion of autologous BM-MNCs in addition to revascularization and guideline-directed medical therapy in patients with acute MI and impaired left ventricular ejection fraction (LVEF) (≤45%). Recruitment for the multinational, open-label, randomized controlled trial commenced in 2016 and is anticipated to conclude in 2019 after 2-year minimum follow-up. For postinfarction ischemic cardiomyopathy, REpetitive Progenitor cEll therapy in Advanced chronic hearT failure (REPEAT, NCT01693042 ), is a multicenter, open-label, randomized controlled trial of single versus repeat intracoronary infusion of BM-MNCs powered for all-cause mortality at 2 years currently enrolling for an anticipated completion in 2022. If the BAMI and REPEAT trials are successfully completed, their outcomes will help to more definitively answer the efficacy of BM-MNCs in acute MI and ischemic cardiomyopathy, respectively.
Bone Marrow–Derived Mesenchymal Stem Cells
The Prospective Randomized Study of Mesenchymal Stem Cell Therapy in Patients Undergoing Cardiac Surgery (PROMETHEUS, NCT00587990 ) trial randomized nine patients undergoing cardiac surgical revascularization to intravenous BM-MSC therapy or placebo and provided early safety data and signal towards long-term benefit at 18 months. More recent studies have continued to demonstrate safety but generally negative primary outcomes, including the PreSERVE-AMI trial of intracoronary infusion of autologous CD34+ BM-MSCs following acute STEMI with impaired LV function. Notably, the multicenter, double-blind, sham-controlled trial ixCELL-DCM studying a proprietary therapeutic cell product consisting of autologous BM-MSCs and M2 macrophages in 126 patients with dilated ischemic cardiomyopathy demonstrated a positive impact on the primary composite end point of all-cause death, cardiovascular hospitalizations, and heart failure decompensation at 1 year, although driven primarily by increased heart failure events in the sham control cohort.
Cardiac Stem/Progenitor Cells
Several groups have reported that multipotent CSCs/CPCs exist in the heart and proposed they can effect true regeneration, either directly or through stimulation of endogenous regenerative capacity and CSC niches, although this has been disputed. The potency of these cells declines with age due to accumulation of DNA damage and telomere attrition and is, unfortunately, inversely related to the incidence and severity of cardiac disease. The Stem Cell Infusion in Patients with Ischemic cardiomyopathy (SCIPIO, NCT00474461 ) trial was the first randomized study of intracoronary autologous CSCs/CPCs in ischemic cardiomyopathy. The small study of 20 treatment and 13 control patients with LVEF less than 40% randomized to cell therapy or placebo at 4 months post MI at the time of surgical revascularization demonstrated improved LVEF from 30% at baseline to 38% and 42% at 1 and 2 years, respectively, while the placebo control experienced a 3.7% absolute improvement in LVEF at 2 years. Additional end points such as infarct size and New York Heart Association (NYHA) functional classification were improved at 1 year and sustained at 2 years post therapy. Initial enthusiasm at the positive results have been tempered by controversy and a statement of concern issued by the host journal. Nevertheless, the study has spurred further research into a subpopulation of CSCs/CPCs with high expression of Nkx2.5, Tbx5, Mesp-1, and Mef2C, so-called cardiopoietic stem cells (CpSCs) which are thought to be particularly reparative. Lineage-specification of BM-MSCs using growth factors to guide cardiopoiesis and to enrich the CpSC subpopulation led to the first clinical trial of these so-called second-generation adult stem cell products in the Cardiopoietic Stem Cell Therapy in Heart Failure (C-CURE, NCT00810238 ) trial.
The C-CURE trial used BM-CpSCs in ischemic cardiomyopathy, demonstrating safety and suggestion of efficacy including significantly improved LVEF, reduced left ventricular end-systolic volume (LVESV), and improved 6-minute walk test (6MWT) compared with the standard of care. The follow-up study, European-based Congestive Heart Failure Cardiopoietic Regenerative Therapy (CHART-1, NCT01768702 ) trial, is a double-blind, sham-controlled study of 315 patients with ischemic cardiomyopathy treated with an endomyocardial injection of CpSCs. Although safety was again demonstrated, the trial failed to achieve the prespecified primary composite end point (all-cause death, worsening heart failure, LVEF, LV end-diastolic volume, 6MWT), compared with sham control. The negative result of CHART-1, the largest trial of its kind to date, has raised several questions including the choice of cell type (autologous source with comprised regenerative capacity), untested dosing and delivery, and insufficient statistical power. The North American counterpart, CHART-2, ( NCT02317458 ) is now evaluating whether transendocardial injection of CpSCs is effective in a subgroup of patients with more advanced heart failure and an end-diastolic volume of 200 to 370 mL.
Additional studies of second-generation cell products are currently ongoing. Combination therapy of intramyocardial injection of BM-MSCs and CSCs/CPCs is being studied in the multicenter phase II trial Combination of Mesenchymal and C-Kit+ Cardiac Stem Cells as Regenerative Therapy for Heart Failure (CONCERT-HF, NCT02501811 ) of 144 patients with ischemic cardiomyopathy randomized to BM-MSCs alone, CSCs/CPCs alone, combination MSCs and CSCs/CPCs, or placebo. The multicenter phase II trial Efficacy and Safety of Allogeneic Mesenchymal Precursor Cells (Rexlemestrocel-L) for the Treatment of Heart Failure (DREAM HF-1, NCT01569178 ) will randomize 600 patients with ischemic cardiomyopathy to mesenchymal precursor cells or placebo (study completion date of 2019). Finally, the phase I/II Transendocardial Autologous Cells in Heart Failure Trial (TAC-HFT, NCT02503280 ) will investigate combined MSCs and CSCs/CPCs or placebo in idiopathic dilated cardiomyopathy and is intended to start enrollment in 2020.
Cardiosphere-Derived Cells
A unique, heterogeneous population of endogenous cells derived from heart explants called CDCs have undergone extensive preclinical and clinical testing. Cardiospheres are heterogeneous spheroid-forming colonies of nonmyocyte cells that are formed from adult heart tissue in culture, which include MSCs, smooth muscle cells, endothelial cells, cardiac fibroblasts, and presumably CPCs. In preclinical studies, CDCs improved LV function when transplanted into infarcted myocardium. The single-center Cardiosphere-Derived Autologous Stem Cells to Reverse Ventricular Dysfunction (CADUCEUS, NCT00893360 ) trial randomized patients post MI with impaired LVEF less than 40% to cell therapy or placebo. At 12 months, therapy failed to improve functional end points of LVEF or NYHA functional classification, although scar size was reduced 12.3% at 12 months compared with placebo. The follow-up multicenter phase II trial Allogeneic Heart Stem Cells to Achieve Myocardial Generation (ALLSTAR, NCT01458405 ) was recently terminated early for futility to achieve the primary end point of scar size by magnetic resonance imaging (MRI) after interim analysis at 6 months. CDC therapy is currently being investigated for dilated cardiomyopathy in end-stage Duchenne muscular dystrophy.
Open Questions in Optimizing Cardiac Cell Therapy
The modest and/or frequent negative results of cardiac cell therapy trials have been attributed to a number reasons, both specific to the therapy itself and the study design used. However, although each of these interacting variables could have significant influence on the efficacy of cell therapy, it is not clear if, or how, these vexing issues will ever be resolved given the accumulating negative trials in the field of adult cell therapy.
Cell Source
Multiple competing cell types have been proposed for cardiac cell therapy without comparative study to assess superiority or clearly defining the mechanisms of action for benefits (regenerative versus paracrine). Combination therapy could also provide additive benefits given the diverse mechanism of action of the various cell types but has not been studied systematically. Although combination therapy is being pursued in the ongoing CONCERT and TAC-HFT trials, it is questionable whether sufficient preclinical data exist to identify the most effective combinations. Another important variable is autologous versus allogeneic source. Although autologous source avoids the immunocompatibility issues of allogeneically sourced cells, patient comorbidities such as advanced age, diabetes, smoking, and obesity may limit the biologic potency of harvested cell components. Allogeneic cells also allow for “off-the-shelf” product development under strict quality-controlled conditions and ease of scalability.
Cell Dose
For replacement therapies such as proposed with pluripotent stem cell-derived cardiomyocytes, dose escalation until lost myocardium is fully remuscularized seems logical. Accordingly, preclinical studies have described administration of upwards of one billion ESC-derived cardiac myocytes, although full dosing studies in appropriate human-sized models have not occurred. For adult stem cell products with primarily paracrine effects, the appropriate dosing is unclear. A reverse dose response has been reported in the POSEIDON trial likely due to vascular obstruction and maladaptive neoangiogenesis with intracoronary administration, whereas higher doses appeared beneficial for transendocardial delivery, as observed in the TRIDENT trial. Variable cell potency further complicates the issue of cell dosing, particularly for autologously sourced cells. In the absence of well-powered and appropriately controlled dose response trials, optimal dosing remains unknown.
Timing of Therapy
Although often reported interchangeably, administration of cells in the acute, subacute, or chronic phases following MI would be expected to work by significantly different mechanisms and require different properties. Acute administration aims to modulate inflammation and apoptosis, whereas subacute or chronic therapy aims to augment myocardial repair and regeneration. Although not studied systematically, a meta-analysis of adult cell therapy suggests that 2 to 8 days post MI is the most favorable window to modulate injury response and potentiate repair mechanisms. Interestingly, preclinical studies have generally focused on the subacute phase, occurring at 2 weeks after the resolution of acute phase inflammation and reperfusion injury that may be hostile to engraftment. Indeed, ESC-derived cardiac myocyte transplantation is beneficial only in this subacute phase, insofar as limited studies in chronic cardiomyopathy have been negative.
Route of Administration
Several routes of cell administration have been studied preclinically, including intravenous, percutaneous endomyocardial, intracoronary, transcoronary endocardial, retrograde intracoronary sinus with antegrade coronary obstruction, and open surgical epicardial. By far the most common route of adult cell delivery post MI is intracoronary infusion, given the central role for catheter-based revascularization in contemporary practice. However, intracoronary cell delivery is associated with rapid washout and little retention of cells, which may be sufficient for paracrine effects but not for remuscularization. Intramyocardial injection, whether epicardially or endocardially delivered, is superior for cell engraftment but poses a higher risk of arrhythmia and perforation of the infarcted myocardium after MI and thus may be more appropriate for subacute and chronic therapy where remuscularization is the goal. More granular issues of optimal target tissue, such as periinfarct versus intrainfarct myocardium or transmural infarcted versus stunned/hibernating myocardium, are largely unexplored.
Patient Characteristics
The myriad of patient characteristics affecting myocardial substrate have not been studied yet undoubtedly impact response to cell therapy, particularly for autologous cell products where poor patient parameters impair both cell potency and host receptivity have not been investigated. Patient comorbidities such as advanced age, diabetes, and injury-related features such as ischemia time, reperfusion, revascularization strategy, and reperfusion burden are highly variable between patients and influence the natural history of infarction and subsequent heart failure. Given the small size of existing studies and heterogeneity with respect to inclusion criteria, it is unlikely this will be resolved soon.
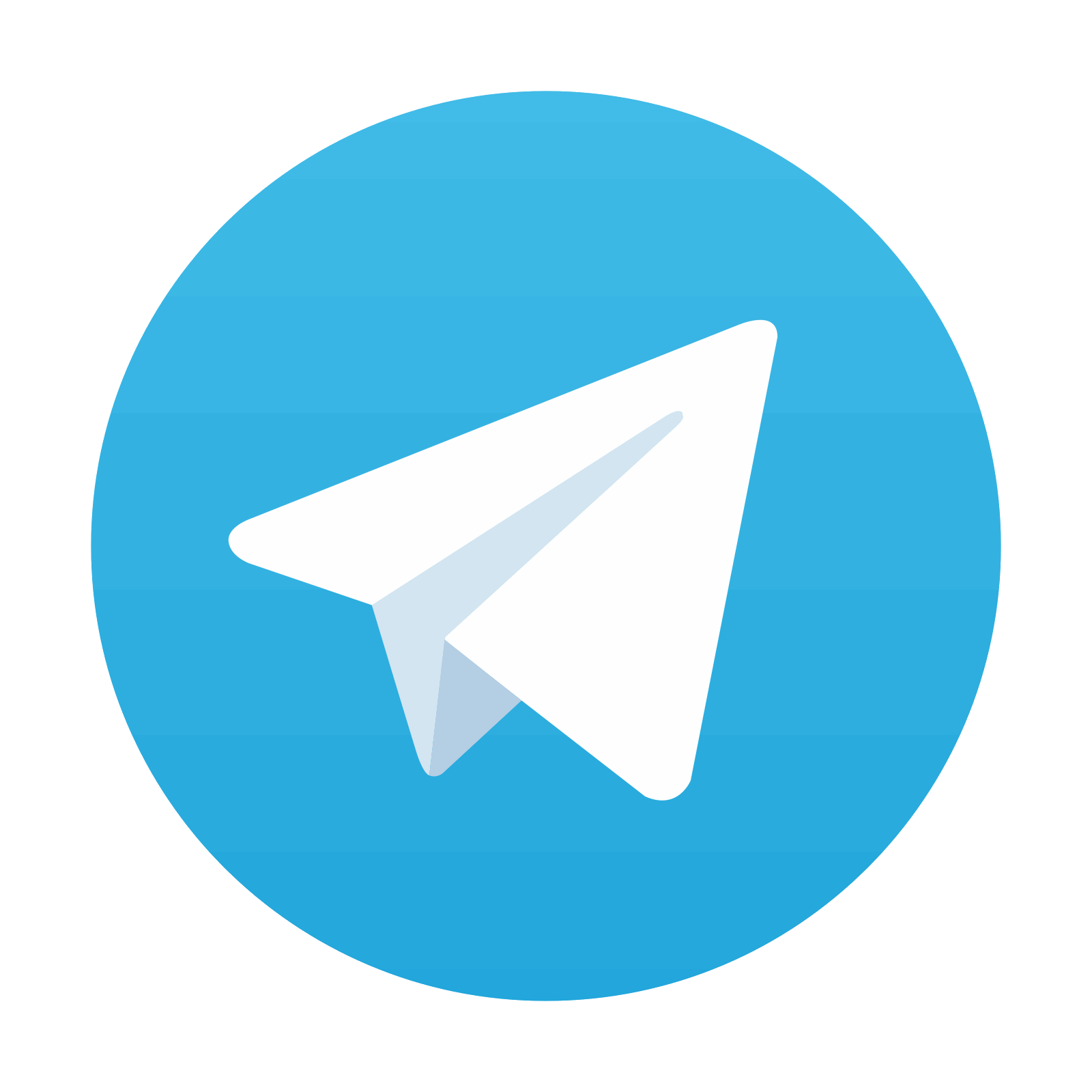
Stay updated, free articles. Join our Telegram channel

Full access? Get Clinical Tree
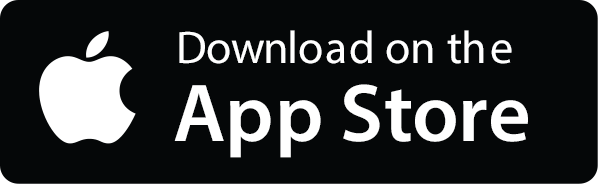
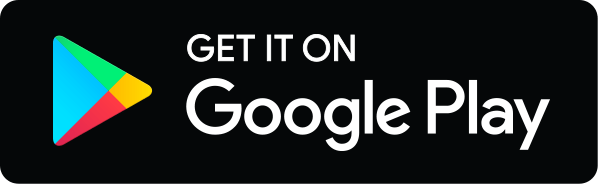