Background
Identification of the extent of scar transmurality in chronic ischemic heart disease is important because it correlates with viability. The aim of this retrospective study was to evaluate whether layer-specific two-dimensional speckle-tracking echocardiography allows distinction of scar presence and transmurality.
Methods
A total of 70 subjects, 49 with chronic ischemic cardiomyopathy and 21 healthy subjects, underwent two-dimensional speckle-tracking echocardiography and late gadolinium-enhanced cardiac magnetic resonance. Scar extent was determined as the relative amount of hyperenhancement using late gadolinium-enhanced cardiac magnetic resonance in an 18-segment model (0% hyperenhancement = normal; 1%–50% = subendocardial scar; 51%–100% = transmural scar). In the same 18-segment model, peak systolic circumferential strain and longitudinal strain were calculated separately for the endocardial and epicardial layers as well as the full-wall myocardial thickness.
Results
All strain parameters showed cutoff values (area under the curve > 0.69) that allowed the discrimination of normal versus scar segments but not of transmural versus subendocardial scars. This was true for all strain parameters analyzed, without differences in efficacy between longitudinal and circumferential strain and subendocardial, subepicardial, and full-wall-thickness strain values. Circumferential and longitudinal strain in normal segments showed transmural and basoapical gradients (greatest values at the subendocardial layer and apex). In segments with scar, transmural gradient was maintained, whereas basoapical gradient was lost because the reduction of strain values in the presence of the scar was greater at the apex.
Conclusions
The two-dimensional speckle-tracking echocardiographic values distinguish scar presence but not transmurality; thus, they are not useful predictors of scar segment viability. It remains unclear why there is a greater strain value reduction in the presence of a scar at the apical level.
Highlights
- •
Transmural extent of scar in ischemic myocardial dysfunction is very important.
- •
Strain echocardiography distinguishes the presence of scar, not transmurality.
- •
In normal segments there are transmural and basoapical gradients of strain values.
- •
In scar segments, transmural but not basoapical gradient of strain is maintained.
- •
Reduction of strain values in the presence of scar is greater at apex than at base.
Anatomic and functional differences among layers of the left ventricular (LV) wall are seen even in normal myocardium. It is evident from histologic analysis that many diseases affect myocardial layers to different extents. Any technique that allows a layer-specific analysis of myocardial function is likely to increase morphologic and pathophysiologic understanding of myocardial diseases, particularly ischemic heart disease. A subendocardial infarct causes an area of ischemic necrosis limited to the inner one third or one half of the ventricular wall. In the chronic phase, this necrosis develops into a subendocardial scar (SES). Conversely, in a transmural infarct, necrosis extends through the entire thickness of the myocardium to produce a transmural scar (TMS). Differentiating TMS from SES is clinically important because the identification of SES implies that viable myocardium is present, which may benefit from revascularization.
Late gadolinium-enhanced (LGE) cardiac magnetic resonance (CMR) is the gold standard for the detection and sizing of myocardial scars as well as the identification of both SES and TMS. Two-dimensional (2D) speckle-tracking echocardiography (STE) is a technique that is used to detect and quantify myocardial deformation by strain, a measure of myocardial deformation as a percentage change in myocardial segment length.
Previous studies have suggested that 2D STE can differentiate TMS from SES, but with modest accuracy. Previously described techniques for analyzing myocardial deformation parameters considered the total myocardial wall thickness. In the past few years, the evolution of previously validated 2D speckle-tracking echocardiographic analysis has been described for echocardiography, which enables the separate quantification of deformation of the subendocardial and subepicardial myocardial layers. Some studies have found that this recent layer-specific 2D speckle-tracking echocardiographic analysis appears more accurate than total wall thickness analysis for differentiating SES from TMS. However, few studies have been published on this topic with small numbers of patients and with results that are not totally comparable and frequently discordant.
The aim of this study was to evaluate whether 2D speckle-tracking echocardiographic analysis can discriminate between normal myocardium and scars and between SES and TMS and evaluate any differences in discriminatory accuracy when comparing longitudinal strain for the subendocardial layer (LSEN) or circumferential strain for the subendocardial layer (CSEN) and longitudinal strain for the subepicardial layer (LSEP) or circumferential strain for the subepicardial layer (CSEP) layer-specific and full-wall-thickness strain as well as comparing longitudinal strain (LS) and circumferential strain (CS). We compared 2D STE with LGE CMR.
Then, because the structure of the left ventricle presents anatomic, functional, and electric differences both across the wall and along the ventricle from the basal to the apical level, we also tested for differences in the accuracy of 2D STE when detecting scars among the basal, midventricular, and apical levels.
Methods
Study Population
This retrospective study consisted of 70 subjects (mean age, 62.4 ± 13.3 years; 49 men) who underwent layer-specific 2D STE and LGE CMR at our institution.
We excluded patients in whom the interval between 2D STE and LGE CMR exceeded 6 months. Most of the examinations were done with a time interval of slightly less than 3 months between 2D STE and LGE CMR, and the median time between the examinations was 73 days.
Forty-nine patients were affected by chronic ischemic heart disease, while 21 control subjects with normal values on 2D STE and LGE CMR and without any history of ischemia were selected on the basis of normal LGE CMR reports collected at our institution. LGE CMR examination of control subjects was prescribed for different clinical reasons but mainly for research of the foci of fibrosis or dysplasia in healthy subjects with unexplained ventricular extrasystole or arrhythmia.
Absence of sinus rhythm as well as myocardial infarction or percutaneous coronary revascularization between 2D STE and LGE CMR or <3 months before the first examination were exclusion criteria. We also excluded patients with suboptimal echocardiographic views.
Echocardiography
Echocardiography was performed using a Vivid E9 System (GE Vingmed Ultrasound AS, Horten, Norway) equipped with a 5–4.6 MHz transducer active-matrix single-crystal phased array (M5S). Standard parasternal long-axis and short-axis views at the basal, midventricular, and apical levels and in the apical four-, three-, and two-chamber views were acquired with a frame rate of 56 to 92 frames/sec using tissue harmonic imaging.
Speckle-Tracking Analysis
Echocardiographic images from the apical and parasternal short-axis views (basal, midventricular, and apical levels) were stored in digital cine loop format. Peak negative end-systolic LS and CS from the LSEN/CSEN, LSEP/CSEP, and full wall thickness were assessed using an offline software package (EchoPAC version BT11; GE Vingmed Ultrasound AS). The analysis of myocardial deformation according to strain was evaluated on a frame-by-frame basis by automatic tracking of acoustic markers (speckles) that are identified and followed within consecutive 2D echocardiographic images and are expected to change their positions in accordance with the surrounding tissue motion. Loops of three consecutive cardiac cycles were acquired, and deformation parameters were determined as an average of the three loops.
The system automatically determines tracking quality, and segments with suboptimal tracking quality were excluded from the analysis. The endocardial and epicardial borders were automatically traced in the end-systolic frame of the 2D images from the four-, three-, and two-chamber apical views for the analysis of LS and from the parasternal short-axis views for the analysis of CS. In cases of disagreement by the observer regarding the automatically defined borders, manual correction was applied for endocardial-like epicardial borders. Segments with insufficient quality despite manual correction were excluded.
The system allowed separate analysis of CS and LS peak end-systolic and peak postsystolic strains of the LSEN/CSEN, LSEP/CSEP, and full wall thickness outlined by the tracked borders of each LV segment.
The measurements presented for the LSEN/CSEN and LSEP/CSEP layers are the values at the inner- and outer-tracked region-of-interest lines, which represent the endocardial and epicardial border, respectively. Thus, according to the definition of endocardial and epicardial contours, the layers were automatically defined by the system.
End-systole was defined as aortic valve closure in the apical axis view and transferred to all other views. Peak end-systolic strain is the peak before or at the time of aortic valve closure, while peak postsystolic strain is peak systolic strain independent of aortic valve closure.
All views were analyzed using the system to obtain quantitative function parameters for each segment in an 18-segment LV model (six segments at each LV level). Segmental speckle-tracking echocardiographic data were matched with CMR data using the same 18-segment model.
Late Gadolinium-Enhanced Cardiac Magnetic Resonance
All patients were examined in the supine position using a 3-T scanner (Magnetom Skyra; Siemens Healthcare, Erlangen, Germany) with a 32-channel phased-array coil while holding their breath.
After localization, cine sequences were acquired using balanced steady-state free precession sequences with long- and short-axis images and projections comparable with those used in cardiac ultrasonography. Short-axis acquisitions were performed using multiple slices to cover both ventricles entirely from the base to the apex (field of view, 350 × 295; matrix size, 216 × 256; acceleration factor, 2 generalized autocalibrating partial parallel acquisition; slice thickness, 8 mm; interslice gap, 20%; number of phases, 25). To evaluate myocardial scars, late enhancement acquisitions (2D phase-sensitive segmented, inversion recovery gradient echo) were performed 10 min after an intravenous bolus injection of 0.2 mmol/kg gadolinium-based contrast medium. Positive hyperenhancement was visually defined as >2 SDs of the signal intensity of the nonenhanced myocardium.
Short- and long-axis slices (8 mm) were acquired on a prospective electrocardiographically gated gradient-echo sequence with inversion prepulse using the same slices of cine sequences (field of view, 330 × 330; matrix size, 192 × 256; acceleration factor, 2 generalized autocalibrating partial parallel acquisition; slice thickness, 8 mm; interslice gap, 20%; inversion time set according to scout images). To evaluate the scar extent, the same 18-segment LV model used for the echocardiographic analysis was applied. To align the echocardiographic and LGE CMR segments, anatomic landmarks (i.e., the papillary muscles and LV outflow tract orientation) were used. Each myocardial segment was evaluated for the presence of hyperenhancement; 0% hyperenhancement was considered to be normal, 1% to 50% hyperenhancement was considered to reflect SES, and 51% to 100% hyperenhancement was considered to reflect TMS.
We differentiated TMS from SES using a 50% cutoff value for the extent of TMS because the recovery of scar segments after revascularization above this percentage is poor.
Statistical Analysis
Computations were performed using Stata version 14.1 (StataCorp, College Station, TX); a two-tailed P value < .05 was considered to indicate statistical significance. Data are expressed as mean ± SD if continuous and as counts and percentages if categorical. Comparisons between myocardial scar groups were performed using linear regression models with Huber-White robust standard errors to account for heteroscedasticity. The unit of analysis was the patient or the segment. In the latter case, regression models included calculation of Huber-White robust standard errors while clustering on the patient to account for intrapatient measurement correlations. The mean difference and 95% CI were derived from the model. The following groups were compared: (1) no scar versus any scar, followed by post hoc comparisons of (1a) no scar versus SES and (1b) no scar versus TMS, and (2) SES versus TMS. Receiver operating characteristic (ROC) curve analysis was used to identify the optimal cutoff for the type of scar that maximizes sensitivity and specificity. The area under the ROC curve (AUC) was used as an overall measure of discrimination. The closer the AUC to 1, the greater the discrimination; an AUC of 0.50 corresponds to no discrimination.
Results
Patient Characteristics
The study population consisted of 70 subjects (mean age, 62.4 ± 13.3 years; 49 men; 21 healthy control subjects). The mean ejection fraction defined by biplane echocardiography was 51.2 ± 13.1%, and the mean ejection fraction defined by CMR was 50.8 ± 14.6%. All patients were in sinus rhythm at the time of the examinations (mean heart rate, 66.6 ± 13 beats/min). Scar was visible on LGE CMR images in 49 patients (SES in 24 [55.9%], TMS in 19 [44.2%], and both SES and TMS in six [8.6%]). A total of 21 patients without LGE CMR findings of scar and histories of ischemic heart disease as described in our database were considered the control group. The mean age of normal control subjects was 54.5 ± 12.1 years, 55.0% were men, 30.0% had histories of hypertension, and none had histories of diabetes, renal failure, or myocardial infarction or had undergone percutaneous coronary intervention. The patients affected by chronic ischemic heart disease had a mean age of 65.4 ± 9.7 years, 81.6% were men, 16.3% had diabetes, 79.6% had histories of hypertension, and none had histories of renal failure. The baseline clinical, echocardiographic, and LGE CMR data of the 70 subjects are shown in Table 1 .
Variable | Value |
---|---|
Age (y) | 62.4 ± 13.3 |
Male | 49 (70%) |
Female | 21 (30%) |
Weight (kg) | 76.8 ± 12.9 |
Height (cm) | 167.9 ± 21.8 |
BMI (kg/m 2 ) | 26.5 ± 4.1 |
BSA (m 2 ) | 1.9 ± 0.2 |
Heart rate (beats/min) | 66.6 ± 13.0 |
History of PCI | 32 (45.7%) |
History of bypass | 8 (11.4%) |
Hypertension | 46 (65.7%) |
Diabetes | 9 (12.9%) |
Chronic renal failure | 5 (7.1%) |
Ejection fraction (%) (echocardiography) | 51.2 ± 13.1 |
Ejection fraction (%) (CMR) | 50.8 ± 14.6 |
SES (LGE CMR) | 24 (55.9%) |
TMS (LGE CMR) | 19 (44.2%) |
SES+TMS (LGE CMR) | 6 (8.6%) |
Absence of scar (LGE CMR) | 21 (30%) |
Cardiac Magnetic Resonance
In total, 1,260 segments were analyzed: 1,036 normal, 116 with SES (1%–50% hyperenhancement), and 108 with TMS (51%–100% hyperenhancement). The distribution of segments with scar (SES and TMS) was as follows: 79 apical (37 SES, 42 TMS), 80 midventricular (40 SES, 40 TMS), and 65 basal (39 SES, 26 TMS).
Normal Myocardial versus Scar Segments
The analysis included 1,020 normal and 219 scar (113 SES, 106 TMS) myocardial segments for LS and 830 normal and 171 scar (91 SES, 80 TMS) segments for CS. Twenty-one segments (2%) for LS and 259 segments (21%) for CS were excluded because of suboptimal echocardiographic tracking quality.
In Tables 2 and 3 , peak end-systolic ( Table 2 ) and peak postsystolic ( Table 3 ) LS and CS values of normal segments are compared with those of scar segments (SES and TMS).
Parameter | Level | No scar, mean ± SD | Scar (SES and TMS), mean ± SD | Difference | P | Cutoff | AUC | Sensitivity | Specificity |
---|---|---|---|---|---|---|---|---|---|
CS full wall thickness | −19.2 ± 8.7% | −9.4 ± 6.3% | 9.2 | <.001 | −13.9% | 0.73 | 0.75 | 0.71 | |
Basal | −16.6 ± 7.5% | −9.6 ± 5.4% | 5.9 | <.001 | −13.3% | 0.71 | 0.77 | 0.65 | |
Midventricular | −18.1 ± 7.6% | −9.3 ± 6.2% | 8.1 | <.001 | −15.4% | 0.71 | 0.81 | 0.61 | |
Apical | −23.9 ± 9.5% | −9.4 ± 7.5% | 14.9 | <.001 | −16.8% | 0.84 | 0.88 | 0.80 | |
CSEP | −14.3 ± 7.6% | −6.8 ± 4.8% | 6.8 | <.001 | −10.7% | 0.74 | 0.81 | 0.67 | |
Basal | −12.4 ± 8.0% | −6.7 ± 4.5% | 4.3 | <.001 | −10.1% | 0.71 | 0.81 | 0.62 | |
Midventricular | −13.1 ± 6.3% | −6.6 ± 4.6% | 5.7 | <.001 | −9.1% | 0.70 | 0.71 | 0.70 | |
Apical | −18.0 ± 7.4% | −6.9 ± 5.4% | 11.4 | <.001 | −12.1% | 0.84 | 0.86 | 0.81 | |
CSEN | −26.8 ± 12.0% | −13.4 ± 9.1% | 12.8 | <.001 | −17.6% | 0.73 | 0.68 | 0.78 | |
Basal | −22.8 ± 9.6% | −13.5 ± 7.4% | 8.4 | <.001 | −14.9% | 0.72 | 0.66 | 0.78 | |
Midventricular | −25.3 ± 10.2% | −13.2 ± 9.0% | 11.4 | <.001 | −21.1% | 0.73 | 0.81 | 0.65 | |
Apical | −33.2 ± 13.9% | −13.7 ± 11.2% | 20.0 | <.001 | −25.7% | 0.81 | 0.86 | 0.75 | |
LS full wall thickness | −16.6 ± 6.6% | −8.9 ± 6.0% | 8.3 | <.001 | −13.5% | 0.75 | 0.79 | 0.70 | |
Basal | −13.7 ± 4.8% | −8.7 ± 5.7% | 6.2 | <.001 | −13.2% | 0.71 | 0.84 | 0.57 | |
Midventricular | −15.5 ± 5.1% | −9.1 ± 5.6% | 6.5 | <.001 | −13.0% | 0.76 | 0.78 | 0.73 | |
Apical | −20.6 ± 7.5% | −9.0 ± 6.7% | 11.9 | <.001 | −14.4% | 0.83 | 0.84 | 0.82 | |
LSEP | −14.1 ± 5.4% | −7.4 ± 5.3% | 7.1 | <.001 | −11.3% | 0.76 | 0.79 | 0.72 | |
Basal | −13.0 ± 4.8% | −7.8 ± 5.4% | 6.0 | <.001 | −11.3% | 0.72 | 0.77 | 0.67 | |
Midventricular | −14.0 ± 4.9% | −8.0 ± 5.1% | 6.0 | <.001 | −11.1% | 0.75 | 0.77 | 0.74 | |
Apical | −15.4 ± 6.3% | −6.5 ± 5.2% | 9.1 | <.001 | −9.6% | 0.82 | 0.79 | 0.84 | |
LSEN | −19.4 ± 9.5% | −10.5 ± 7.9% | 10.0 | <.001 | −14.9% | 0.71 | 0.73 | 0.69 | |
Basal | −14.3 ± 5.5% | −9.5 ± 6.3% | 6.4 | <.001 | −13.0% | 0.68 | 0.73 | 0.62 | |
Midventricular | −17.0 ± 6.1% | −10.0 ± 6.5% | 7.1 | <.001 | −14.8% | 0.76 | 0.81 | 0.70 | |
Apical | −27.3 ± 10.5% | −11.8 ± 9.9% | 15.9 | <.001 | −18.6% | 0.81 | 0.78 | 0.83 |
Parameter | Level | No scar, mean ± SD | Scar (SES and TMS), mean ± SD | Difference | P | Cutoff | AUC | Sensitivity | Specificity |
---|---|---|---|---|---|---|---|---|---|
CS full wall thickness | −19.7 ± 8.5% | −10.3 ± 6.2% | 8.9 | <.001 | −14.9% | 0.73 | 0.77 | 0.70 | |
Basal | −17.0 ± 7.4% | −10.9 ± 5.2% | 5.9 | <.001 | −13.2% | 0.72 | 0.77 | 0.68 | |
Midventricular | −18.6 ± 7.7% | −10.3 ± 5.9% | 7.6 | <.001 | −15.7% | 0.73 | 0.81 | 0.64 | |
Apical | −24.4 ± 9.0% | −10.4 ± 7.8% | 14.4 | <.001 | −16.8% | 0.82 | 0.81 | 0.82 | |
CSEP | −14.5 ± 6.9% | −7.42 ± 4.7% | 6.5 | <.001 | −10.6% | 0.74 | 0.79 | 0.69 | |
Basal | −12.4 ± 6.2% | −7.1 ± 4.4% | 4.2 | <.001 | −10.0% | 0.72 | 0.77 | 0.66 | |
Midventricular | −13.5 ± 6.1% | −7.5 ± 4.3% | 5.5 | <.001 | −12.1% | 0.72 | 0.88 | 0.56 | |
Apical | −18.3 ± 7.1% | −7.7 ± 5.6% | 11.0 | <.001 | −10.7% | 0.82 | 0.77 | 0.87 | |
CSEN | −27.2 ± 11.3% | −14.1 ± 9.6% | 12.7 | <.001 | −16.2% | 0.74 | 0.65 | 0.83 | |
Basal | −23.2 ± 9.4% | −13.2 ± 9.1% | 9.2 | <.001 | −15.9% | 0.73 | 0.70 | 0.76 | |
Midventricular | −25.6 ± 9.8% | −14.4 ± 8.67% | 10.6 | <.001 | −16.0% | 0.73 | 0.63 | 0.83 | |
Apical | −34.1 ± 12.3% | −14.7 ± 11.6% | 19.9 | <.001 | −25.9% | 0.81 | 0.84 | 0.77 | |
LS full wall thickness | −17.1 ± 6.2% | −10.8 ± 5.9% | 7.0 | <.001 | −14.4% | 0.72 | 0.76 | 0.70 | |
Basal | −13.7 ± 4.8% | −8.7 ± 5.7% | 6.2 | <.001 | −13.2% | 0.71 | 0.84 | 0.57 | |
Midventricular | −15.9 ± 4.9% | −10.8 ± 5.5% | 5.1 | <.001 | −14.5% | 0.73 | 0.79 | 0.67 | |
Apical | −21.0 ± 6.9% | −10.47 ± 6.5% | 10.8 | <.001 | −15.8% | 0.81 | 0.83 | 0.79 | |
LSEP | −14.6 ± 5.3% | −9.2 ± 5.2% | 5.8 | <.001 | −12.8% | 0.73 | 0.79 | 0.67 | |
Basal | −13.7 ± 4.8% | −10.1 ± 5.1% | 4.1 | <.001 | −12.8% | 0.68 | 0.75 | 0.61 | |
Midventricular | −14.3 ± 4.6% | −9.6 ± 5.0% | 4.7 | <.001 | −12.35% | 0.71 | 0.72 | 0.71 | |
Apical | −15.7 ± 6.1% | −7.9 ± 5.2% | 8.0 | <.001 | −10.8% | 0.79 | 0.75 | 0.83 | |
LSEN | −20.1 ± 8.9% | −12.8 ± 7.4% | 8.2 | <.001 | −14.81% | 0.67 | 0.62 | 0.71 | |
Basal | −15.2 ± 4.9% | −12.2 ± 6.2% | 4.4 | <.001 | −13.3% | 0.62 | 0.59 | 0.65 | |
Midventricular | −17.5 ± 5.5% | −11.0 ± 6.2% | 5.6 | <.001 | −14.8% | 0.70 | 0.68 | 0.73 | |
Apical | −27.8 ± 9.9% | −14.2 ± 8.9% | 13.9 | <.001 | −21.01% | 0.79 | 0.81 | 0.78 |
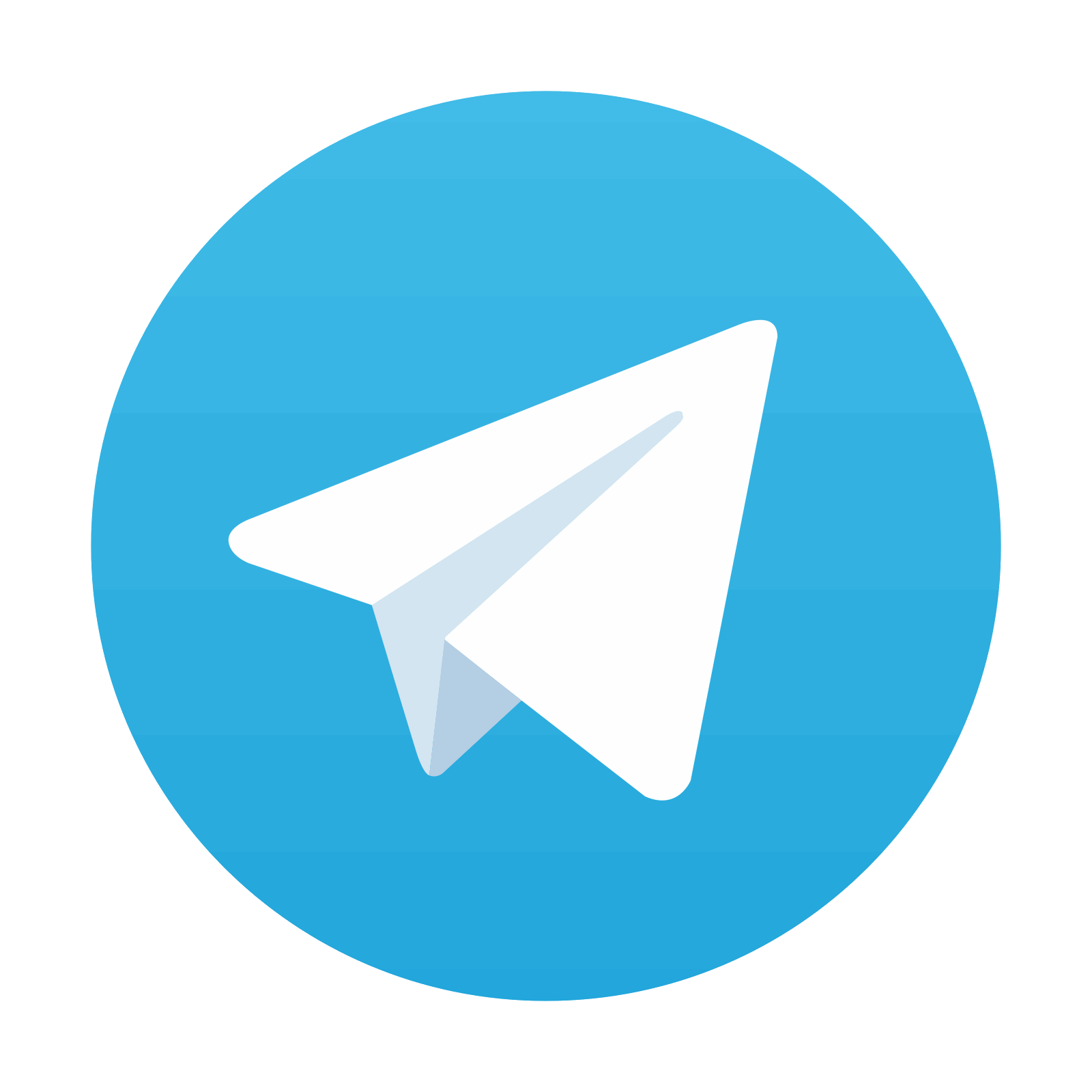
Stay updated, free articles. Join our Telegram channel

Full access? Get Clinical Tree
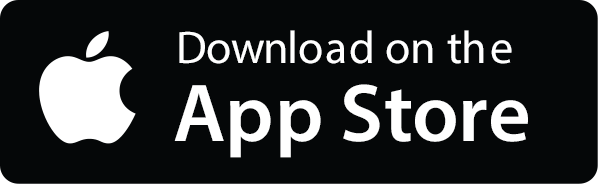
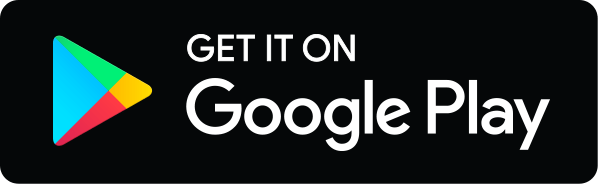
