Chapter 8
Spinal Cord Injury
Written by W. Mudzi, H. van Aswegen and B.M. Morrow
Spinal cord injuries remain a major health concern the world over, with an annual incidence of 12.1–57.8 cases per million in developed and 2.1– 130.7 cases per million in developing countries (Rahimi-Movaghar et al., 2013); up to 10% of these injuries will occur in children (Clarke, 2012). In developing countries, spinal cord injury (SCI) often involves young adults (aged 20–30) in the prime of their economically productive lives; whereas in developed countries the reported mean age (40–60 years) tends to be higher, possibly due to higher mean population age and better medical care systems (Ackery et al., 2004; Rahimi-Movaghar et al., 2013). Spinal cord injury is not a notifiable disease and hence figures relating to the cost to the individual and the health care system, especially in the developing world, are difficult to find (Ackery et al., 2004; Wyndaele and Wyndaele, 2006; Cripps et al., 2011). What are not in dispute are the causes and mechanisms of SCI, which will be discussed later in this chapter.
This chapter contains information about:
•The structure of the spinal cord.
•The causes and mechanisms of SCI.
•Spinal cord lesions and classification of the level of injury.
•Respiratory system complications following SCI and changes in respiratory muscle function.
•The complications related to other bodily systems following SCI.
•The medical and surgical management of a patient who sustained SCI.
•The physiotherapy aims for the management of a patient who has sustained SCI in the intensive care unit and the spinal ward.
•The contraindications and precautions related to the physiotherapy management of a patient with SCI.
•The physiotherapy interventions for patients who have suffered SCI.
•Adult and paediatric clinical case scenarios.
8.1. Structure of the Spinal Cord
The spinal column consists of 33 vertebrae and is divided into four regions: cervical, thoracic, lumbar and sacral (Gondim, 2013). Intervertebral discs separate the vertebrae and each vertebra has a vertebral arch, inside which the spinal cord is located. The spinal cord functions as a conduction pathway for impulses to and from the brain and, together with the brain, forms the central nervous system. It also serves as the centre for all spinal reflexes. The spinal cord is a cylindrical structure of nervous tissue that extends from the foramen magnum in the skull to the level of the second lumbar vertebra. It is surrounded by dura mater, arachnoid and pia mater connective tissue membranes and is made up of white and grey matter (Kahle and Frotscher, 2003; Gondim, 2013) (Fig. 8.1).
Nerve roots, which form 31 pairs of spinal nerves with motor and sensory nerve fibres, connect the spinal cord with all bodily structures. There are eight cervical, 12 thoracic, five lumbar and five sacral nerves (Fig. 8.2).
The collection of nerves at the bottom of the spinal column is called the cauda equina, with the conus medullaris at the inferior end of the spinal cord. At the intervertebral foramen of each vertebral body, dorsal nerve roots exit the cord posteriorly and ventral nerve roots enter the cord anteriorly. The nerve roots that leave the spinal cord contain motor fibres and those that enter the spinal cord contain sensory fibres (Fig. 8.1). Spinal nerves C1–C7 exit above their corresponding vertebrae, whereas C8 and the rest of the spinal nerves exit below the level of their corresponding vertebrae (Marieb, 1992; Gondim, 2013).
Fig. 8.1:Diagram of a transection of the spinal cord, illustrating the white and grey matter and sensory and motor spinal nerve roots.
The internal structure of the spinal cord, as viewed transversely, shows a central point of cerebral spinal fluid accumulation, which is surrounded by grey matter (in the shape of an H). The grey matter contains neuronal cell bodies. The outer portion of the spinal cord consists of white matter, which contains nerve fibres (axons) (Kahle and Frotscher, 2003; Gondim, 2013) (Fig 8.1).
Dermatomes are areas of the skin that are innervated by the nerve roots of each spinal nerve. Dermatomes can be mapped fairly accurately on the surface of the body (Fig 8.3).
Table 8.1:Ascending and descending tracts of the spinal cord and their functions.
Name | Location | Function |
Ascending tracts | ||
Lateral spinothalamic | Lateral white columns | Pain and temperature sensation as well as proprioception and exteroception (sensation of impulses that originate outside the body) |
Anterior spinothalamic | Anterior white columns | Crude touch and pressure sensation |
Posterior spinocerebellar | Lateral white columns | Proprioceptive impulses from joints, tendons and muscle spindles |
Fasciculi gracilis and cuneatus | Posterior white columns | Location and quality of tactile stimulation as well as limb position and body posture |
Descending tracts | ||
Lateral corticospinal | Lateral white columns | Voluntary movement and muscle contraction of hands, fingers, feet and toes on the opposite side |
Anterior corticospinal | Anterior white columns | Similar to the above but muscle function on the same side |
Lateral reticulospinal | Lateral white columns | Facilitatory action on motor neurons to skeletal muscles |
Medial reticulospinal | Anterior white columns | Inhibitory action on motor neurons to skeletal muscles |
Loss of sensation in a dermatome therefore acts as an indication of the level of SCI. The dermatomes of the trunk do overlap and are not as clearly defined as the dermatomes on the extremities (Marieb, 1992; Gondim, 2013).
The term myotome refers to a group of muscles that are innervated by the motor fibres of a single nerve root and is referred to as the motor equivalent of a dermatome. Myotome testing, using isometric muscle contraction, can assist with determination of the level of SCI.
The impulse conduction function of the spinal cord is facilitated through spinal cord tracts. The ascending tracts conduct impulses from the body up to the brain and the descending tracts conduct impulses from the brain down to the different parts of the body. Many spinal cord tracts exist, and only the most important ones are listed in Table 8.1 (Kahle and Frotscher, 2003).
A visual summary of these tracts is provided in Fig. 8.4.
Blood supply to the spinal cord is provided by the anterior spinal artery and two posterior spinal arteries. The anterior spinal artery supplies blood to two-thirds of the anterior aspects of the spinal cord. The posterior spinal arteries supply blood to most of the posterior aspects of the spinal cord. In addition to these arteries, segmental arteries that arise from various blood vessels in the cervical, thoracic and lumbar areas of the spine assist with blood supply to the spinal cord. The segmental arteries form anterior medullary and posterior medullary arteries. The great anterior medullary artery supplies blood to the lumbar enlargement of the spinal cord (Newton, 2008).
The reader is referred to Section 8.11 for further reading that offers more detailed information on the structure of the spinal cord.
8.2. Causes and Mechanisms of Injury
8.2.1. Causes of injury in
In developing countries, males are more likely to suffer from traumatic SCI than females (4.8:1), as females are traditionally more involved with home-based activities which place them at a lower risk for SCI (Rahimi-Movaghar et al., 2013). In developed countries the male to female ratio is more equal (3:4) due to females being more involved in competitive sporting and social activities (Rahimi-Movaghar et al., 2013). The causes of SCI can be traumatic or non-traumatic. Traumatic causes of SCI account for 70–80% of injuries through fractures or dislocations. Dislocations account for approximately 25% of trauma cases alone (Pickett et al., 2006; Cripps et al., 2011). Traumatic causes of SCI can be either direct or indirect and are summarised in Table 8.2. Non-traumatic causes of SCI fall outside the scope of this text and will not be discussed.
Table 8.2:Direct and indirect causes of traumatic SCI*.
Direct causes | Indirect causes |
Nerve injury: •Penetrating injury such as gunshot or stab wounds | Injury to the bone, soft tissue or vessels of the spinal cord: •Fracture dislocations:
•Haematoma formation •Ischaemia |
Generally, the most vulnerable areas for SCI through trauma are:
•upper (C1–C2) and lower cervical regions (C5–C7);
•mid-thoracic region (T4–T7); and
•thoracolumbar junction (T10–L2).
These will be discussed below.
8.2.2. Mechanisms of injury in adults
8.2.2.1. Primary mechanisms of injury to the spinal cord
The primary mechanism of injury refers to the initial mechanical trauma to the spinal cord. In cases of violence, the spinal cord may be severed (missile penetration), but more commonly neurological compromise following SCI is the result of ischaemic injury to the cord. Injury to the grey matter occurs within one hour after injury and is irreversible; injury to the white matter becomes irreversible 72 hours after injury (Dumont et al., 2001).
8.2.2.1.1. Dislocation of vertebral bodies
This may occur anywhere in the cervical spine. Most commonly, dislocation occurs between C1 and C2 and C5 and C7 as a result of the lack of a solid vertebral body at C1 (which makes that section of the spine more vulnerable), anatomical curvature of the cervical spine and relative mobility of the lower cervical spine, which attaches to the stiff thoracic spine (Middleditch and Oliver, 2005). Dislocations can also occur in the lower thoracic segments, T11 and T12, but are normally rare in the thoracic region due to stabilisation from the rib cage.
The degree of dislocation does not necessarily parallel the degree of damage to the spinal cord. The degree of injury is influenced by other factors, which include pre-existing degenerative changes in the vertebral spine, blood vessel damage, swelling and disruption of the anterior longitudinal ligament (Sekhon and Fehlings, 2001). Spinal dislocation can also damage nerve roots, intervertebral discs and blood vessels (Baydur et al., 2001).
8.2.2.1.2. Fracture of vertebrae
Vertebral fracture can occur with or without displacement. The fracture may involve vertebral bodies (wedge compression or burst fractures), laminae or pedicles. Bony fragments may be pushed into the spinal canal and impinge on the spinal cord or nerve roots. Table 8.3 summarises the mechanisms involved with various types of vertebral fractures.
Upper cervical spine fractures (C1–C3) occur regularly, with a reported rate of up to 25% (Longo et al., 2010) (Fig. 8.5). Causes of injury to the odontoid or atlas in young adults include motor vehicle accidents and penetrating neck injuries. In the elderly, odontoid peg fractures occur as a result of falls. Traumatic spondylolisthesis of the axis is often referred to as hangman’s fracture. Stable odontoid fractures (fracture of the tip of the odontoid) are managed conservatively, but unstable fractures (fracture at the junction of the odontoid with the body of the axis) might need surgical intervention, where appropriate, as not all elderly people are able to undergo surgery (Longo et al., 2010).
Table 8.3:Mechanisms involved in fractures of the vertebrae*.
Type of fracture | Mechanism of injury |
Compression | |
•Anterior wedge •Lateral wedge | •Anterior flexion •Lateral flexion |
Burst | |
•Grade A •Grades B •Grade C •Grade D | •Axial load •Axial load with flexion •Axial load with rotation •Axial load with lateral flexion |
Fracture-dislocation | |
•Flexion-rotation •Shear | •Flexion-rotation •Anterior-posterior or posterior-anterior |
8.2.2.1.3. Spinal epidural haematoma
Although uncommon, haematoma formation results from bleeding from the traumatic incident but can occur in patients with a coagulation defect following anticoagulation therapy (Binder et al., 2004). It may be acute or chronic, spontaneous, posttraumatic, iatrogenic, or associated with specific medications and disease states (Binder et al., 2004; Cha et al., 2011). Spinal epidural haematoma is mostly localised to the cervical or thoracic spine (Cha et al., 2011). If a patient with spinal epidural haematoma presents with abnormal neurological signs, prompt spinal decompression is performed surgically through evacuation of the haematoma (Cha et al., 2011).
8.2.2.1.4. Spinal subdural haematoma
This is a rare cause of acute spinal cord compression. It can occur with minor trauma and normally presents with what can be termed dramatic symptoms. Paraplegia is preceded by severe back pain in cases of acute spinal subdural haematoma and emergency surgery is often required; however, there are reports of cases in which spontaneous resolution has occurred (Oh et al., 2009).
8.2.2.1.5. Indirect trauma of the spinal cord
This type of injury can result from blows to the head which then result in energy transmission along the brainstem and spinal cord, causing haemorrhages in the grey matter of the upper cervical region.
8.2.2.1.6. Laceration of the spinal cord
Severe injury may lead to laceration of the spinal cord. The most common causes of laceration of the spinal cord (partial or complete) include stab wounds, gunshot wounds and fracture dislocations. Surgical error may also lead to partial laceration of the cord. The continuity of the spinal cord is disturbed and there will be oedema and haemorrhage of meninges and cord followed by scarring of nervous tissue (Dumont et al., 2001).
8.2.2.1.7. Haematomyelia
This can occur as a complication of a fracture dislocation or whiplash injury. It produces a spindle-shaped haemorrhage within the substance of the spinal cord located in the grey matter (Pullarkat et al., 2000). It is usually followed by loss of function below the level of the lesion. As oedema subsides and the clot is slowly absorbed, there is a return of function in the posterior and lateral white columns (Rodesch et al., 2004). The patient usually has a flaccid paralysis in muscles supplied by the anterior horn cells at the level of the lesion. There is gradual development of spastic paralysis, with loss of pain and temperature sensation over segments affected by the clot. Pain and temperature sensation are preserved below the level of the haematomyelia and posterior column function is normal. Haematomyelia is managed surgically either through aggressive (urgent clot evacuation) or less aggressive approaches (plateau of neurologic deficit prior to clot removal), depending on the preference of the attending surgeon. Regardless of the management approach used, the underlying cause for the haematomyelia should be adequately addressed (Oskouian, 2012).
8.2.2.2. Secondary mechanisms of injury to the spinal cord
Secondary mechanisms of injury to the spinal cord set in within minutes of the primary injury and involve a cascade of vascular, cellular and biochemical events that extend from the primary mechanism of injury. The secondary mechanisms of injury may last for weeks or months after the initial injury, and during this phase the area of trauma enlarges (Oyinbo, 2011). Fewer inflammatory mediators are released with mild SCI and may result in less secondary cord injury compared to that caused by moderate or severe primary SCI (Oyinbo, 2011). Delayed specialised management of the patient with acute SCI may further contribute to a worsened outcome due to the ensuing secondary damage to the cord (Oyinbo, 2011).
Secondary SCI occurs when blood flow at the site of injury is reduced due to local vascular injury to the venules and capillaries, vasospasm and intravascular thrombosis, which lead to ischaemia. Over the ensuing hours hypoperfusion and ischaemia spread outwards from the injury site, up and down the spinal cord. Spinal cord autoregulation is lost and cord injury is exacerbated by systemic hypotension (Dumont et al., 2001; Kwon et al., 2004). Other causative factors of ischaemia of the spinal cord include oedema, inflammation and cord compression. The ischaemic area encourages the release of inflammatory mediators such as reactive oxygen species. These inflammatory mediators cause oxidative cell membrane damage, which may eventually lead to apoptosis and cell death (Dumont et al., 2001; Kwon et al., 2004). An in-depth discussion of the complex intracellular mechanisms that contribute to apoptosis and cell death following SCI is beyond the scope of this text. Readers are referred to Section 8.11 for further suggested reading.
8.2.2.2.1. Neurogenic shock
Neurogenic shock results from central nervous system injury such as cervical or high thoracic SCI. It causes hypotension and inadequate tissue perfusion due to sudden loss of sympathetic vasomotor function, which leads to severe disruption of the balance between vasodilator and vasoconstrictor functions of the arterioles and venules, hence decreased systemic and vascular resistance and pooling of blood in the peripheries (Denton and McKinlay, 2009). Bradycardia develops and the patient is unable to increase cardiac output by changes in heart rate (Denton and McKinlay, 2009). This shock state leads to ischaemia, which may contribute to secondary injury of the spinal cord (Dumont et al., 2001).
8.2.2.2.2. Spinal shock
Spinal shock is not related to the circulatory system. Depressed spinal reflexes inferior to the level of injury is referred to as spinal shock. Those with mild SCI may not develop spinal shock, whereas those with more severe injury will. The four phases of spinal shock reported in the literature include the following (Ditunno et al., 2004).
8.2.2.2.2.3. Early hyperreflexia. Four days to one month following SCI, deep tendon reflexes return fully for all ages, but the timing of reflex return varies between individual patients. Ankle jerk reflexes precede knee jerk. Babinsky sign appears soon after recovery of the ankle jerk reflex. Less episodes of bradycardia, cardiac arrhythmias and hypotension are observed and episodes of autonomic dysreflexia may begin to emerge in patients with injuries above T6 (see further information in Section 8.2.2.2.3).
During spinal shock, a patient’s neurological deficit may present at a higher spinal level than the original injury due to cord swelling. In the initial period of spinal shock the patient is nursed in a supine position to minimise secondary injury to the spinal cord. Over the course of the following weeks the patient is gradually brought into a head-up position; however, if this position leads to deterioration of the patient’s neurological function, the supine position is resumed. As the swelling eventually subsides, the patient’s deep tendon reflexes will begin to return and this signifies the end of the spinal shock period.
8.2.2.2.3. Autonomic dysreflexia
Partial or complete elimination of supraspinal sympathetic control of cardiovascular functions (e.g. coronary blood flow, cardiac contractility and heart rate) occur with moderate to severe SCI. Autonomic dysreflexia is a potentially life-threatening condition that may occur in patients with SCI above level T6 (Furlan, 2013; Stephenson, 2013), whereby an inciting stimulus (not necessarily noxious) below the level of the lesion causes an uninhibited sympathetic reflex and unbalanced physiological response. This sympathetic over-activity causes vasoconstriction and severe hypertension and is also known as hyperreflexia.
An inciting stimulus (originating most commonly from the bladder and bowel) is generated below the level of the lesion and impulses are sent via the spinal cord to the brain. These impulses are prevented from reaching the brain by the cord injury (Stephenson, 2013). However, the sympathetic nervous system is stimulated by the receptors located at the sympathetic ganglion at T5–T6 and a massive sympathetic response is generated, causing widespread vasoconstriction and peripheral arterial hypertension (Stephenson, 2013). The brain detects this sudden increased blood pressure (BP) through the baroreceptors located in the carotid artery and aortic arch; subsequently the parasympathetic nervous system is stimulated via the vagal nerve. Again the SCI (at T6 or above) prevents the inhibitory effect of the parasympathetic descending impulses below the lesion, which normally would cause peripheral vasodilation (Stephenson, 2013); however, there is a parasympathetic response above the level of the lesion that results in the patient presenting with flushed features. Through the vagal nerve, parasympathetic cardiac responses remain intact as the only supraspinal control of the heart. This leads to the development of heart rate irregularities and cardiac arrhythmias. The brain attempts to lower the peripheral arterial hypertension through slowing the heart rate using stimulation of the vagal nerve; however, this compensatory bradycardia is often unsuccessful and the patient’s hypertensive state continues (Stephenson, 2013). The reflex hypertension only resolves once the inciting stimulus is removed (Furlan, 2013; Stephenson, 2013).
Episodes of autonomic dysreflexia may occur during the sub-acute (recovery from spinal shock) and chronic stages after severe SCI (Furlan, 2013) and have a higher incidence in complete spinal cord lesions (91%) than incomplete lesions (27%) (Stephenson, 2013). Patients with SCI above T6 present with systolic blood pressure (SBP) and diastolic blood pressure (DBP) values well below the norm during the acute stage of injury. Normal SBP for such patients is 90–110 mmHg (Stephenson, 2013). During episodes of autonomic dysreflexia, SBP rises by more than 30 mmHg and DBP by more than 20 mmHg. Therefore it may seem as though the patient’s BP had stabilised to normal values; however, a life-threatening situation has developed (Furlan, 2013; Stephenson, 2013). At the onset of an episode of autonomic dysreflexia, bradycardia or tachycardia develops together with the signs and symptoms summarised in Table 8.4 (Furlan, 2013; Stephenson, 2013).
Preventive strategies for autonomic dysreflexia include proper bowel and bladder care and the prevention of pressure sore formation. Short-acting anti-hypertensive medication may be used to lower BP during an episode of autonomic dysreflexia if non-pharmacological measures (e.g. loosening of tight clothing, unblocking or repositioning the indwelling urinary catheter and removal of faecal impaction) are ineffective (Furlan, 2013; Stephenson, 2013).
Table 8.4:Signs and symptoms associated with an episode of autonomic dysreflexia.
Signs and symptoms | Reason for development |
Headache and seizures | Elevated BP |
Visual disturbances | Elevated BP |
Altered heart rate or rhythm | Vagal nerve response to elevated BP |
Flushing, diaphoresis, and profuse sweating (above the level of the lesion) | Vasodilation in response to elevated BP |
Nasal congestion | Vasodilation in response to elevated BP |
Pallor, cold extremities and piloerection (gooseflesh) (below the level of the lesion) | Sympathetic tone and the lack of descending inhibitory parasympathetic tone |
Chills without fever, muscle spasm, penile erection | Sympathetic tone and the lack of descending inhibitory parasympathetic tone |
Bronchospasm | Increased vagal nerve tone |
The reader is referred to Section 8.11 for further suggested reading on this topic.
8.2.3. Causes and mechanisms of injury in paediatrics
The causes of SCI are similar between adults and children, with motor vehicle accidents and falls being the most common (Mortavazi et al., 2011; Clarke, 2012). Sports injuries are also a common cause of SCI in older children (Mortavazi et al., 2011). Non-accidental injuries and penetrating injuries (such as gunshot and stab wounds) have also been reported in small numbers (Mortavazi et al., 2011), along with other rare causes such as high cervical injuries related to skeletal dysplasias (e.g. achondroplasia), juvenile rheumatoid arthritis and Down’s syndrome or Trisomy 21 (Zidek and Srinivasan, 2003).
Distraction forces that stretch the spinal cord, such as flexion, extension, rotation or dislocation, may cause SCI in children. The mechanism of injury, however, is profoundly different between adults and children. Whereas the mechanism of SCI in adults is most commonly that of vertebral fracture, the most common mechanisms for SCI in children younger than 10 years are vertebral dislocations without fracture and SCI without radiological abnormality (SCIWORA). Children younger than eight years are significantly more likely to sustain SCIWORA than older children and adolescents (Pang and Wilberger, 1982; Clarke, 2012). Spinal cord injury without radiological abnormality presents clinically as an SCI, but plain x-ray shows normal alignment and no vertebral fracture; although magnetic resonance imaging (MRI) may show ligament rupture. It is believed that SCIWORA is caused by vertebral displacement followed by re-alignment with stretching, tearing or contusion of the spinal cord and is extremely rare in the adult population (Pang and Wilberger, 1982; Clarke, 2012).
Factors related to immaturity, such as ligament laxity, shallow horizontal facet joints, underdeveloped spinous processes and relatively weak neck muscles, predispose the child to SCI as a result of increased flexibility under fairly low-loading conditions (Dumont et al., 2001; Mortavazi et al., 2011; Clarke, 2012). The most common level of SCI in children is the cervical spine, with up to 80% of paediatric SCI being cervical (compared to about 55% in adults) (Patel et al., 2001). Younger children more commonly sustain upper cervical spine injury (C2–C3) compared to C4– C5 in adults and adolescents (Patel et al., 2001). This high rate of cervical injury in children is likely a result of increased flexibility in the cervical spine compared to the thoracic and lumbar regions, as well as the relatively large head size in children (Clarke, 2012). Injury patterns approach an adult pattern from eight years of age (Mortavazi et al., 2011).
8.3. Spinal Cord Lesions and Classification of the Level of Injury
8.3.1. Types of spinal cord lesions
The clinical effects of SCI depend on the extent and location of the damage to the spinal cord. The extent of the damage to the spinal cord may result in either a complete or incomplete lesion. The location of the SCI may result in either paraplegia or tetraplegia.
8.3.1.1. Complete lesion of the spinal cord
This type of lesion is characterised by loss of both the motor and sensory abilities of the patient below the neurological level, including the sacral segments S4 and S5 (Kirshblum et al., 2004).
8.3.1.2. Incomplete lesion of the spinal cord
There is preservation of either sensory and/or motor function below the neurological level with an incomplete lesion of the spinal cord. It is now globally agreed that the presence of any sensation in the lower sacral segments of S4–S5 means that the lesion is incomplete. This preservation is a good predictor of improved outcome, especially if there is pinprick sensation 72 hours to one week after injury (Kirshblum et al., 2004).
Syndromes associated with incomplete lesions of the spinal cord are below.
8.3.1.2.1. Anterior cord syndrome
This syndrome occurs due to injury to the anterior two-thirds of the spinal cord. It often results from compression of the anterior spinal artery caused by flexion injuries, direct damage by bone fragments or disc compression. The resultant neurological deficits are usually due to damage of the corticospinal and spinothalamic tracts. The neurological manifestation includes muscle weakness, incomplete sensory loss, loss of sensitivity to pain and temperature below the level of the lesion with preservation of posterior column function (McKinley et al., 2007; Scivoletto and Di Donna, 2009).
8.3.1.2.2. Central cord syndrome
This is the most common of the incomplete traumatic cervical cord syndromes. It is typically seen in older patients with cervical spondylosis and in certain ethnic groups who present with a tight cervical canal (Harrop et al., 2006). In these patients, hyperextension from minor trauma will result in spinal cord compression. This injury can cause ischaemia within the grey matter in the central portion of the cord, but the peripheral area of the cord remains relatively unaffected. The result is that the patient presents with significant loss of function in their upper limbs but relatively preserved function in their lower limbs. Young adults may develop central cord syndrome due to severe spinal column injury from high-energy trauma such as motor vehicle accidents, falls and diving accidents (Harrop et al., 2006). Low-energy traumatic injury that leads to acute central cervical disc herniation may also cause central cord compression (Harrop et al., 2006). The pathogenesis of central cord syndrome suggests the involvement of the lateral white matter columns and corticospinal tracts, which have a great influence on hand function. Patients present with flaccid paralysis of the upper limbs and relatively strong but spastic leg function. This is due to the fact that the cervical tracts are located more centrally within the cord. Sacral sensation and bowel and bladder function are usually partially spared (Harrop et al., 2006; McKinley et al., 2007).
8.3.1.2.3. Posterior cord syndrome
Posterior cord syndrome is a very rare incomplete lesion of the spinal cord. This syndrome is seen in hyperextension injuries and causes contusion of the posterior spinal cord columns. The trauma to the posterior tracts results in loss of proprioception, deep touch and vibration sense. The patient presents with good motor function, pain and temperature sensation, but at times with profound loss of proprioception, which can make walking very difficult and results in an ataxic-type gait (McKinley et al., 2007).
8.3.1.2.4. Brown Sequard syndrome
Brown Sequard syndrome refers to injury in one hemi-section of the spinal cord. It usually occurs in stab or gunshot wound trauma. Contralaterally (opposite the side of the injury), there is a loss of sensitivity to pain, temperature and crude touch below the lesion from interruption of the spinothalamic tracts. The spinothalamic tracts cross or intersect at the level of the spinal cord; hence the hemi-section of the cord preserves pain, temperature and crude touch sensation on the side of the injury.
Ipsilaterally (the same side as the injury), interruption of the corticospinal tracts results in paralysis of voluntary movements below the level of the lesion, spasticity, positive Babinski sign and hyperreflexia. Interruption of the posterior columns results in loss of vibration, form perception, two-point discrimination and proprioception (McKinley et al., 2007; Scivoletto and Di Donna, 2009). Patients who present with more upper limb weakness than lower limb weakness are likely to be able to ambulate before discharge from the hospital (McKinley et al., 2007).
8.3.1.2.5. Compression of the conus medullaris and cauda equina
The spinal cord ends at the level of L1–L2 vertebrae. Compression of the conus medullaris usually results from a compression fracture of L1, which causes contusion and haemorrhage with damage to sacral segments of the cord. Injuries above the conus are predominantly upper motor neuron lesions, although anterior horn cells of lower motor neurons can be damaged at the site of the injury. Damage to the conus can involve both upper and lower motor neurons (McKinley et al., 2007; Lavy et al., 2009).
Simultaneous compression of several lumbar and sacral nerve roots in the lower lumbar region (L4–S1) lead to the development of cauda equina syndrome. Injuries involving the cauda equina are lower motor neuron injuries and will therefore result in flaccid paralysis. The patient also typically presents with neuromuscular and urogenital symptoms such as low back pain, sciatica (usually bilateral), saddle sensory disturbances, bladder and bowel dysfunction and sensory loss in the lower limbs that may differ in severity from one patient to the next (McKinley et al., 2007; Lavy et al., 2009).
8.3.2. Location of injury
8.3.2.1. Paraplegia
Paraplegia refers to damage to spinal cord levels T1 and below. Consequently the resulting paralysis can affect the lower limbs, bladder and bowel function and, depending on the level, the trunk. Thoracic spine injury affects intercostal and abdominal muscle innervation, which impairs ventilation and may lead to respiratory muscle fatigue and failure (refer to Section 8.4) (Kirshblum et al., 2004).
8.3.2.2. Tetraplegia
Tetraplegia refers to loss of motor and/or sensory function as a result of damage to the cervical segment of the spinal cord. There is paralysis of the upper and lower limbs, trunk, bowel and bladder function. As this injury affects both inspiratory and expiratory muscles of respiration and the sympathetic nervous system, it has a greater negative impact on cardiovascular system and respiratory system stability than paraplegia, leading to the development of cardiac arrhythmia, hypotension and respiratory distress and failure (refer to Section 8.4) (Kirshblum et al., 2004).
8.3.3. Classification of the level of injury
In the 1970s a method of classification of SCI was developed by Frankel in the UK. This system classified the patient as having a lesion A, B, C or D. The Frankel classification system caused some confusion among clinicians, and the American Spinal Injury Association (ASIA) refined Frankel’s classification to alleviate this confusion. More and more countries and major trauma centres have adopted the ASIA classification scale for SCI (Ditunno et al., 1994) (Table 8.5). This classification now supersedes the Frankel classification system. The term ‘neurological level’ in the ASIA scale refers to the spinal roots that enter and exit the spinal column between the vertebral bodies. Classification of the level of injury differs between clinicians, with neurologists classifying the level of SCI as the first spinal segmental level with abnormal neurological loss, orthopaedic surgeons classifying it as the level of bony involvement, and rehabilitation specialists classifying it as the lowest spinal segmental level with preserved function.
Table 8.5:The ASIA impairment scale*.
Grade | Description |
A | Complete lesion: no motor or sensory function is preserved in the sacral segments S4–S5 |
B | Incomplete lesion: sensory but not motor function is preserved below the neurological level and includes the sacral segments S4–S5 |
C | Incomplete lesion: motor function is preserved below the neurological level and the majority of key muscles below the neurological level have a muscle grade of less than three |
D | Incomplete lesion: motor function is preserved below the neurological level and at least half of the key muscles below the neurological level have a muscle grade greater than or equal to three |
E | Motor as well as sensory function is normal |
*Adapted from American Spinal Cord Injury Association (2003) and Eng and Chan (2013).
The ASIA includes bilateral assessment of 28 dermatomes using pinprick and light touch sensation and manual muscle testing of 10 key muscles. Total motor and sensory scores are calculated based on these results and are used in combination with anal sphincter sensory and motor function to determine ASIA classification (Eng and Chan, 2013). It is important to note that, in SCI rehabilitation, short-term and long-term goals are established based upon the patient’s neurological level and degree of preserved function below the level of injury.
8.4. Respiratory System Complications following Spinal Cord Injury and Changes in Respiratory Muscle Function
8.4.1. Spinal cord injury and respiratory muscle function
Trauma to the spinal cord results in a loss of motor neurons, interneurons, myelin insulation and ascending and descending long tract axons within the injury zone, which can result in neurological deficits spanning many segments. Depending on the level of the lesion, loss of innervation will lead to paralysis or paresis of respiratory muscles and consequently altered lung mechanics (Tables 8.6 and 8.7). Spinal shock can severely impact the patient’s ability to breathe spontaneously (due to muscle paralysis) and hence might necessitate intubation and mechanical ventilation (MV). Hypoxaemia may lead to secondary injury to the spinal cord and should be avoided at all cost.
Table 8.6:Muscles of respiration*.
Muscle | Innervation | Function related to respiration |
Diaphragm | Phrenic nerve through segmental nerve roots C3–C5 | Primary muscle of inspiration and active during tidal and forced inspiration |
External intercostals | Segmental nerve roots T1–T11 | Primary muscles of inspiration and active during tidal and forced inspiration |
Scalenes | Segmental nerve roots C4–C8 | Accessory muscle of inspiration and only active during forced inspiration to facilitate elevation of the upper rib cage |
Sternocleidomastoid and trapezius | Segmental nerve roots C1–C4 and accessory nerve XI | Accessory muscles of inspiration and only active during forced inspiration to facilitate elevation of the upper rib cage |
External oblique | Segmental nerve roots T6–T12 and subcostal nerve | Forced expiration |
Pectoralis major | Segmental nerves C5–T1 | Forced expiration |
Internal intercostals | Segmental nerve roots T1–T11 | Forced expiration |
Internal oblique and transverse abdominis | Segmental nerve roots T6–T12 | Forced expiration |
Rectus abdominis | Thoracoabdominal nerve through nerve roots T7–T11 | Forced expiration |
*Terson de Paleville et al. (2011).
The function of the respiratory muscles below the level of injury may be permanently impaired as a result of the primary injury; however, the function of the respiratory muscles above the level of injury may be temporarily impaired due to the presence of oedema fluid and blood in the spinal canal, as mentioned in Section 8.2.2.2.2. These patients often require intubation and MV in the intensive care unit (ICU) until the oedema fluid and amount of haemorrhage in the spinal canal has subsided in order for nervous supply to be restored to the muscles that were not affected by the injury. As the swelling in the spinal canal subsides, the patient may be able to resume spontaneous breathing and may eventually be weaned from the ventilator. When muscle paralysis changes to spasticity, respiratory function improves due to the development of chest wall rigidity (Berney et al., 2011; Vásquez et al., 2013). Although MV is life-saving, it does have negative consequences on respiratory function, such as weakening of the preserved muscles. Acquiring a nosocomial lung infection in addition to muscle weakness increases ventilation time and ICU length of stay and eventually leads to difficulty in weaning patients from MV.
Table 8.7:Level of spinal cord injury and its effects on respiratory muscle function and vital capacity*.
Level | Effect on respiratory muscles | Vital capacity in acute stage |
C1–C2 | •Partial innervation of accessory inspiratory muscles •Likely to be ventilator-dependent | 5–10% of normal (500–600 ml) |
C3–C6 | •Innervation of accessory inspiratory muscles •Partial innervation of primary inspiratory muscles | 20–30% of normal (1 litre) |
C7–T4 | •Innervation of sternocleidomastoid, trapezius and scalene muscles •Innervation of diaphragm •Partial innervation of intercostal muscles | 30–50% of normal (1.4–2.3 litres) |
T5–T10 | •Innervation of primary and accessory inspiratory muscles •Partial innervation of intercostal muscles •Partial innervation of expiratory muscles | 75–100% of normal (4–5 litres) |
8.4.2. Respiratory complications following spinal cord injury
Respiratory complications are a leading cause of morbidity and mortality in patients with SCI, being more severe in patients with high-level and complete injuries (Cotton et al., 2005; Brown et al., 2006; Shavelle et al., 2006; Kawu et al., 2011; Vásquez et al., 2013). Serious complications such as pneumonia, atelectasis and ventilatory failure are experienced by up to 83% of patients with cervical lesions and 50% of patients with high-thoracic spinal cord lesions (T1–T6) in the acute phase (first five days) of injury (Berlly and Shem, 2007; Berney et al., 2011; Terson de Paleville et al., 2011). The level of SCI and the degree of motor function loss correlates directly with the development of respiratory complications. Age, pre-existing medical illness and smoking history may be additional predisposing factors to the development of respiratory complications over the first five days following SCI (Berlly and Shem, 2007). Intensive chest physiotherapy management during this acute phase after injury is advocated to prevent the development of respiratory complications (Berney et al., 2011).
8.4.2.1. Complications encountered in the early phase after spinal cord injury
Respiratory complications encountered by patients in the acute stage after SCI include the overproduction of pulmonary secretions, bronchospasm, atelectasis, ventilator-associated pneumonia, pulmonary oedema or respiratory failure.
8.4.2.1.1. Overproduction of pulmonary secretions
Hypersecretion of mucus after SCI is thought to be caused by the loss of sympathetic control and unopposed vagal activity in the first few weeks after injury. Overproduction of secretions is worsened by pre-existing factors such as smoking, chronic obstructive pulmonary disease, asthma and ageing (Berlly and Shem, 2007; Sheel et al., 2008). Mucus hypersecretion can occur within one hour after cervical SCI and goes hand-in-hand with changes in the chemical content of mucus. Tenacious secretions lead to the formation of mucus plugs in the lung periphery. Weakness or paralysis of the muscles of inspiration and forced expiration leads to the generation of inefficient inspiratory volumes and poor peak expiratory flow rate and results in a poor cough effort. The presence of an additional chest wall injury, in patients with thoracic SCI, may further impair the patient’s ability to cough and clear retained secretions effectively (Berlly and Shem, 2007). All of these factors contribute to the development of atelectasis or pneumonia and subsequent respiratory failure. The chemical content of mucus returns to normal only months after injury (Berlly and Shem, 2007).
8.4.2.1.2. Bronchospasm
Patients with cervical SCI often suffer from bronchospasm, even in the absence of a history of asthma, due to autonomic nervous system dysfunction seen in the acute phase after injury. Resting airway tone is increased due to the interruption of sympathetic nervous system supply to the lungs in the presence of intact parasympathetic nervous system activity, as mentioned previously. Bronchospasm contributes to secretion retention and respiratory distress. Therefore the patient will be in need of bronchodilator therapy before physiotherapy treatment is initiated during the acute phase (Berlly and Shem, 2007; Vásquez et al., 2013).
8.4.2.1.3. Atelectasis
Atelectasis may occur due to poor lung expansion as a result of weak or paralysed inspiratory muscles, retained bronchial secretions, weak cough effort, cephalad movement of abdominal content and decreased production of surfactant in lung segments that have lost volume. These changes can also lead to the development of pneumonia and respiratory failure (Berlly and Shem, 2007).
8.4.2.1.4. Respiratory failure
Respiratory complications after cervical SCI include hypoventilation, hypercapnia, reduction in surfactant production, atelectasis, increased work of breathing and respiratory muscle fatigue (Wong et al., 2012). Patients with lesions above C3 are at particular risk for the development of respiratory failure. Patients with SCI at level C3 or below may be able to breathe spontaneously following the injury, but evidence shows that respiratory failure occurs in some of these patients by the fourth day after hospital admission and may last for up to five weeks (Wong et al., 2012). Risk factors for the development of respiratory failure have been mentioned in the previous paragraphs and are summarised in Table 8.8.
Table 8.8:Risk factors for the development of respiratory failure in patients with spinal cord injury.
Spinal shock Decreased lung expansion due to impaired innervation of the inspiratory muscles Impaired cough effort due to impaired innervation of the expiratory muscles Increased secretion production Decreased surfactant production resulting in lung volume loss |
Respiratory failure has a direct influence on the patient’s mortality and therefore patients with cervical or thoracic SCI (especially above T6) should be closely monitored for signs of respiratory distress, which is a precursor to respiratory failure, in the acute phase after injury.
8.4.2.1.5. Ventilator-associated pneumonia
Similar to other patients who are intubated and mechanically ventilated, patients with SCI are at risk of developing ventilator-associated pneumonia (VAP) due to the presence of the endotracheal tube (ETT) and impaired mucociliary function and cough ability (Mietto et al., 2013). Ventilator-associated pneumonia is associated with increased mortality, hospital length of stay and health care cost (Mietto et al., 2013). Patients with SCI and weaning and extubation failure face a high risk for the development of VAP (Call et al., 2011).
8.4.2.1.6. Pulmonary oedema
Up to 50% of patients with tetraplegia present with pulmonary oedema during the acute stage following injury (Berlly and Shem, 2007). Pulmonary oedema may arise due to a variety of factors, such as direct trauma to the lung tissue, acute respiratory distress syndrome and fluid overload, or may be neurogenic in origin (Berlly and Shem, 2007). Overhydration may occur in instances in which low BP is misinterpreted as traumatic hypovolaemic shock (instead of a normal feature of spinal shock) and treatment with fluid resuscitation is erroneously performed. Careful fluid resuscitation is important to avoid overhydration, particularly in the polytrauma patient, where a balance between treatment for hypovolaemia and the natural reduction in BP after SCI is vital.
The information shared in this section highlights the need for early respiratory treatment provided by physiotherapists, the main aim being the prevention of atelectasis and respiratory infections (Vásquez et al., 2013). It has been shown that comprehensive clinical pathways and structured respiratory protocols reduce respiratory complications in patients with tetraplegia. It limits cost to the patient and hospital as it plays a role in altering the patient’s need for intubation and MV (Berney et al., 2011). The reader is referred to Section 8.9 for detailed information on physiotherapy intervention strategies that should be used in the management of patients with SCI in the acute phase after injury. Section 8.11 suggests further reading on clinical practice guidelines for the respiratory management of patients with SCI.
8.4.2.2. Complications encountered in the later stages following spinal cord injury
In the later stages following SCI, patients develop changes in respiratory pattern, chest wall and lung compliance, lung capacity and lung volumes, and may also develop aspiration pneumonia or pulmonary thromboembolism.
8.4.2.2.1. Changes in respiratory pattern
Anatomically, the diaphragm is positioned in apposition (side-by-side) to the rib cage. The area extending from the origin of the diaphragm on the rib cage to the dome of the diaphragm is referred to as the zone of apposition. During diaphragmatic contraction in individuals without SCI, intraabdominal pressure rises as the dome of the diaphragm moves caudally and assists with the elevation of the rib cage through the zone of apposition (Brown et al., 2006). In patients with cervical SCI, however, the abdomen is highly compliant, which leads to no or minimal increase in intra-abdominal pressure at the onset of inhalation. The lack of increased intra-abdominal pressure causes the lower ribs to be pulled inwards as the orientation of the diaphragm fibres is not conducive towards the elevation of these ribs (Brown et al., 2006). The reader is referred to Sections 8.4.2.2.3 and 8.9.1.1 for information on the effect of body positioning on respiration.
In cervical SCI, during spontaneous inhalation the upper anterior chest wall moves inwards when the diaphragm contracts due to the lack of innervation of the external intercostal muscles. This gives rise to an abnormal breathing pattern commonly called ‘paradoxical’ breathing, which is isolated diaphragmatic action with retraction of the chest wall (Berlly and Shem, 2007).
A patient with cervical SCI and diaphragm weakness may suffer from episodes of breathlessness when spastic contractions of the abdominal muscles occur. During spastic abdominal muscle contraction, gastric and oesophageal pressures increase. This increase in pressure needs to be overcome by the patient for inspiration to take place; hence resulting in breathlessness (Terson de Pavilles et al., 2011).
8.4.2.2.2. Changes in compliance
Within one month following SCI, reductions in lung compliance is observed. The exact cause of reduced lung compliance in this early stage after injury is unknown. It is suggested that reductions in lung volumes due to respiratory muscle weakness and changes in the mechanical properties of the lung from alterations in surfactant production in areas of atelectasis may be contributing factors to the reduction in lung compliance (Brown et al., 2006; Harvey, 2008).
A reduction in chest wall compliance is observed in patients with tetraplegia due to the rigidity of the chest wall from muscle spasticity. Articular changes occur over time at the costosternal and costovertebral joints due to poor inspiratory muscle performance, which restricts the stretching of the chest wall to the level of total lung capacity (TLC) (Brown et al., 2006; Harvey, 2008).
8.4.2.2.3. Changes in lung capacity and lung volumes
Muscle weakness or paralysis and spastic muscle contractions have a significant impact on lung capacity (functional residual capacity (FRC), TLC, vital capacity (VC) and forced vital capacity (FVC)) and lung volume (expiratory reserve volume (ERV), forced expiratory volume in one second (FEV1), tidal volume (TV) and residual volume (RV)) following SCI. The higher the lesion, the more severely it affects lung capacity and lung volumes (Baydur et al., 2001; Terson de Paleville et al., 2011).
Patients with complete cervical lesions below C2 experience decreases in VC of 20–50% of predicted values due to changes in lung compliance and impairments in inspiratory and expiratory muscle function (Brown et al., 2006; Berlly and Shem, 2007). This drastic reduction in VC leads to inefficient ventilation and poor cough ability. Gaseous exchange is affected by reductions in VC, TLC and VT as hypoventilation leads to carbon dioxide retention and hypoxaemia (Harvey, 2008). Reductions in mean values for FEV1 and FVC to 40–50% of predicted values have also been reported (Brown et al., 2006). Over time, there seems to be an improvement in VC, FEV1 and FVC values by approximately 10–15% as a result of improved diaphragmatic efficiency (Brown et al., 2006).
Changes in body positioning influence lung volume and lung capacity in patients with tetraplegia. When a person without SCI moves from a seated to supine position, VC reduces by approximately 7% and inspiratory capacity (IC) increases by approximately 55%. These changes in lung capacity are attributed to fluid shifts in and out of the thorax, resulting in a larger intrathoracic volume in the supine position (Baydur et al., 2001). In tetraplegia, movement into the supine position leads to increases in VC and IC, not only because of increased intrathoracic volume but also due to the effect of gravity on the abdominal contents (Baydur et al., 2001). This explains why patients with tetraplegia report less breathlessness and greater ability to cough without an abdominal binder in a supine position compared to seated position.
8.4.2.2.4. Aspiration pneumonia
Patients with SCI are at high risk for developing aspiration pneumonia (Chin, 2014). Table 8.9 lists the risk factors for aspiration pneumonia in this patient population (Paralyzed Veterans of America, 2005).
The reader is referred to Section 8.6.3.3.9 for information on preventive strategies used for aspiration pneumonia.
Table 8.9:Risk factors for the development of aspiration pneumonia following spinal cord injury.
Spinal shock Supine position Cervical spinal cord injury with dysphagia Slowing of gastro-intestinal tract function, e.g. development of paralytic ileus Nausea and vomiting Neck swelling from recent anterior cervical spine surgery Impaired cognitive state Poor cough effort or inability to expectorate secretions Presence of a tracheostomy |
8.4.2.2.5. Pulmonary thromboembolism and deep venous thrombosis
Patients with SCI have a three times higher risk for the development of pulmonary embolism and deep venous thrombosis than the general population from 72 hours following injury onwards (Miranda and Hassouna, 2000; Berlly and Shem, 2007). Pulmonary embolism develops in approximately 5% of patients with SCI and deep venous thrombosis in 15% of these patients (McKinney, 2013). Deep venous thrombosis develops as early as 72 hours following injury and the risk remains high until two weeks after SCI (McKinney, 2013).
Possible explanations offered for this increased incidence of thrombosis include metabolic changes in blood vessels due to the interruption of neurologic impulses and resultant paralysis, decreased venous distensibility and increased resistance to venous blood flow, sluggish vasomotor tone and, lastly, vascular adaptations to inactivity and muscle atrophy (Miranda and Hassouna, 2000; McKinney, 2013). Prophylactic therapy in the form of low molecular weight heparin and the use of intermittent pneumatic compression devices as precautionary methods are recommended for a minimum of eight weeks following SCI (Berlly and Shem, 2007). Regular screening of the patient for sudden onset of shortness of breath, difficulty breathing and hypoxia or limb pain, limb swelling, limb tenderness, limb discolouration and increased skin temperature is of vital importance.
8.4.3. Recovery of respiratory function following spinal cord injury
Respiratory function improves spontaneously in patients with low level tetraplegia or paraplegia within the first year after injury, and only a small percentage of patients require further ventilatory support. This improvement is ascribed to small improvements in general motor function. Very little improvement is noted after the first year, and in some cases loss of function is even observed (Zimmer et al., 2007). Breathlessness is a symptom that many patients with chronic SCI report and seems to be more prominent in patients with tetraplegia than in those with paraplegia. Environmental factors, such as exposure to hot air, or lifestyle choices, e.g. exposure to direct or second-hand smoke and increased body mass index, may increase the sensation of breathlessness in tetraplegic patients (Stepp et al., 2008; Schilero et al., 2009). Increased body mass index leads to decreased TLC, FRC and RV. Patients with thoracic SCI between T1 and T6 have permanently altered lung mechanics, but have a greater chance of recovering from future lung infections than those with tetraplegia. In those with thoracic lesions below T6, lung mechanics are less altered and their ability to recover from future lung infections is greater.
8.4.4. Spinal cord injury not associated with respiratory compromise
Not all SCIs result in respiratory function abnormalities. Patients with central cord syndrome present with minimal to no respiratory system involvement if they are mobilised early during their admission. Posterior cord syndrome does not affect the respiratory system much and hence chest complications are rare. The effects of Brown Sequard syndrome on the chest are minimal, and if the patient does not develop other complications they are unlikely to develop respiratory complications. If a patient with compression of the conus medullaris and cauda equina is mobile, there should not be any complications concerning the respiratory system. Early mobilisation, in patients with stable SCI, or prophylatic chest physiotherapy should help prevent any respiratory system complications.
Table 8.10:Bodily system complications secondary to spinal cord injury.
Bodily system | Complications |
Digestive system and gastro-intestinal tract | •Cholecystitis •Upper gastro-intestinal tract bleeding •Stool incontinence •Constipation •Haemorrhoids |
Integumentary system | •Pressure ulcers |
Musculoskeletal system | •Muscle weakness •Atrophy •Spasticity •Osteoporosis •Hypercalcemia |
Psychological | •Pain •Depression |
Renal and urinary systems | •Hydronephrosis •Urinary tract infections |
Reproductive system | •Male infertility •Erectile dysfunction |
Vascular system | •Thromboembolism |
8.5. Complications Related to Other Bodily Systems following Spinal Cord Injury
Patients with SCI are at a higher risk of developing complications related to the integumentary, musculoskeletal, vascular, gastrointestinal, digestive and renal systems. A short summary of complications commonly reported are listed in Table 8.10 (Wuermser et al., 2007; Rahimi-Movaghar et al., 2013). The development of any one of these complications increases patient morbidity and hospital length of stay and therefore high quality care should be provided by the interdisciplinary team members to any patient with SCI to avoid its development as far as possible.
8.6. Medical and Surgical Management
On admission to the emergency department, the patient with suspected acute SCI undergoes assessment through use of primary and secondary survey procedures; thereafter, definitive care is provided.
Key Message
At the scene of the accident, a patient with suspected SCI undergoes triple immobilisation. This involves placing the patient in a hard cervical collar, head restraints with sand bags and strapping to a long spinal board. The patient is kept in a flat supine position and log rolled, when necessary, until the spine is cleared of fractures through clinical and radiological investigations in the emergency department.
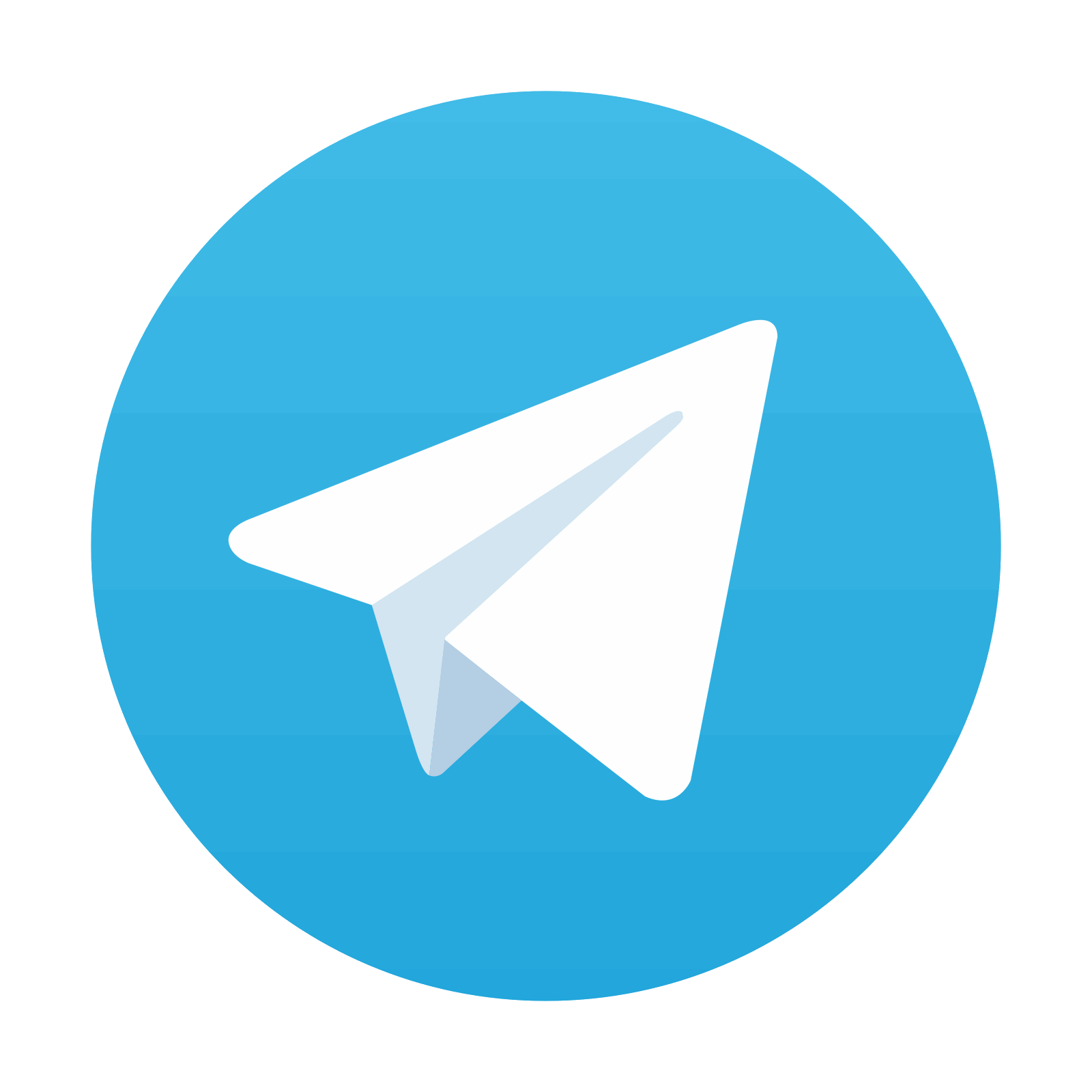
Stay updated, free articles. Join our Telegram channel

Full access? Get Clinical Tree
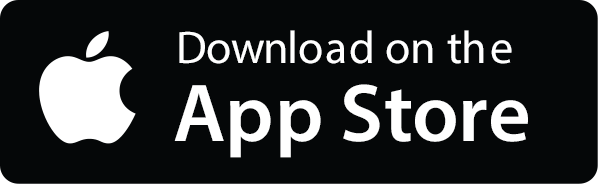
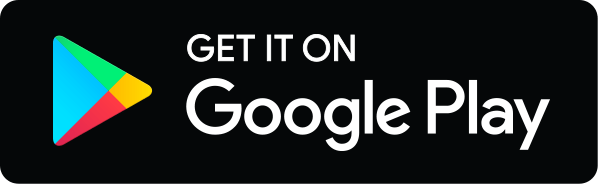