(1)
Division of Public Health Sciences, Wake Forest School of Medicine, Winston-Salem, NC, USA
Synopsis
QT interval is the repolarization time (RT) of the ventricular region repolarized last. Epicardial and endocardial RT (RTepi and RTendo) represent regional RTs and QT subintervals. Based on these ECG measures of repolarization a working model was constructed to account for gender differences in the waveform of the ST and the durations of QT and QT subintervals.
Normal and abnormal repolarization patterns are generated by ion channel currents which modulate regional action potential durations (APD) and waveforms. In normal men the transient outward potassium current (Ito) plays an important role in generating the characteristic male ST-T waveform. Increased Ito shifts the ST segment dome to a higher potential level, reduces APD, enhances activation of the delayed rectifiers (IKs,IKr) and promotes faster repolarization. Action potential phase 2 and phase 3 slopes decrease (become more negative) generating the asymmetric T waveform in males with upsloping ‘U-shaped’ ST segment with reduced convexity and tall T wave.
Epicardial and endocardial action potential difference at phase 2 in women in contrast to men, is minimal so that STJ-point elevation is absent and ST segment is flat. The convexity of the J-Tp segment is high. Potassium channel subunit Kir2.3 levels in females at epicardium have been found to be lower than at endocardium. Kir2.3 mediates cardiac inward rectifier IK1. There are several expected results from lower Kir2.3 level. RTepi is prolonged more than RTendo. Rate-adjusted QTpeak (QTpa) prolongs. The interval from Tpeak (Tp) to the inflection point at T wave downstroke (Txd) representing crossmural dispersion of left ventricular repolarization (TpTxd interval) increases and also QT increases if TpTe remains unchanged or increases (by reduced IK1 prolonging phase 3 at basal regions normally repolarized last).
The gender difference in QT is due to QT shortening in adolescent boys and QT remains shorter in men than in women through adulthood. Testosterone modulates the main repolarizing current (L-typeCa2+) at phase 2 and the primary repolarizing current (IKs) during phase 3, shortening APD and QT.
ECG measures of deviant repolarization are clinically important predictors of adverse outcome events, including various angular measures of the spatial direction of deviant repolarization. Sex differences in ion channel currents modulating action potential durations are not only of interest from the perspective of electrophysiological mechanisms to elucidate their mechanism but they have practical clinical implications as described in previous chapters.
Abbreviations Acronyms and Ion Channel Current Labels
AF
Atrial fibrillation
APD
Action potential duration
CHD
Coronary heart disease
CVD
Cardiovascular disease
HF
Heart failure
ICaL
Inward calcium current with long-lasting activated state
ICaT
Transient inward calcium current
IK1
Potassium current in inward rectifier ion channel
IKACh
Inward potassium current through ACh-activated K+ channel.
IKr
Rapidly activating outward rectifying potassium current
IKs
Slowly activating outward rectifying potassium current
IKur
Ultrarapidly activating outward rectifying potassium current
INa
Inward sodium current
Ito
Transient outward potassium current
LGIC
Ligand-gated ion channel ion channel proteins which bind a chemical messenged (ligand) such as neurotransmitter and open a channel to ion transport through the membrane
NCX
Sodium-calcium exchange protein that removes 1 Ca2+ from inside cell in exchange of 3 Na+
SA
Sino-atrial node
SCD
Sudden cardiac death
9.1 Introduction
Consideration of cardiac ion channel currents and the factors that modulate them is of utmost importance for the understanding of the QT interval and other repolarization features. A variety of labels have been used in electrophysiological literature for ion currents. In this chapter the labels for ion currents follow the terminology used by Grant is his extensive review [1]. Ion currents are activated and inactivated at various phases of action potential. Figure 9.1 adapted from the review by Nattel and Carlson [2] shows ion currents that generate normal ventricular action potential upstroke (phase 0), overshoot and notch (phase1), slow phase of repolarization (plateau or phase 2), fast phase of repolarization (phase 3) and the resting potential (phase 4). Of main interest in this context are the ion currents involved in modifying action potential duration (APD) and waveform.
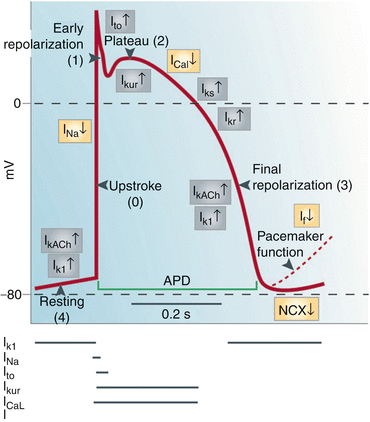
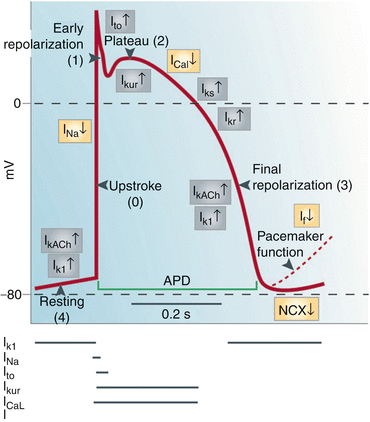
Fig. 9.1
Membrane currents that generate normal ventricular action potential upstroke (phase 0), overshoot and notch (phase1), slow phase of repolarization (plateau or phase 2), and fast phase of repolarization (phase 3 (and the resting potential (phase 4). Broken line shows the SA node in pacemaker potential. The inward currents, INa, ICa, and If, are shown in yellow boxes; the electrogenic sodium-calcium exchanger (NCX) is also shown in yellow although it may generate inward or outward current. The outward currents I KAch, I K1, I to, I Kur, IKr, and I Ks are shown in gray boxes (Reproduced from Nattel and Carlsson [2], with permission)
9.2 Ion Channel Currents Modulating Ventricular Action Potential Duration
These currents include inward calcium currents (ICa), transient outward potassium currents Ito and IKur and delayed rectifier K+currents IKr and IKs shown in Fig. 9.1. ICa2+ is involved with both of these categories in regulating ventricular APD. IKr is the rapidly activating outward rectifying potassium current and IKs the slowly activating outward rectifying potassium current.
The impact of Ito on phase 2 of action potential is through opening of Ca2+ channels thereby modulating action potential duration (APD). Left ventricular epicardial layers where repolarization normally starts have higher levels of Ito expression. The rate of Ito inactivation is variable and highly voltage-dependent. α-adrenergic stimulation reduces Ito in human myocytes through PKA-dependent phosphorylation. Chronic α-adrenergic stimulation and anangiotensin II also reduces channel expression. In large mammals Ito reduction shifts phase 2 plateau to more positive potentials increasing the activation of the delayed rectifier (K+ currents IKr and IKs in Fig. 9.1) and promoting faster repolarization. IKs is expressed in all cell types, but is reduced in midmyocardial myocytes. These cells have the longest action potential duration across the myocardial wall.
9.3 Inward Calcium Current
For the inward calcium currents (ICa) the labels are assigned based on their time-dependent properties. The label ICaL signifies long-lasting and label T in ICaT refers to transient. ICaT channels are scarce or absent in mammalian ventricular myocytes. In atria, ICaT current is carried through a different voltage-gated ionic channel and it may be associated with abnormal automaticity described later. The main focus here is on ICaL in ventricular myocytes.
ICaL activation occurs with a delay with respect of depolarization (which is brought about by inward INa current, phase 0 of the action potential) has reached a lower level of membrane potential. The activated state of ICaL is long lasting. It is important for excitation-contraction coupling by triggering the release of intracellular Ca2+. The balance of inward ICaL current and K+ efflux determines to a large extent the quasi-stationary equilibrium during phase 2 (the slow phase of repolarization generally called “plateau”). ICaL is thus an important determinant of phase 2 duration and the onset of the fast phase of repolarization (phase 3) occurring at the inactivation of ICaL as shown in Fig. 9.1. With inactivation of ICaL the outward potassium current with its high concentrations gradient becomes a dominant force in transmembrane ionic balance and the fast phase of repolarization (phase 3) starts. ICaL is thus an important determinant of APD. Neurotransmitters and calcium channel blockers have a strong influence on ICaL.
9.4 Resting Potential and Inward Rectifiers
From other voltage-gated outward potassium currents, IK1 is the “inward rectifier”. IK1 channels have a high conductance when the membranes are polarized to a high negative value and IKr is important for maintaining the resting potential (phase 4). The conductance of IK1 is reduced sharply when the membrane potential decreases to above – 40 mV at the onset of depolarization (phase 0).
9.5 Pacemaker Potential of the Sino-Atrial Node
Ion channels contributing to phase 4 depolarization include If, IK+ activated during the preceding action potential, ICaL and ICaT, background Na+ current and the sodium-calcium exchanger (NCX). If is activated when the membrane potential falls below −40 mV when repolarization is completed.
Ito has a fast component in human atria. The SA node action potential has a steep slope and short duration in phase 2. Phase 3 starts and ends early and the resting potential starts decreasing immediately (pacemaker potential. The slope of this resting potential decline is rate-dependent and the rate-dependence is more pronounced in women than in men.
IKur is highly expressed in SA node and other atrial myocytes and is the basis for the much shorter duration of the action potential in atria than in ventricles. Ligand-dependent gating with Ach-activated K+ current is a part of the mechanism of the vagal control of the heart rate. Derailed control SA nodes pacemaking activity in AF was covered in Chap. 5.
9.6 Drugs Modulating Cardiac Ion Channels
Classification of antiarrhythmic mechanisms proposed in 1991 by a Working Group on Arrhythmias of the European Society of Cardiology [3] is shown in Table 9.1. The table shows the desired antiarrhythmic effect on the most likely vulnerable period and ion currents most likely to achieve the desired effect.
Table 9.1
Categorization of arrhythmogenic mechanisms, desired antiarrhythmic effect on the most likely vulnerable parameter and ionic currents most likely to achieve the desired antiarrhythmic effect
Mechanism of arrhythmia | Antiarrhythmic effect on vulnerable parameter | Ionic currents most likely to achieve desired effect |
---|---|---|
Enhanced normal automaticity | Decrease rate of phase 4 depolarization | Block If, ICa-T |
Activate IK(Ach) | ||
Abnormal automaticity | Increase (hyperpolarize) max diastolic potential or decrease rate of phase 4 depolarization in latent focus | Activate IK; IK(Ach) |
Block ICa-L; INa | ||
Triggered activity induced by EAD | Shorten APD | Activate IK |
Block ICa-L; INa | ||
Triggered activity induced by DAD | Reduce calcium overload or suppress DAD | Block ICa-L |
Block ICa-L; INa | ||
Na channel dependent reentry with primary impaired conduction (long excitatory gap) | Decrease excitability and conduction | Block INa |
Na channel-dependent reentry with conduction encroaching on refractoriness (short excitatory gap) | Prolong effective refractory period | Block IK |
Ca channel-dependent reentry | Decrease excitability and conduction | Block ICa-L |
Reflection | Decrease excitability | Block ICa-L; INa |
Parasystole | Decrease rate of phase 4 depolarization in automatic (Purkinje) focus | Block If (if max neg diastolic potential hyperpolarized) |
Drugs modulating cardiac ion channels are in the realm of extensive electrophysiological and pharmacological research [4]. This subject area is beyond the scope of the present monograph. The next few paragraphs merely point out the vast range of normal and abnormal conditions related to the multitude of cardiac ion channels and gene mutations.
9.6.1 Potassium Channels and Channel Blockers
KCNH2, the hERG-gene codes for a protein labeled Kv11.1, the alpha subunit of a potassium channel. The hERG potassium channels are essential for normal electrical activity in the heart. Inherited LQTS is associated with mutations in the hERG gene predisposing individuals to life-threatening arrhythmias. Arrhythmia can also be induced by a blockage of hERG channels by a large number of diverse cardioactive agents which has caused disappointing results for many preclinical drug safety studies.
The origin of the label ‘Ether-à-go-go’ denoting the hERG-gene is interesting. According to Wikipedia, The hERG gene is the human homolog of the Ether-a-go-go gene found in the Drosophila fly and named in the 1960s by William D. Kaplan. When flies with mutations in this gene are anaesthetized with ether, their legs start to shake, like the legs of the dancers at the then popular ‘Whisky A Go-Go’ nightclub in West Hollywood.
Arrhythmias related to abnormal repolarization are commonly caused by mutations in genes encoding K+-channels. Mutations in hHERG-gene and in gene encoding KvLQT1 cause the majority of Romano-Ward syndromes, autosomal dominant LQTS. Quinidine, procainamide, and disopyramide are examples of drugs that block K+-channel prolonging QT and in some setups causing TdP. Antiarrhythmic drugs have lost their appeal because of serious side effects although they are important in the management of patients with recurrent AF. IKur channel is a new target for search of new drugs for AF after discovery of atrial-specific distribution of IKur.
9.6.2 Ca2+: Channel
Verapamil and diltiazem are examples of class IV drugs blocking open and inactivated Ca2+– channels. The sudden cardiac death syndrome combining ECG features of the Brugada syndrome including short QT associated with a loss of function of the L-type Ca2 α1- or β2b subunit of the channel has been described by Antzelevitch et al. [5].
9.6.3 If: Channel
This channel has been targeted in search for agents (ivabradine etc.) which slow down heart rate without negative inotropic effect or hypotension which is the disadvantage of β-blockers. These drugs have been found effective in management of patients with stable angina pectoris. Newer findings include mutations of hyperpolarization-activated cyclic nucleotide-gated potassium channel 4 or HCN4 associated with idiopathic sinus bradycardia and chronotrophic incompetence in one family [6] and severe bradycardia, QT prolongation, and torsade de pointes in another family [5]. If expression is upregulated in cardiac hypertrophy and congestive heart failure and may contribute to the arrhythmias observed in these disease conditions.
9.7 QT Interval
9.7.1 QT Rate-Adjustment Formulas
A variety of formulas with substantial differences in functional form have been introduced in ECG literature for QT adjustment for heart rate variation [7, 8]. QT rate sensitivity in women is higher than in men (the slope coefficient in linear regression of QT vs. RR interval is larger in women than in men) and the rate sensitivity decreases with age in both gender groups. The formulas by Bazett [9] and Fridericia [10] introduced in 1920 continue to be most commonly used for QT rate adjustment in clinical applications. Bazett’s formula (QTcBz) leaves a large bias in rate-adjusted QT at high and low heart rates by overcorrecting at higher heart rates and undercorrecting at low heart rates. This increases the proportion of false negative QT prolongations at high heart rates and false positives at low heart rates. The bias with Fridericia’s formula (QTcFr) is in opposite direction to that with QTcBz although the bias is smaller in magnitude. Both of these two formulas ignore the gender difference in QT rate sensitivity. Another problem with many QT rate adjustment formulas is that they may produce a satisfactory rate adjustment of the mean or median QT but do not properly adjust upper limits of QT distribution [8].
Newer formulas introduced labeled QTcLogLin and QTcMod in Table 9.2 may reduce the problems noted above [8]. This table provides a framework for categorization of QTc formulas as linear and inverse based on their generic form and QT prediction function. Rate-adjusted upper normal limits are strongly age- and gender-dependent.
Table 9.2
Categorization of QTc formulas as linear and inverse based on their generic forms and QT prediction functions
QTc formula label
![]() Stay updated, free articles. Join our Telegram channel![]() Full access? Get Clinical Tree![]() ![]() ![]() |
---|