Over the past decade, cardiac resynchronization therapy (CRT) has changed the treatment of patients with end-stage heart failure. Currently, on the basis of the guidelines of the American Heart Association, American College of Cardiology, and Heart Rhythm Society, CRT is considered a class I indication in patients with drug-refractory heart failure (New York Heart Association functional class III or ambulatory class IV) with left ventricular (LV) ejection fraction ≤ 35%, broad QRS complex (≥120 ms), and sinus rhythm. However, when patients are selected according to the aforementioned criteria, approximately 30% do not have beneficial responses on the basis of clinical outcomes or echocardiographic indicators of reverse remodeling, indicating that currently used guidelines are not perfect at identifying patients with heart failure most likely to benefit from CRT. This is a major issue because CRT is a costly procedure and may potentially expose patients to periprocedural or device-related complications ; therefore, it should be reserved for patients with heart failure for whom positive responses can be anticipated, to optimize the allocation of organizational and financial resources and also to avoid unjustified risk exposure. Hence, a question arises: can cardiac imaging techniques, specifically echocardiography, be applied to better identify CRT responders among the cohort of candidates for biventricular pacemaker implantation on the basis of conventional electrocardiographic and clinical criteria?
The Issue of Mechanical Dyssynchrony
In the past few years, LV mechanical dyssynchrony (ie, the disparity in regional contracting timing) has been considered a possible solution to overcome the limitations of electrical dyssynchrony evaluated by QRS duration. On a pathophysiologic basis, mechanical dyssynchrony appears to be a more correct approach to the problem of dyssynchrony evaluation, because it ultimately determines LV mechanical dysfunction and remodeling. Different echocardiographic methods have been used in numerous small, single-center observational studies to assess mechanical dyssynchrony, and repeatedly favorable results have suggested it as an important determinant of response to CRT. However, the well-known Predictors of Response to CRT (PROSPECT) study, published in 2008, has shown that several echocardiographic parameters (particularly those based on M-mode, conventional Doppler, and Doppler tissue imaging techniques) have only modest accuracy to predict response to CRT, in contrast to the results of the multitude of previously published studies coming from many international centers.
Unfortunately, the PROSPECT trial has been considered by part of the scientific cardiology community, particularly by electrophysiologists, as a definitive study, mainly because of its multicenter design. Many clinicians and investigators have concluded that echocardiographic markers of dyssynchrony basically have no clinical value and that they have little future as predictors of favorable response to CRT. This conclusion, however, is not supported by the PROSPECT study, which had several methodologic and procedural problems and did not test the most pathophysiologically sound and clinically valuable echocardiographic parameters of dyssynchrony, which are based on myocardial deformation (strain) analysis performed by speckle-tracking echocardiography ( Table 1 ). These parameters evaluate directly dyssynchrony of myocardial contraction (not motion or displacement), have the advantage of not being affected by the numerous pitfalls of Doppler tissue imaging, and in several ultrasound studies were able to predict CRT response. In addition, magnetic resonance imaging (MRI) has shown the value of LV strain dyssynchrony in discriminating responders from nonresponders to CRT.
Study | Patients | Endpoint | Follow-up | Parameter | Cutoff value | Sensitivity (%) | Specificity (%) |
---|---|---|---|---|---|---|---|
Suffoletto et al | 50 | ΔEF ≥ 15% | 8 ± 5 months | Radial earliest-latest delay ∗ | 130 ms | 89 | 83 |
Delgado et al | 161 | ΔESV ≥ 15% | 6 months | Radial AS-P delay † | 130 ms | 83 | 80 |
SDt 6s ‡ | 76 ms | 77 | 60 | ||||
Lim et al | 65 | ΔESV ≥ 15% | 3 months | SD-ϵ § | 95 ms | — | — |
SDI ‖ | 25% | 95 | 83 | ||||
Sade et al | 33 | ΔESV>10% | 2-5 days | LV torsion at AVC | 0.1°/cm | 90 | 77 |
LV twist at AVC | 1° | ||||||
D’Andrea et al | 45 | ΔESV ≥ 15% | 6 months | GLS | −12% | 84.7 | 88.8 |
Carasso et al | 76 | ΔEF>10% and/or ΔESV ≥15% | 6 ±4 months | Holosystolic stretching | +5% | 98 | 88 |
∗ Time difference in peak radial strain from earliest to latest of 6 segments in the midventricular short-axis view.
† Time difference in peak systolic radial strain of the AS and P segments in the midventricular short-axis view.
‡ Standard deviation of time to peak systolic strain for all 6 segments of the midventricular short-axis view.
§ Standard deviation of time to peak longitudinal strain of 12 (basal and mid) segments of the left ventricle. For this parameter, sensitivity and specificity are not reported, but the area under the receiving operating characteristic curve was 0.70 ( P = .007) for diagnosis of response to CRT.
On the basis of the evidence coming from myocardial deformation imaging studies, therefore, LV mechanical dyssynchrony can be confirmed as an important factor conditioning the response to CRT, although it still may not be sufficient for patient stratification in addition to the conventional electrocardiographic and clinical criteria. In fact, the accuracy of mechanical dyssynchrony parameters is not perfect ( Table 1 ), and a number of CRT nonresponders remain. How can this be explained? Several factors should probably be considered.
First, mechanical dyssynchrony is not necessarily due to electrical dyssynchrony but may develop as a consequence of the so-called contractile disparity. In other words, LV walls with different contractile properties may generate different contracting forces, and this may produce a time delay in the peak of segmental contractions. In this situation, which is not uncommon in the left ventricles of patients with heart failure, if electrical dyssynchrony is not present, there is no clear evidence that CRT is effective in reducing mechanical strain dyssynchrony, while other interventions, such as β-blockers, have been reported to do so.
Second, at present there is still uncertainty on what is the best approach to determine strain dyssynchrony (radial, longitudinal, and circumferential) for prediction of CRT benefit. Using speckle-tracking echocardiography, radial strain dyssynchrony has been reported to predict CRT response by different authors, while longitudinal and circumferential strain dyssynchrony seem not to have a significant role in discriminating responders from nonresponders to CRT. Apparently, these results disagree with those of MRI-based and Doppler tissue imaging–based deformation imaging studies, demonstrating the value of circumferential and longitudinal strain dyssynchrony.
Finally, other determinants unrelated to dyssynchrony may condition CRT response ( Table 2 ) and should probably be taken into account, in addition to LV mechanical dyssynchrony, for a more comprehensive preimplantation characterization of patients who are candidates for CRT.
LV mechanical dyssynchrony |
LV myocardial scar tissue |
LV contractile reserve |
LV lead position (relative to the most delayed viable segment) |
Coronary venous anatomy |
Device optimization |
Appropriate follow-up strategy |
The Issue of Global Scar Burden and Contractile Reserve
On the basis of the current literature, scar tissue seems to play a pivotal role in conditioning the response to CRT, especially in patients with ischemic heart failure. This should not be surprising, because scar tissue has also shown a (negative) predictive value for the response to β-blocker therapy in patients with heart failure and to revascularization. The total extent of scar tissue in the left ventricle (the so-called global scar burden) is probably the most important parameter: extensive scar tissue limits response to CRT.
Different imaging techniques have been used for the assessment of scar tissue. Although contrast-enhanced MRI may be preferred because its high spatial resolution permits the precise delineation of scar transmurality, global LV longitudinal myocardial strain evaluated by speckle-tracking echocardiography may provide comparable results in predicting response to CRT ( Table 1 ). Standard echocardiography has also been used successfully to identify scar burden and predict the results of CRT. The relative merit of LV global scar burden and mechanical dyssynchrony has been recently investigated in a specific MRI study, and both factors have been recognized as determinants of CRT response in the same patients.
The results of the above-mentioned studies agree with those of other investigations indicating that the global amount of viable myocardium within the left ventricle assessed by nuclear techniques may condition CRT response, as does the global LV contractile reserve elicited by low-dose dobutamine and evaluated by conventional echocardiography. Considered comprehensively, all of these observations highlight the role of the myocardial substrate, in addition to dyssynchrony evaluation, in determining the LV functional response and reverse remodeling that may result from biventricular pacing.
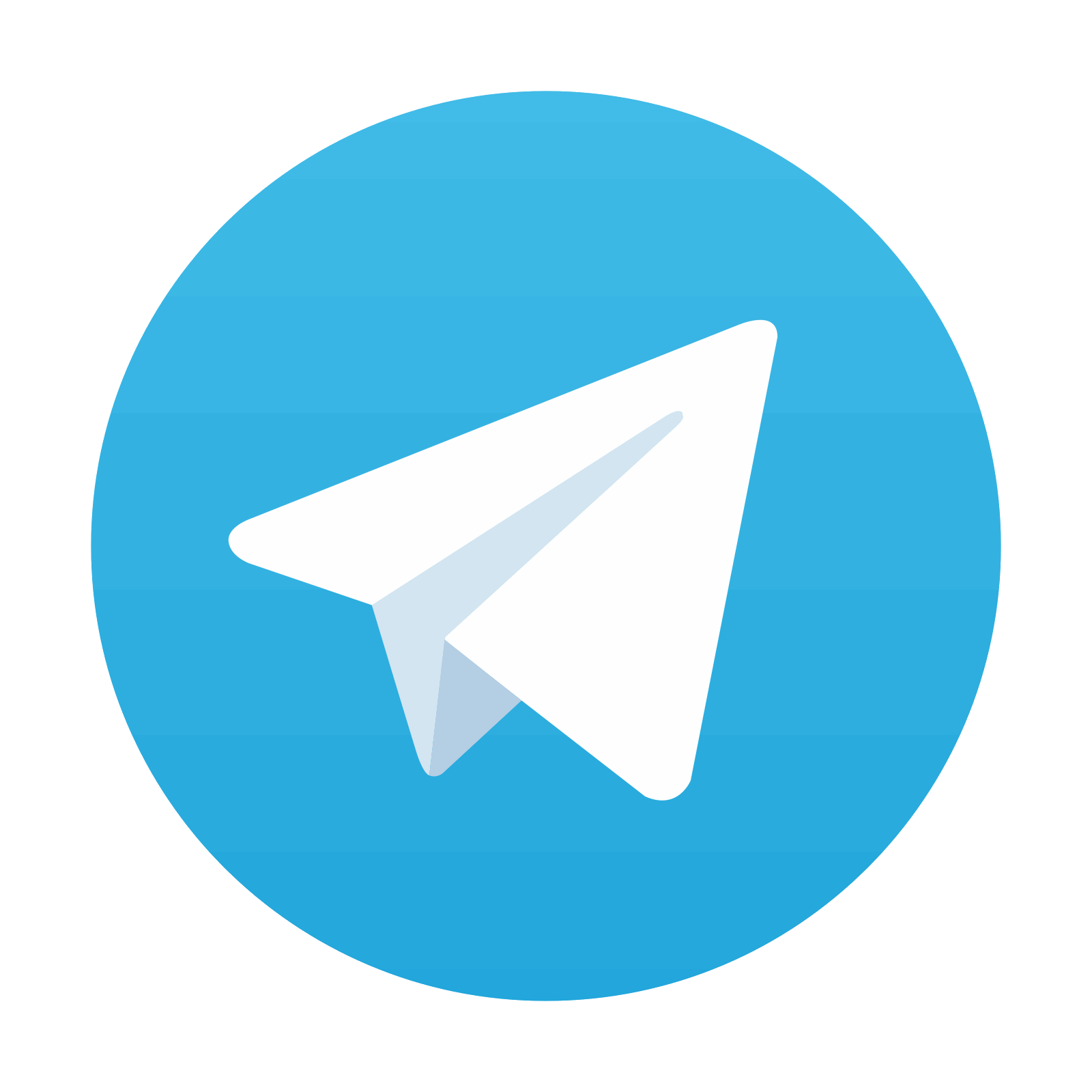
Stay updated, free articles. Join our Telegram channel

Full access? Get Clinical Tree
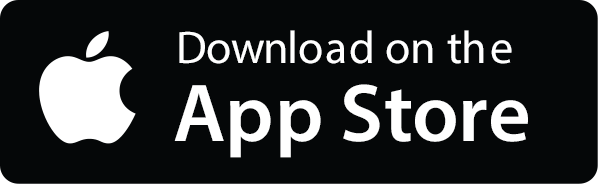
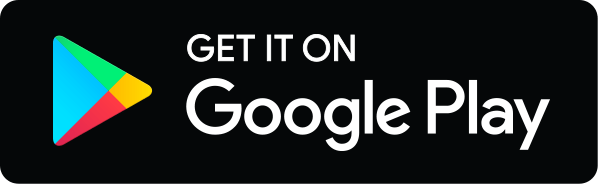