Background
Central venous and arterial catheters are a major source of thromboembolic disease in children. The investigators hypothesized that guided high–mechanical index (MI) impulses from diagnostic three-dimensional (3D) ultrasound during an intravenous microbubble infusion could dissolve these thrombi.
Methods
An in vitro system simulating intracatheter thrombi was created and then treated with guided high-MI impulses from 3D ultrasound, using low-MI microbubble sensitive imaging pulse sequence schemes to detect the microbubbles. Ten aged thrombi >24 hours old were tested using 3D ultrasound coupled with a continuous diluted microbubble infusion (group A) and 10 with 3D ultrasound alone (group B).
Results
The mean thrombus age was 28.6 hours (range, 26.6–30.3 hours). Group A exhibited a 55 ± 19% reduction in venous thrombus size compared with 31 ± 10% in group B ( P = .008). Feasibility testing was performed in four pigs, establishing an in vivo model to investigate further the efficacy of this approach.
Conclusions
Sonothrombolysis of aged intracatheter venous thrombi can be achieved with commercially available microbubbles and guided high-MI ultrasound from a diagnostic 3D transducer.
Central venous catheters (CVCs) and arterial catheters are a major source of thromboembolic disease in children. CVCs are widely used in the care of pediatric patients for intensive care, chemotherapy, transfusion therapy, acquisition of blood samples, and to facilitate supportive care, including nutrition and hydration. The presence of an indwelling CVC is the single most important risk factor for childhood venous thromboembolism (VTE), with approximately 60% of childhood VTE associated with a CVC. VTE is a growing health problem in children and is associated with significant acute mortality and chronic morbidity. There has been a dramatic increase in the diagnosis of VTE at children’s hospitals in the United States. The current estimated incidence of VTE is 4.2/1,000 pediatric hospitalizations. Furthermore, acute VTE is associated with an estimated twofold increased risk for in-hospital death. Chronic consequences are recurrence of VTE and post-thrombotic syndrome. Children may be impaired for longer periods of time with these complications compared with adults.
The optimal therapy for pediatric CVC-associated thrombus or VTE remains poorly defined. There are several management issues unique to children. Removal of the CVC is often fraught with problems because many patients require long-term access, and the insertion of a new CVC may further increase thromboembolic risk. The decision on CVC removal after a thrombotic event must be balanced against factors such as the difficulty of venous access and the need for further treatment and blood sampling. Pediatric CVC-associated thrombus and VTE warrant rapid thrombus resolution in most cases, and the early implementation of thrombolysis is beneficial. However, the use of thrombolytic drugs in children is controversial because of concerns of major hemorrhage. Reported rates of major bleeding with thrombolytic drugs in children are as high as 40%. Because of the absence of large, well-designed clinical trials assessing the use of thrombolytic drugs in children, therapeutic regimens have been extrapolated from adult guidelines. There are important differences in the fibrinolytic system in children that influence the response to thrombolytic agents, and the pathophysiologic mechanisms of thrombosis are quite different from those in adults. Finally, thromboprophylaxis with heparin has been shown in several trials to have no benefit and in general is not recommended in children.
Ultrasound has been investigated as an adjunct to pharmacologic thrombolysis, as well as an independent treatment for vascular thrombosis. Guided high–mechanical index (MI) impulses from a diagnostic ultrasound system during intravenous microbubble infusion have the potential to dissolve intravascular thrombi (termed sonothrombolysis) without the need for fibrinolytic therapy. Cavitation and resultant shear forces are one of the proposed mechanisms for thrombus dissolution by ultrasound. We have previously studied the feasibility of treating deeply located acute intravascular thrombi with ultrasound and intravenous microbubbles. The potential utility of these therapies has not been previously tested using CVCs, in which thrombi are older and more stasis related than arterial thrombi. However, ultrasound appears to dissolve thrombi by a mechanical shearing effect related to cavitation and thus may be more effective than lytic agents in aged thrombi. Our hypothesis, therefore, was that sonothrombolysis might be an alternative method of treating CVC-related thrombi.
Methods
To test our hypothesis, we developed an in vitro system simulating intracatheter thrombi ( Figures 1 and 2 ). Our objective was to test the feasibility and success of sonothrombolysis with guided high-MI impulses from a three-dimensional (3D) ultrasound transducer and using low-MI microbubble sensitive imaging pulse sequence schemes to detect the microbubbles. The study was approved by the Institutional Animal Care and Use Committee and was in compliance with the standards in the Guide for the Care and Use of Laboratory Animals.


Venous blood samples were drawn from healthy pigs to prepare thrombi aged > 24 hours within a Silastic tubing (Dow Corning, Midland, MI) of length 6 cm, internal diameter 2.64 mm, and wall thickness 1.12 mm. The blood samples were not anticoagulated. Each tubing had a layer of Transpore surgical tape (3M Healthcare, St. Paul, MN) secured to the central aspect of its inner wall with the adhesive surface exposed. A plastic connector was attached to one end of the tubing for stability of the unit on a flat surface during weighing as well as during facilitation of thrombus formation. The tubing with the connector was weighed as a unit on a balance with 0.001 g accuracy (Adam Equipment Inc., Milton Keynes, United Kingdom). After withdrawal of venous blood from the pig, 0.1 mL of the blood sample was introduced into the center of the tubing in a contiguous manner. The sample adhered to the surface of the tape as a film < 1.5 cm in length, facilitating red cell deposition on its rough surface and serum formation above. After >24 hours, the tubing with the adherent thrombus within it was weighed and then connected to the in vitro flow system. A 5-cm-thick tissue-mimicking phantom was placed over the tubing to simulate the typical distance between a diagnostic transducer and the superior vena cava in a pediatric patient.
A diagnostic 3D ultrasound system (iE33; Philips Medical Systems, Andover, MA) with an X3-1 matrix-array transducer of 1.6-MHz frequency was used at an angle of 90° with respect to the thrombus ( Figure 3 ). The live 3D volume of coverage had a focused beam, which covered a 30° × 60° field at a depth of 5 cm. During treatment, the transducer was fixed with the aid of a holder so that the center of its 3D beam matrix (90° with respect to the thrombus) permitted the highest peak negative pressure to reach the thrombus underneath. The spatial distribution of peak negative pressures during the high-MI impulses from the 3D transducer was measured through the 5-cm standoff using a calibrated 0.5-mm-diameter needle hydrophone (Precision Acoustics Ltd., Dorchester, United Kingdom). The hydrophone was moved to different locations by a computer-controlled three-axis positioning system with 1.0-mm increments.

For treatment of 10 samples (group A), brief high-MI (>1.0) impulses (10 seconds on, 10 seconds off) were delivered to cavitate microbubbles within the region of interest during the continuous infusion of 0.5% diluted Definity (Perflutren Lipid Microspheres; Lantheus Medical Imaging, North Billerica, MA). The diluted microbubble solution was connected to a saline infusion pump flowing at 1 mL/min. The number of microbubbles passing through the site of the thrombus was calculated to be 6 × 10 7 microbubbles/min. Group B consisted of 10 samples tested with 3D ultrasound alone during 0.9% saline infusion at the same flow rate (1 mL/min). After treatment, each sample with its connector was dried using 30-min air blow and weighed on the same balance as above. In this way, an accurate thrombus weight was obtained before and after sonothrombolytic treatment of each sample.
Establishment of In Vivo Model for Feasibility
To demonstrate feasibility in vivo, we designed and tested the application in a porcine model of chronic indwelling CVC. The design and testing was accomplished in three steps as outlined below.
Step 1: Creation of a Chronic Model of CVC System
After the animal was placed under isoflurane general anesthesia and the surgical site was prepared following aseptic precautions, an incision was made over the jugular furrow of the neck and continued through the subcutaneous tissue and cutaneous coli muscle. The jugular veins were exposed with blunt dissection, and a short incision was made in the skin of the dorsal interscapular region. A trocar was used to tunnel subcutaneously from the ventral incision to the dorsal region of the chest wall. A 10-Fr single-lumen CVC (long-term Carbothane hemodialysis catheter, 35 cm; Bard Inc., Covington, GA) was advanced through the subcutaneous tunnel for placement via surgical cut-down in the superior vena cava. The jugular vein was cannulated using the modified Seldinger technique, and the CVC was positioned with its tip is in the proximal superior vena cava. A check angiogram through the CVC was used to guide accurate position of the tip. The vessel incision was closed with 6-0 Prolene (Ethicon Inc., Somerville, NJ), and the CVC was anchored in the vessel using 4-0 Prolene. The ventral neck incision was closed in layers. The exteriorized portion of the CVC was secured to the skin using nonabsorbable sutures and covered by a protective canvas pouch that was sutured to the dorsum of the animal. The dorsal exteriorizing site was closed with 1-0 Prolene. The animal was recovered from anesthesia with continuous monitoring. The CVC system was flushed with normal saline every 24 hours until step 2.
Step 2: Facilitation of Thrombus Formation in the CVC
The animal returned to the lab 5 days after CVC placement. Under general anesthesia, femoral arterial and venous sheaths (7 Fr) were percutaneously placed for vascular access and hemodynamic monitoring. Further to checking the CVC system with 0.9% saline flush, 0.5 mL of blood was drawn into the CVC to facilitate thrombus formation within its tip over the next 24 hours.
Step 3: Sonothrombolytic Treatment of CVC
Each animal was randomized to receive one of the two treatments for 40 min: 3D ultrasound with microbubble infusion or 3D ultrasound with 0.9% saline infusion. Two end points were chosen as indices of recanalization: comparison of (1) the rate of increase in the drip rate through the CVC during treatment and (2) histopathologic assessment of the residual thrombus present in the explanted CVC.
Before treatment, the CVC tip was localized using fluoroscopy and subclavicular high-frequency two-dimensional ultrasound. The fluid infusion pump was kept at a fixed height of 90 inches, and the change in the drip rate per minute was continuously monitored during treatment using a digital stopwatch. Following treatment, the animal was euthanized, and histopathologic examination of the CVC was performed by a pathologist (S.J.R.).
Statistical Analysis
For the in vitro study, nonpaired t test was used for comparisons between groups. Statistical analysis was performed using Microsoft Excel (Microsoft Corporation, Redmond, WA) and Minitab version 15 (Minitab Inc., State College, PA). A P value < .05 represented significance.
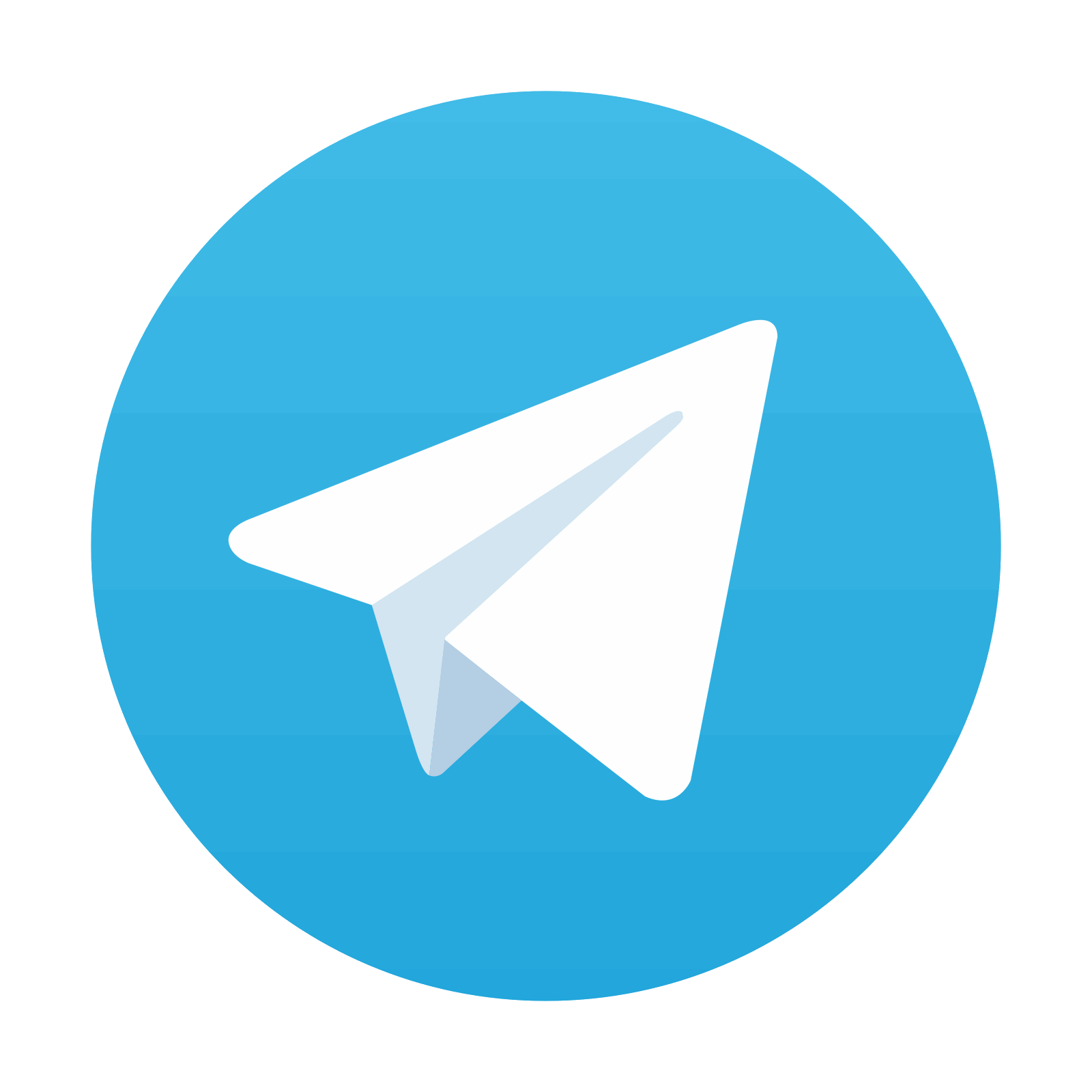
Stay updated, free articles. Join our Telegram channel

Full access? Get Clinical Tree
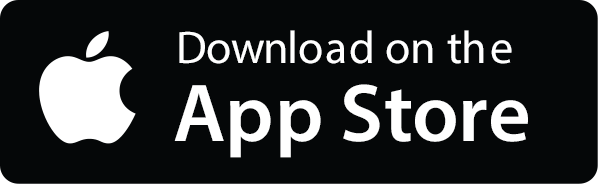
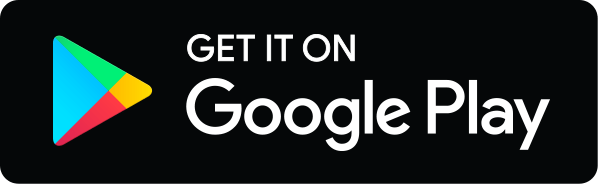