Abstract
Sleep apnea involves almost one billion individuals throughout the world, including 40 million Americans. Of major medical concern is the fact that the prevalence of sleep apnea is significantly increasing due to the epidemic of obesity, physical inactivity, and diabetes mellitus which are important risk factors for the development and persistence of sleep apnea in individuals.
Sleep apnea is characterized by multiple episodes of apnea or hypopnea during sleep, which cause nocturnal arousals, gasping for breath during the night, daytime sleepiness, irritability, forgetfulness, fatigue and recurrent headaches. Obstructive sleep apnea occurs when upper airway obstruction occurs in an individual during sleep with absent or markedly reduced airflow in the presence of continued activity of inspiratory thoracic and diaphragmatic muscles. Central sleep apnea is defined as the absence or the significant reduction of naso-oral airflow due to the withdrawal during sleep of ponto-medullary respiratory center stimulation of the nerves of the inspiratory thoracic and diaphragmatic muscles and absence of contraction of these muscles during apnea. Complex sleep apnea occurs when an individual exhibits characteristics of both obstructive and central sleep apnea.
The severity of sleep apnea is measured by polysomnography and the apnea hypopnea index (AHI), which is the average number of apneas and hypopneas per hour of sleep measured by polysomnography. Sleep apnea is mild if the AHI is 5-14/h with no or mild symptoms, moderate if the AHI is 15 to 30/h with occasional daytime sleepiness, and severe if the AHI is >30/h with frequent daytime sleepiness that interferes with the normal activities of daily life.
Chronic sleep apneas and hypopneas followed by compensatory hyperpneas are associated with significant adverse cardiovascular consequences including: 1) recurrent hypoxemia and hypercarbia; 2) Increased sympathetic nerve activity and decreased parasympathetic nerve activity; 3) oxidative stress and vascular endothelial dysfunction; and 4) cardiac remodeling and cardiovascular disease. Moderate or severe sleep apnea significantly increases the risk of coronary artery disease, congestive heart failure, cerebral vascular events (strokes), and cardiac dysrhythmias, and also increase the morbidity and mortality of these diseases. Nevertheless, sleep apnea is currently underdiagnosed and untreated in many individuals due to the challenges in the prediction and detection of sleep apnea and a lack of well-defined optimal treatment guidelines.
Chronic continuous positive airway pressure for ≥4 h/night for >70% of nights is beneficial in the treatment of patients with sleep apnea. CPAP Improves sleep quality, reduces the AHI, augments cardiac output and increases oxygen delivery to brain and heart, reduces resistant hypertension, decreases cardiac dysrhythmias, and reduces daytime sleepiness.
The present article discusses the diagnosis of obstructive sleep apnea, central sleep apnea, and complex apnea. Thereafter the important pathophysiologic mechanisms in sleep apnea and the relationship of these pathophysiologic mechanics to atherosclerotic vascular disease are reviewed. Guidelines are then provided for the treatment of mild, moderate and severe sleep apnea. In order to reduce the cardiovascular morbidity and mortality caused by sleep apnea and facilitate the diagnosis and the long-term, effective treatment of sleep apnea in patients, the close cooperation is necessary of cardiovascular specialists, pulmonary specialists, and respiratory therapy/rehabilitation specialists.
Introduction
Sleep disordered breathing includes obstructive sleep apnea (OSA), central sleep apnea (CSA) and sleep associated hypoventilation. This discussion is limited to OSA and CSA which involve almost one billion individuals throughout the world, including 40 million Americans. , Within the United States, the National Commission on Sleep Disorders Research estimates that sleep apnea is responsible for 42 million dollars spent for hospital diagnosis and treatment and for 38,000 cardiovascular deaths each year. Of major medical concern is the fact that the prevalence of sleep disordered breathing is significantly increasing due to the epidemic of obesity, inactivity, and diabetes mellitus which are important risk factors for the development and persistence of sleep apnea and sleep associated hypoventilation.
Sleep disordered breathing is characterized by multiple episodes of apneas and hypopnea during sleep, which causes nocturnal arousals, gasping for breath during the night, daytime sleepiness, irritability, forgetfulness, fatigue and recurrent headaches. An apneic episode is the absence or greater than 90% reduction of inspiratory airflow for at least 10 seconds. A hypopnea episode is a slow, shallow, restricted breathing with greater than 30% reduction of airflow that occurs for 10 seconds or longer, repeatedly during sleep. Prolonged or repeated apneas or hypopneas during sleep are associated with a decreased in the arterial oxyhemoglobin saturation (SpO2) of ≥3%.
The severity of sleep disordered breathing (SDB) is measured by the Apnea–Hypopnea Index (AHI), the Respiratory Disturbance Index (RDI), or the Respiratory Event Index (REI). The AHI is the average number of apneas and hypopneas per hour of sleep measured by polysomnography. The RDI is the total number of apneas, hypopneas, and the respiratory effort related arousals per hour of sleep during polysomnography. The REI is the number of apneas and hypopneas per hour of total recording time measured by a monitoring device during presumed sleep (as sleep is not measured) at home or elsewhere. The most common measurement of total OSA and CSA is the AHI. OSA is mild if the AHI is 5-14 events/hour with no or mild symptoms, moderate if the AHI is 15 to 30 events/hour usually with occasional daytime sleepiness, or severe if the AHI is >30 events/hour often associated with frequent daytime sleepiness that interferes with normal activities of daily life.
Apneas and hypopneas followed by compensatory hyperpneas are associated with acute adverse cardiovascular consequences including: 1) Intermittent hypoxemia and fluctuations in the arterial carbon dioxide concentration; 2) Increased sympathetic nerve activity and decreased parasympathetic nerve activity; 3) oxidative stress and vascular endothelial dysfunction; and 4) cardiac remodeling and cardiovascular disease. These adverse cardiovascular consequences of moderate or severe sleep disordered breathing increase the risk of cardiovascular abnormalities and cardiovascular mortality including :
- •
Coronary Artery Disease
- •
Congestive Heart Failure
- •
Cardiac Dysrhythmias
- •
Cerebral Vascular Events (Strokes).
Nevertheless, sleep apnea is currently underdiagnosed and untreated in many individuals due to the challenges in the prediction and detection of sleep apnea, and a lack of well-defined optimal treatment guidelines.
Sleep apnea diagnosis
There are three types of sleep apnea: obstructive sleep apnea, central sleep apnea and complex apnea. Obstructive apnea occurs when upper airway obstruction occurs in an individual during sleep with absent or markedly reduced airflow in the presence of continued activity of inspiratory intercostal and diaphragmatic muscles. In contrast, central sleep apnea occurs when there is a transient absence or reduction of respiratory muscle nerve stimulation by the ponto-medullary respiratory centers in the brain in the generation of breathing rhythm and airflow, usually due to reductions in the partial pressure of CO2 which falls below a level of PCO2 where breathing ceases, i.e. the apneic threshold. Complex sleep apnea occurs when an individual exhibits characteristics of both obstructive and central sleep apnea. The most common sleep apnea is OSA.
Obstructive sleep apnea
The overall prevalence of obstructive sleep apnea (OSA) in the general population is 9 to 38% and varies with the body mass index, sex, age and the AHI definitions used for diagnosis. Obesity is the most strongly predictive risk factor for OSA. In addition, the prevalence of OSA increases approximately 17- fold as people age from young adulthood to approximately 60–65 years. The incidence of sleep apnea in women increases after menopause due to decreases in body estrogen and progesterone concentrations which impact on weight gain and pharyngeal fat deposits with pharyngeal narrowing. In addition, women who are pregnant or who have polycystic ovarian syndrome also have increased incidences of OSA due to their weight gain and hormonal fluctuations. Obstructive sleep apnea results commonly from fat deposition in the neck and pharyngeal area that restricts airflow during sleep and also from posterior displacement of the tongue during sleep with obstruction of the upper airway. , ,
Twenty to 40% of OSA patients, however, are not obese. In these individuals OSA may result from anatomical factors that can obstruct the upper airway such as significant enlargement of the tongue, a long soft palate, a decreased mandibular or maxillary length, pharyngeal neuropathy, rostral fluid shift from the legs to the neck during sleep, or from non-anatomic factors, such as decreased PCO2 chemosensitivity, and decreased arousal threshold during hypoxemia. ,
The OSA syndrome is characterized by recurring events of partial or complete upper airway obstruction during sleep, resulting in decreased alveolar ventilation, intermittent hypoxemia and hypercarbia associated with increased respiratory efforts of the intercostal muscles and diaphragm and intra-thoracic negative pressure swings that frequently lead to patient arousal and fragmented sleep. The International Classification of Sleep Disorders defines OSA as the presence of symptoms or certain comorbidities associated with five or more predominantly obstructive respiratory events per hour or in asymptomatic individuals by 15 or more predominantly obstructive respiratory events per hour.
Symptoms of OSA include loud snoring, frequent nocturnal waking due to gasping or choking, dry mouth, nocturia, morning headaches, poor concentration during the day, daytime sleepiness and fatigue, irritability, and male erectile dysfunction. Symptoms often reported by women include insomnia, impaired memory, and mood disturbances.
The physical examination of the patient with OSA may demonstrate central obesity with increased waist and neck circumference, nasal septal deviation or turbinate hypertrophy, micrognathia, soft palate elongation, macroglossia, and crowding of the posterior oropharynx due to tonsillar enlargement, or dental malocclusion. Signs indicative of pharyngeal obstruction include the presence of snoring indicating a flow-limited upper airway obstruction, a thoracoabdominal paradox with inward motion of the ribcage in concert with outward motion of the abdomen indicative of increased respiratory system resistance, or flattening or scooping of the inspiratory flow loop during pulmonary function testing.
Fig. 1 lists the diagnostic criteria for OSA defined by the American Academy of Sleep Medicine. Fig. 1 is adapted in part from References. ,

An estimated 1 in 5 adults have at least mild OSA and 1 in 15 have at least moderate OSA. Polysomnography is the gold standard for diagnosis of OSA. Polysomnography often includes an electroencephalogram for monitoring sleep staging, arousals, and abnormal seizure activity, an electrooculogram for recording horizontal and vertical eye movements, electromyograms for recording chin muscle tone and periodic leg movements, respiratory channels for recording airflow, respiratory rate/effort and oxygen saturation (SpO2) measurements, and an electrocardiogram for recording heart rhythm. , Home sleep apnea testing can be used in patients with a high pretest probability of OSA but without clinical cardiopulmonary comorbidity. However, 17% of the portable OSA tests are false negatives that are often due to poor sleep and up to 18% have technical measurement failures. Consequently, if the results of the portable testing are negative in an individual for whom there is a high index of suspicion of OSA, additional polysomnography in an accredited facility should be performed. Fig. 2 is a polysomnographic record of an individual with OSA and is adapted, in part, from Reference.

Note in Fig. 2 , the intermittent absence of airflow with obstruction of the upper airway. This is associated with increases in blood pressure and heart rate, and attempts by the thoracic and diaphragmatic muscles to cause airflow. The SpO2 is measured by pulse oximetry usually at the fingertip and the signal is delayed relative to the apnea due to lung-to-finger circulation time. Arousals as noted on the electroencephalogram terminate the obstructive events, with resumption of breathing and restoration of oxygen saturation to normal concentrations.
Central sleep apnea
Central sleep apnea (CSA) is defined as the absence or the significant reduction of naso-oral airflow due to the withdrawal during sleep of ponto-medullary respiratory center stimulation to the nerves of the inspiratory thoracic and diaphragmatic muscles and absence of contraction of these muscles during apnea. The gold standard for the specific diagnosis of CSA is polysomnography with ≥ 5 events of central apnea or hypopneas per hour of sleep with the number of central apneas and/or hypopneas > 50% of the total number of apneas and hypopneas since CSA can occur with obstructive sleep apnea. Central sleep apnea or hypopneas are usually associated with modest decreases in SpO2 with the SpO2 rarely falling below 80 to 85% saturation.
Ventilatory instability is the most widely accepted mechanism leading to CSA in individuals and can be due to increased sensitivity of the ponto-medullary respiratory centers in the brainstem to small changes in CO2. , , Individuals with CSA tend to hyperventilate in response to small increases in PaCO2. Apnea occurs when the PaCO2 decreases below an individual’s apneic threshold, which is 2 to 6 mmHg below the sleeping PaCO2. The ponto-medullary ventilatory stimulation output stops, thereby silencing motor nerves innervating the inspiratory muscles. Ventilation ceases and apnea persists until PaCO2 rises above the apneic threshold. , As the CO2 increases, patient arousal and hyperventilation occur and the cycle of apnea/hypopnea alternating with hyperpnea recurs.
The cessation of ventilatory motor output with CSA initiates a cascade of events that perpetuate unstable breathing including hypoxia, hypercapnia, sympathetic excitation, frequent post-apneic arousals from sleep, surges in ventilatory drive with hyperpnea, and fluctuations in systemic blood pressure. Patients with CSA report frequent awakening with shortness of breath during the night and difficulty sleeping.
Polysomnography and not portable sleep testing is necessary in order to properly differentiate CSA from OSA. Fig. 3 shows the polysomnographic record of an individual with CSA and is adapted in part from Reference. Note in Fig. 3 the lack of thoracic and diaphragmatic muscle movement during the absent airflow. This is associated with an increase in blood pressure and EEG electrical activity with patient arousal.

The following forms of CSA can occur: , ,
- •
Primary (idiopathic) central sleep apnea
- •
Central sleep apnea with Cheyne-Stokes breathing
- •
Central sleep apnea due a medical disorders (Hypothyroidism, Renal Failure) or neuromuscular disorders (Parkinson’s disease, Alzheimer’s disease, Amyotrophic lateral sclerosis)
- •
Central sleep apnea due to opioid or other drug abuse
- •
Central sleep apnea due to high altitude periodic breathing
- •
Treatment-emergent central sleep apnea (TECSA)
Adult CSA can be further divided into 2 main categories based on the PaCO2 levels on awakening. , , The first type is hypocapnic/eucapnic CSA which is characterized by primary (idiopathic) CSA, heart failure-induced CSA, CSA induced by renal failure or other comorbidities, high altitude-induced CSA, and treatment-emergent CSA. The second type is hypercapnic CSA characterized by medication/drug induced CSA and CSA due to neuromuscular disorders. The distinction between hypercapnic vs. eucapnic/hypocapnic (nonhypercapnic) CSA is useful in the identification of the underlying disease/context and deciding on the appropriate ventilator treatment.
Patients with a reduced cardiac output, decreased left ventricular systolic function, and/or atrial fibrillation frequently develop CSA with Cheyne-Stokes breathing. In these patients the low cardiac output delays the signal of the increased blood carbon dioxide concentration during apnea/hypopnea to the ponto-medullary respiratory centers in the brain and significantly limits the brain’s ability to maintain a steady rate of breathing. Breathing then becomes rapid to reduce the carbon dioxide concentration in the blood. However the breathing pattern overcorrects the carbon dioxide concentration and causes an abnormally low concentration of carbon dioxide in the blood. In response, breathing then stops or slows, overcorrecting and restarting the cycle of hyperventilation followed by apnea/hypopnea. Fig. 4 shows the polysomonographic record of an individual with Cheyne Stokes respiration.

Fig. 4 shows the crescendo-decrescendo pattern of airflow and respiratory muscle effort during Cheyne Stokes respiration. The length of the Cheyne-Stokes ventilatory cycle is directly proportional to the lung-to-brainstem chemoreceptor circulation time and inversely proportional to the cardiac output. , Cheyne-Stokes breathing in patients with CSA may also be observed during the day and the arterial PaCO2 in these patients during awake hours is usually less than 40 mmHG.
Male gender, older age, sedentary lifestyle, the presence of atrial fibrillation, ischemic and dilated cardiomyopathy predispose to Cheyne-Stokes respiration. Cheyne Stokes breathing may also occur in 6 to as many as 72% of the patients with cerebral vascular events (strokes) and is more common in hemorrhagic strokes than ischemic infarctions. When Cheyne Stokes respiration does occur in patients with heart failure or stroke, the prognosis is poor.
Central sleep apnea also can occur with drug abuse such as opioid abuse which depresses the central ventilatory drive and results in hypoventilation, hypoxemia and hypercarbia with ataxic or erratic breathing. , Benzodiazepines and baclofen also depress the central ventilatory drive, blunt the response to hypoxia and hypercapnia, leading to CSAs, and increase the risk for OSA by increasing upper airway obstruction through reductions in genioglossus and geniohyoid muscle tone. In addition, the P2Y12 receptor antagonist ticagrelor can result in reductions in ventilatory drive in the respiratory centers and CSA.
Central sleep apnea can be associated with obstructive sleep apnea and can occur in a patient with OSA after restoring the upper airway patency with treatments including positive pressure therapy, an oral appliance, hypoglossal nerve stimulation therapy, or tracheostomy. This form of CSA occurs in approximately five percent of patients and is referred to as Treatment-Emergent Central Sleep Apnea (TECSA) or complex sleep apnea. TECSA represents unmasking of the underlying breathing instability in patients with OSA. , Risk factors for TECSA include male sex, older age group, decreased body mass index, higher baseline AHI and arousal index, chronic medical issues such as chronic heart failure and coronary artery disease and opioid use. The identification of these risk factors and the diagnosis of TECSA is important, as patients with TECSA are at high risk of developing CPAP intolerance and discontinuing therapy.
Diagnostic modalities in sleep apnea
Patient questionnaires have been developed to screen for sleep apnea, including the STOP-Bang questionnaire, the Berlin Questionnaire and the Epworth Sleepiness Scale. , the NoSAS score. and the NoApnea score. These screening questionnaires focus on patient symptoms, anthropometric characteristics associated with obstructive sleep apnea, and have been developed and validated in the general population. However, these screening tools have moderate sensitivity and low to moderate specificity for OSA whereas polysomnography has the highest sensitivity and specificity for diagnosis sleep apnea.
Table 1 lists the currently available devices that are available for the evaluation of sleep disordered breathing and the advantages and disadvantages of the devices. Table 1 is adapted in part from References. ,
Diagnostic technique | Description | Advantages | Disadvantages |
---|---|---|---|
POLYSOMNOGRAPHY | Incorporates electroencepholgram, electrooculogram, electrocardiogram, electromyelogram, airflow, thoracic & abdominal effort, and oximetry to evaluate apneas and hypopneas and leg muscle movements to monitor for other abnormal movements during sleep | Highly sensitive and specific for detecting sleep apneas and hypopneas | Requires overnight procedure in an accredited facility for recordings and involves ≥ 1 technician. Average cost $3000.00 dependent on medical insurance |
HOME SLEEP APNEA TEST | Commercially available devices monitor airflow and respiratory muscle effort. | Not labor intensive. Average cost $300 to $600. | Less accurate than polysomnography because of false negative tests. |
WATCH or RING PERIPHERAL ARTERY TONOMETRY | Devices record home snoring, sleep/ wake state, SpO2, heart rate, body position | Not labor intensive. Less expensive than polysomnography: Average cost $250 to $450 | Primarily for OSA diagnosis. Less accurate than polysomnography. Cardiac dysrhythmias limit specificity. Device may be dislodged from finger during sleep. |
PULSE OXIMETRY | Records nocturnal peripheral oxygen saturation | Not labor intensive. Inexpensive: Cost $150 to $300 | Less accurate than polysomnography or home sleep apnea tests. Easily dislodged from the finger during sleep. Cannot distinguish OSA from CSA. |
Pathophysiologic mechanisms in obstructive and central sleep apnea
Apneas and hypopneas are associated with four acute adverse consequences: 1) Intermittent hypoxemia and hypercarbia; 2) Increased systemic norepinephrine and epinephrine concentrations; 3) large intrathoracic pressure swings; and 4) frequent arousals from sleep. These consequences are qualitatively similar for OSA and CSA, but are more pronounced in OSA.
Chronic intermittent hypoxia and repeated night time arousals cause increased norepinephrine and epinephrine release from sympathetic nerve endings and the adrenal gland, decrease parasympathetic nerve activity, activation of the renin-angiotensin-aldosterone system, and endothelin release. This results in surges in systemic blood pressure, systemic hypertension, increased heart rate, and vascular endothelial dysfunction.
Large intrathoracic pressure swings increase the transmural pressures of the atria, ventricles, intrathoracic aorta, and increase LV afterload, decrease stroke volume and significantly increase myocardial oxygen requirements. , In healthy individuals, intrathoracic pressure during inspiration is approximately -8 cm H2O (-5.8 mmHg). In patients with OSA, attempts to breath against an obstructed upper airway generates negative intrathoracic pressures of approximately -30 cm H2O (-22.1 mmHg). These large pressure shifts during OSA increase venous return to the right heart and increase LV afterload, increase cardiac wall stress, and decrease LV compliance which contribute to ventricular remodeling. Approximately 20 to 30% of patients with chronic OSA also have pulmonary hypertension and increased RV afterload. The thin-walled left and right atria are vulnerable to the surrounding negative pressure and stretch easily, which facilitates the development of atrial dysrhythmias.
Intermittent hypoxia promotes significant oxidative stress and systemic inflammation due to activation of nuclear factor kappa B, inflammatory cytokines (interleukin-6, tumor necrosis factor-alpha), chemokines (monocyte chemoattractant protein-1, interleukin-8), cell adhesion molecules (intercellular adhesion molecule-1), and enzymes (cycloxygenase-2) , In this regard, the serum concentrations of C Reactive Protein (CRP) and TNFa correlate with the AHI, the oxygen desaturation index (ODI), and the percent time the arterial oxygen saturation is less than 90% and inversely correlate with the lowest oxygen saturation in individuals with sleep apnea. , In addition, the concentration of the intercellular adhesion molecule-1 positively correlates with the oxygen desaturation index.
Intermittent hypoxia increases the generation of sterol regulatory element binding protein-1 and stearoyl coenzyme A desaturase-1, the peroxidation of lipids, HDL dysfunction, and increases the total cholesterol concentration in the serum which contributes to the development of dyslipidemia, the formation of vascular atherosclerotic plaques and subsequent coronary artery obstructive disease. , Increased oxidative stress also suppresses nitric oxide formation, which results in vascular endothelial cell dysfunction, dysregulation of vascular vasomotor tone, and vascular atherosclerosis. In the microcirculation, the production of the reactive nitrogen species peroxynitrite is significantly increased during hypoxia which also contributes to microvascular dysfunction.
Chronic cycles of hypoxia then deoxygenation also activate the phospholipase C pathway which leads to synthesis of lef-1. This results in decreases in mitochondrial and myocyte function, increases in endothelin-1 and vasoconstriction, and ultimately can contribute to myocardial ischemia and infarction. In addition, recurrent hypoxia activates platelets and increases platelet aggregation which contributes to vascular thrombosis and myocardial ischemia/infarction. In this regard, the degree of platelet activation positively correlates with the severity of oxygen desaturation during sleep. ,
Fig. 5 summarizes the different pathophysiologic mechanisms that contribute to cardiovascular disease in patients with sleep apnea. Fig. 5 is adapted in part from Reference.

Epidemiological data suggest that sleep apnea, especially OSA where the majority of investigations have been performed, can contribute to the development of cardiovascular disease (CVD) and amplify the severity of CVD. Repeated nightly episodes of intermittent hypoxia, sympathetic nervous system activation and abrupt swings in intrathoracic pressure can cause blood pressure surges, sustained hypertension, cardiac ischemia and contribute over time to cardiac structural and electrical remodeling, decreased left ventricular systolic and diastolic function, heart failure, cardiac dysrhythmias, stroke and sudden cardiac death. Obstructive sleep apnea can contribute to CVD not only in older adults but also in adults 20 to 40 years of age.
Sleep apnea and cardiovascular disease: Hypertension, Acute Myocardial Infarction, Congestive Heart Failure, Cerebral Vascular Disease (Stroke), and Cardiac Dysrhythmias
Hypertension
The Sleep Heart Health Study, which included more than 6000 participants, and the Wisconsin Sleep Cohort Study of 1451 participants identified an independent association between OSA and systemic arterial hypertension. , Moreover, a prospective follow-up of the Sleep Heart Health population demonstrated that subjects with an apnea/ hypopnea index of 0 to 4.9, 5 to 14.9, and more than 15 events per hour of sleep had odds ratios of developing systemic hypertension over the next 4-years of 1.42, 2.03, and 2.89 respectively, compared with matched control subjects without sleep apneas. The prevalence of OSA is particularly high in patients with drug-resistant hypertension as demonstrated by a study that found occult OSA in many as 83% of patients who had uncontrolled hypertension despite taking three or more antihypertensive drugs at optimum dosages.
Coronary artery disease
Sleep apnea, whether due to OSA or CSA, can independently increase the risk of coronary events due to intermittent hypoxia and oxidative stress, sympathetic nervous system activation, abrupt alterations in intrathoracic pressure, acute blood pressure surges, and increased platelet aggregation
Individuals with OSA with apnea/hypopnea indices ≥40/hour and associated marked hypoxemia are at significant risk for nocturnal cardiac ischemia, myocardial infarction, and sudden cardiac death in comparison with patients without OSA. Cardiac events are especially pronounced between 12 midnight and 6AM due to severe perturbations of autonomic, hemodynamic, humoral, and vascular regulation during sleep. In these people, the relative risk of myocardial infarction and sudden death from midnight to 6 a.m. is 2.6 times greater than the general population and the relative risk is 1.87 for people with mild-to-moderate OSA with AHI of 5 to 39. This contrasts with people with coronary artery disease (CAD) but without obstructive sleep apnea and the general population where myocardial ischemia and infarction and sudden death occur more commonly from 6AM to 12 noon.
Untreated sleep apnea, especially OSA, significantly increases the risk of developing atherosclerotic coronary artery disease. In an investigation of 308 middle-aged individuals with OSA who were followed for seven years, the presence of OSA at baseline was associated with an almost fivefold increase in risk of development of atherosclerotic CAD that was independent of the traditional cardiovascular risk factors. Similarly, in the Sleep Heart Health Study, men aged 40 to 70 years with OSA with an AHI greater than 30/h, who were followed for a mean of 8.7 years, had a 68% higher risk of developing atherosclerotic CAD, including acute myocardial infarction, recurrent myocardial ischemia requiring a coronary revascularization procedure, and cardiac death. Obstructive sleep apnea also has been implicated in the development of coronary atherosclerotic plaque instability and vascular thrombosis even after controlling for confounding cardiac risk factors such as age, weight, and tobacco use. In summary, patients with OSA are at significant risk of experiencing morbidity and mortality from atherosclerotic CAD.
Heart failure
Sleep apnea is present in as many as 50 to 70% of the patients with heart failure, especially men greater than 60 years of age with atrial fibrillation. In addition, the prevalence of sleep apnea in patients with heart failure with preserved ejection fraction appears to be similar to that in patients with heart failure with reduced ejection fraction. , Central sleep apnea occurs in approximately forty percent of the sleep apnea cases observed in heart failure patients, while OSA occurs in the remaining patients with heart failure. Moreover, CSA and OSA are independent predictors of increased morbidity and mortality in patients with chronic heart failure.
Sleep apnea can cause heart failure and heart failure progression due to repeated exposure of the heart to intermittent hypoxia and myocardial ischemia, sympathetic nervous system stimulation, sustained hypertension, increased preload and afterload, decreased vascular endothelial function, and cardiac dysrhythmias. , In this regard, among patients with sleep apnea, a chronic AHI >11/h is associated with a 2.38 increased relative likelihood of developing heart failure independent of other cardiovascular risk factors. In comparison with heart failure patients who do not have sleep apnea, patients with sleep apnea and heart failure have higher rates of hospital admissions, higher hospital costs, higher morbidity that accounts for greater three and six-month post hospital discharge costs, and greater mortality. In summary, sleep apnea can precipitate or aggravate heart failure in patients.
Stroke
Obstructive sleep apnea increases the risk for stroke by 1.97 times in comparison to individuals without OSA and this risk is independent of other risk factors such as age, sex, race, tobacco use, alcohol consumption, body mass index, and the presence of diabetes mellitus, dyslipidemia, atrial fibrillation, or hypertension. Moreover, patients with severe nocturnal hypoxia with an AHI ≥ 20 have as much as 4.3 times greater odds for stroke than individuals without sleep apnea. Persistent OSA is also an independent risk factor for stroke recurrence, prolonged hospitalization for the treatment of stroke, poor functional and cognitive outcomes, and increased mortality , The cognitive deficits in stroke patients arise from an interplay of hypoxia, hypercapnia, changes in cerebral blood flow, and sleep fragmentation that negatively impact daily functioning, work performance and productivity. Consequently, sleep apnea should always be considered when assessing patients with strokes or recurrent transient ischemic attacks.
Cerebral vascular events (strokes) can also precipitate sleep apnea. Central sleep apnea occurs in 6% to 24% of patients after a stroke and is most common within the first five days after a stroke. However, OSA is the most common type of sleep apnea post stroke with more than 50% of stroke survivors developing this type of sleep apnea during the acute phase of stroke.
After an acute stroke, the symptoms and signs of mild sleep apnea tend to decrease in three to six months but moderate to severe sleep apnea can persist in as many as one-third of patients in the chronic phase of stroke. In a study of 165 patients with stroke, an initial AHI that averaged 21/h was significantly associated with an AHI that averaged 18 at 3 months and was also associated with a modified Rankin disability scale >3 with moderate to severe neurological deficits at 3 months. In these patients obstructive sleep apnea was more frequent (84%) than central sleep apnea (13%). Furthermore, approximately 20% of patients with stroke develop a Cheyne-Stokes breathing pattern, which is also associated with an increased stroke functional severity and decreased survival.
In summary, medical personnel must be watchful for sleep apnea in patients during the acute phase of stroke and must re-evaluated patients for sleep apnea three or more months after stroke in order to assess the persistence and the severity of sleep apnea, the prognosis for neurological recovery, as well as the need for ventilator support.
Cardiac dysrhythmias
During sleep apnea, the electrophysiological mechanisms that can contribute to atrial and ventricular dysrhythmias include. , :
- •
Abnormal automaticity due to hypoxemia and respiratory acidosis.
- •
Reentry mechanisms due to vagal stimulation from respiration against an obstructed airway in OSA.
- •
Triggered automaticity due to sympathetic nerve hyperactivity
- •
Triggered electrical activity via early afterdepolarizations.
- •
Delayed after-depolarizations due to increased circulating catecholamines
In patients with OSA, atrial fibrillation occurs in more than 5% of patients but the actual percentage is dependent upon on the patient population studied and the severity of the OSA. In the Sleep Heart Health Study, moderate to severe OSA was independently associated with a four-fold higher risk of atrial fibrillation compared to those patients without OSA. In contrast in all patients diagnosed with atrial fibrillation, the prevalence of OSA is as high as 32% to 39% , Moreover, in the Outcomes Registry for Better Informed Treatment of Atrial Fibrillation (ORBIT-AF) Trial, which included >10 000 patients with atrial fibrillation, patients with coexisting OSA had significantly worse symptoms from atrial fibrillation and greater rates of hospitalizations for treatment. Even after successful electrical cardioversion of atrial fibrillation, 51% of patients with OSA that are untreated have recurrences of the atrial fibrillation in comparison with 30% of patients without OSA. , The development of atrial fibrillation in patients with CSA is strongly associated with mortality.
In patients with OSA and nocturnal hypoxemia, bradycardia and conduction rhythm disorders also occur due to the activation of the diving reflex by hypoxemia and apnea, with reflex activation of the cardiac vagal nerves. In these patients, the length of the apneas can be as long as 60 seconds. In a report of 23 patients with moderate-to-severe OSA who underwent 16 months of ECG monitoring with an implanted loop ECG recorder, 11 of the patients had frequent heart rates <40 beats/minute and/or prolonged sinus node pauses primarily during sleep. In these patients, the incidence of these dysrhythmias was also related to the body mass index, and the severity of oxygen desaturation during sleep.
In summary, patients with significant bradycardia, sinus pauses, or paroxysmal atrial fibrillation occurring predominantly during sleep should be evaluated for sleep apnea as the etiology of the dysrhythmia. If sleep apnea is diagnosed, the apnea should be aggressively treated. Thereafter, the dysrhythmia should be re-evaluated with a cardiac event monitor.
Serious and potentially fatal ventricular dysrhythmias occur during sleep in patients with OSA due to intrathoracic pressure shifts causing myocardial stretch and remodeling, surges in catecholamines and systemic blood pressure, ventricular hypertrophy and fibrosis, and myocardial oxygen demand/supply mismatch with resultant myocardial ischemia and apoptosis. In addition, parasympathetic nervous system activation during OSA can result in ECG QTc interval prolongation and increased heterogeneity of ventricular repolarization that is manifested as increased QTc dispersion. The incidence of complex ventricular ectopy is most frequent in patients with OSA, obesity, and cardiac disease.
In patients with OSA, ventricular dysrhythmias, particularly premature ventricular contractions, occur more frequently during the apneic phases than during the hyperpneas phase. In contrast, in patients with CSA with the same degree of severity of sleep apnea, ventricular ectopy occurs more frequently during hyperpneas. This is due in patients with CSA to the prolonged circulation time from the lung to the brainstem chemoreceptors as well as sympathetic activation, and increased negative intrathoracic pressure with myocardial stretch and mechanoelectrical dissociation during the hyperpnea. ,
In the Sleep Heart Health Study, bigeminy, trigeminy, quadrigeminy or non-sustained ventricular tachycardia occurred in 25% of the individuals with OSA compared to 14.5% in individuals without sleep-disordered breathing. In a separate study, complex ventricular ectopy occurred in 10.8% of patients with OSA during sleep but only 2.3% of patients without OSA. In addition in patients with SDB/OSA, the odds of non-sustained ventricular tachycardia are three times greater than in patients without SDB even after adjusting for age, sex, body mass index, and prevalent coronary heart disease. Moreover, the recurrence rate of complex ventricular tachycardia (VT) after ventricular catheter ablation is more than seven times greater in patients with OSA and heart disease than in patients with heart disease and VT but without OSA.
In summary, patients with recurrent nocturnal ventricular dysrhythmias or patients with nocturnal dysrhythmias refractory to standard medical therapy or catheter ablation should be investigated for the presence of sleep apnea.
Treatment of sleep apnea: a multidisciplinary approach
General measures
In order to facilitate the long-term, effective treatment of sleep apnea in patients, the close cooperation is necessary of cardiovascular specialists, pulmonary specialists, and respiratory therapy/ rehabilitation specialists. Patient education and engagement using real-time electronic feedback, telemedicine, and peer support are promising strategies for improving adherence to treatments.
Patients with sleep apnea should avoid alcohol and medications such as opioids and benzodiazepines which can depress the respiratory centers and can increase the AHI. Patients should also sleep on their side and avoid the supine position in order to decrease the probability of upper airway obstruction due to posterior displacement of the tongue. The head of the bed should be elevated in order to increase lung function, ventilation-perfusion matching, and oxygen saturation. Patients should pursue a weight reduction diet because a 10% weight loss can be associated with a 26% decrease in the AHI and a 28% reduction in daytime sleepiness in obese patients with OSA.
Patients should also perform regular physical activity, such as brisk walking, which can decrease the AHI by as much as 32%, even without significant weight loss, due to increased upper airway dilator muscle tone, stabilization of chemoreceptor sensitivity, increased slow-wave deep sleep, decreased fluid accumulation in the neck, and a decrease in the systemic inflammatory response. ,
The use of glucagon-like peptide one (GLP-1) agonists or the dual GLP-gastric inhibitory polypeptides/glucose dependent insulin tropic polypeptides (GLP-1/GIP) are beneficial in causing weight loss and decreasing the AHI in patients with OSA. In the Study of Tripeptide in Obesity or Overweight (Surmount) Trial, treatment with the GLP-1/GIP receptor agonist tripeptide reduced the mean body weight in patients by 18% from baseline and decreased the AHI by 29.3 events per hour in patients on CPAP treated with tripeptide and by 5.5 events per hour in patients on CPAP treated with placebo. In addition in patients not on CPAP, Tripeptide reduced the AHI by 25.3 events per hour and by −5.3 events per hour in patients on placebo over 52 weeks.
Chronically obese patients with OSA who are at high risk for major adverse cardiovascular events despite treatment with diet and medications should be evaluated for bariatric surgery for weight loss. In the Metabolic surgery for OSA and Incident Cardiovascular Disease (MOSAIC) Study of patients with obesity and moderate to severe OSA, the cumulative incidence of major adverse cardiovascular events at 10 years, including heart failure and coronary events, was 27.0% in the bariatric surgical group versus 35.6% in the nonsurgical group. All-cause mortality at 10 years was 9.1% in the bariatric surgical group versus 12.5% in the control group. At 10 years, patients treated with bariatric surgery lost approximately 33.2 kg compared with 6.6 kg in the control group. Polysomnographic studies were performed before and after surgery in 13.8% of the patients. Fifty-eight percent of the surgical patients had severe OSA at baseline, but this number decreased to 13% after a median of 2.1 years after surgery. This study illustrates the importance of obesity in the pathophysiology of OSA and the cardiopulmonary benefits of weight reduction surgery
CPAP in patients with OSA
Chronic CPAP therapy is beneficial in the treatment of patients with OSA because CPAP. , :
- •
Improves sleep quality
- •
Reduces the AHI and the severity of OSA
- •
Augments cardiac output and increases oxygen delivery to the heart and brain
- •
Reduces hypertension, especially resistant systemic hypertension
- •
Decreases dysrhythmias
- •
Reduces daytime sleepiness
In patients with OSA and stroke, CPAP decreases stroke severity and increased neurological functioning. In addition, the use of CPAP for greater than 4 h during sleep reverses the increase in nocturnal blood pressure that often occurs in OSA patients. Moreover, CPAP decreases the systolic blood pressure in OSA patients with resistant hypertension by 5 mm Hg and by 3–5 mm Hg in diastolic blood pressure. Significantly, reductions of 2–3 mm Hg in systolic blood pressure are associated with a 4–8% decreases in the future risk of coronary artery disease and stroke.
CPAP can also reduce the incidence of atrial fibrillation in patients with OSA, In a review of 8 studies of patients with OSA, including 698 patients treated with CPAP and 549 patients not treated with CPAP, patients treated with CPAP had a 42% decreased risk of developing atrial fibrillation. In addition, an inverse relationship between CPAP therapy and atrial fibrillation recurrence was observed. Moreover, the risk of recurrent atrial fibrillation following catheter ablation among patients with OSA treated with CPAP is significantly reduced in comparison with OSA patients not treated with CPAP. , Moreover, the effective treatment of OSA with CPAP can prevent sleep apnea induced heart block in as many as 80 to 90% of patients.
CPAP treatment of patients with OSA can also decrease the frequency of ventricular premature complexes in patients with OSA by as much as 58% by decreasing circulating catecholamines and QT interval dispersion and increasing LV stroke volume. ,
While there is good evidence that CPAP treatment in symptomatic patients with OSA decreases blood pressure in hypertensive patients as well as the incidence of atrial fibrillation and the frequency of premature ventricular contractions, CPAP therapy has not been definitely proven to significantly reduce the cardiovascular mortality associated with moderate to severe OSA. In the Sleep Apnea Cardiovascular Endpoints (SAVE) study of 2717 adults with moderate-to-severe OSA and coronary or cerebrovascular disease treated with CPAP plus usual care versus usual care alone, CPAP significantly reduced snoring and daytime sleepiness, but there was no statistically significant effect of CPAP on the composite end-point of death from cardiovascular causes, myocardial infarction, stroke, or hospitalization for unstable angina, heart failure, or transient ischemic attacks over a mean follow-up of 3.7 years. In this study, the patient adherence to CPAP therapy was 3.3 h per night.
In the Randomized Intervention with Continuous Positive Airway Pressure in CAD and OSA (RICCADSA) trial of the effect of positive airway pressure on cardiovascular outcomes in CAD patients with OSA without daytime sleepiness, there was no statistically significant difference between 122 CPAP treated patients and 122 untreated patients in the composite primary endpoint of coronary revascularization, myocardial infarction, stroke, or cardiovascular mortality over a mean follow-up of 57 months.
In the Impact of Sleep Apnea Syndrome in the Evolution of Acute Coronary Syndrome study (ISAACC study), the effect of treatment with CPAP was evaluated on the primary endpoint of cardiovascular events, including cardiovascular death or acute myocardial infarction, non-fatal stroke, hospital admission for heart failure, and new hospitalizations for unstable angina or transient ischemic attack in patients with cardiovascular disease and OSA followed up for 3.35 years. The mean time of adherence to CPAP treatment was 2.78 hours/night. In this study, cardiovascular events occurred in 90 patients in the CPAP treatment group and 102 events in the usual care group. CPAP treatment did not statistically significantly reduce the prevalence of cardiovascular events in this study.
The lack of benefit of CPAP therapy observed in the 3 randomized control trials is best explained by the low compliance of trial participants with CPAP therapy for less than 4 h/night. In this regard, the failure to use CPAP during early morning hours when rapid-eye-movement sleep dominates and hypoxemia is severe, contributed to reduced CPAP efficiency. In the RICCADSA trial, analysis of the outcomes in patients who were adherent to CPAP for more than four hours/night identified a low risk group for the composite outcome of repeat coronary artery revascularization, myocardial infarction, stroke or cardiovascular mortality with six events in the CPAP usage for ≥4 h/night versus 43 events for patients with less than 4 h of CPAP use per night or no CPAP. Similarly in the SAVE study, patients who used CPAP for 4 or more hours/night had a lower risk of cerebral events/strokes (hazard ratio, 0.56)
Patient education and engagement using real-time electronic feedback, telemedicine, and peer support are promising strategies for improving adherence to CPAP treatments. Currently, large, randomized studies of CPAP treatment for ≥ 4 four hours for >70% of nights are required in order to resolve the efficacy of CPAP treatment for patients with moderate to severe OSA and cardiovascular disease. In this regard, the Sleep SMART Trial is assessing whether CPAP treatment for sleep apnea initiated acutely after a stroke or a transient ischemic attack reduces recurrent stroke, acute coronary syndromes, and all-cause mortality 6 months after the acute event, and improves stroke outcomes at 3 months.
Alternative treatments for OSA
Hypoglossal nerve stimulation is available for patients with moderate to severe obstructive sleep apnea who do not tolerate CPAP because of discomfort, air leaks, failure to improve, nasal blockage, and/or claustrophobia. A generator/sensor device with two leads (Inspire UAS System) is surgically implanted under the clavicle. A stimulation lead is attached to a distal branch of the hypoglossal nerve which controls the genioglossus muscle in the tongue. The second lead is attached to the fourth or fifth intercostal muscle in order to detect the respiratory cycle. The device is designed to sense ventilatory effort and provide stimulation to the hypoglossal nerve in synchrony with respiration. Hypoglossal nerve stimulation increases muscle tone to selected upper airway muscles innervated by the hypoglossal nerve and causes the tongue to move forward in the mouth thereby preventing upper airway obstruction during sleep. In the Stimulation Therapy for Apnea Reduction (STAR) study, 98 patients with OSA treated with hypoglossal nerve stimulation were followed for 36 months. The self-reported daily device usage was 81%. Patient polysomnography was performed at 12, 18 and 36 months. In 73 patients the AHI was reduced from the median value of 32.0 ± 11.8 events per hour at baseline to 11.5 ± 13.9 events/hour at 36 months. Soft or no snoring reported by the bed partner increased from 17% at baseline to 80% at 36 months. Ninety-one of the 98 patients were followed for a total observation time of 48 months. In response to hypoglossal nerve stimulation during sleep, daytime sleepiness as measured by Epworth Sleepiness Scale (ESS) was significantly reduced (7.3 ± 4.9 versus baseline 11.4 ± 5.1) and sleep-related quality of life as measured by Functional Outcomes of Sleep Questionnaire (FOSQ) significantly improved (17.5 ± 2.9 versus 14.6 ± 3) when compared with baseline. Soft to no snoring was reported by 85% of the bed partners. Two patients required additional surgery for lead malfunction. Seventy-five patients reported nightly use of the device at 48 months. The authors of this study conclude that hypoglossal nerve stimulation can produce a sustained benefit on patient-reported outcomes (ESS, FOSQ, snoring) at 48 months and is an effective treatment for patients with moderate to severe obstructive sleep apnea.
The optimization of hypoglossal nerve stimulation requires careful patient selection, electrode configuration and placement, and evaluation of tongue activation patterns. Current contraindications for the use of hypoglossal nerve stimulation include patients with central and/or complex apneas >25% of the total apnea-hypopnea index (AHI), anatomical abnormalities that compromise the performance of upper airway stimulation, such as the presence of complete concentric collapse of the soft palate, a BMI>40 kg/m 2 , severe cardiopulmonary disorders, and active psychiatric disease that confound functional sleep-related assessments. ,
Other surgical treatments may include uvulopalatopharyngoplasty which can be considered in selected patients with severe OSA who do not respond to or do not tolerate CPAP treatment. However, uvulopalatopharyngoplasty or laser-assisted uvuloplasty are not uniformly successful in decreasing the AHI or improving patient outcomes, and are not currently routinely recommended. Maxillomandibular advancement can also be an option for highly selected patients with OSA with a decreased mandibular or maxillary length who are intolerant of other therapies. However, this procedure can be associated with bone segment displacement, non-union of the maxilla or mandible, facial nerve injury, complete anesthesia of the lip or chin, severe malocclusion, bleeding or infection.
Oral appliances are recommended as the initial treatment for patients with mild to moderate OSA. The oral devices are either mandibular advancement or tongue-retaining devices. After the oral appliance has been custom fitted, a polysomnographic test or home sleep study should be obtained to evaluate treatment efficacy. In general, oral appliances modestly improve daytime sleepiness and blood pressure but decrease the AHI to a lesser extent than CPAP treatment. , Adverse effects of oral appliances include aggravation of temporomandibular joint dysfunction, jaw pain, teeth shift, gingival irritation, excessive salivation or dry mouth. Consequently, regular dental evaluations are important for all patients with OSA who are prescribed oral appliances.
Additional treatment options for patients with OSA include myofunctional therapy exercises to strengthen oropharyngeal muscles, tonsillectomy and adenoidectomy in young patients when tonsillar enlargement encroaches on the upper airway, and tracheostomy, which is highly effective but rarely utilized currently unless other treatments are ineffective and there is substantial patient comorbidity.
Treatment of central sleep apnea
In patients with CSA, clinicians should focus on the optimization of treatment for comorbid conditions, especially if concurrent OSA is present. If that does not resolve CSA, CPAP can be beneficial in decreasing the AHI, especially in patients with CSA and Cheyne Stokes respiration. In an investigation of 29 patients with CSA with Cheyne-Stokes respirations and heart failure, CPAP produced an 8% increase in LVEF at 3 months and a relative risk reduction of 81% in patient mortality or cardiac transplantation rate over 2.2 years. In the patients in this study, the dedicated use of CPAP was required for approximately 6 h/night for three months in order to be beneficial. However in this study, CPAP had no significant effect on either mortality or cardiac transplantation in patients with CSA without Cheyne Stokes respiration.
The Canadian Continuous Positive Airway Pressure for Patients with Central Sleep Apnea and Heart Failure trial (CANPAP trial) tested the hypothesis that CPAP would improve the survival rate without heart transplantation of patients who have central sleep apnea and heart failure. In this study, CPAP treatment compared with no CPAP treatment significantly decreased the frequency of apnea or hyponea by 21/h vs. –2/h, increased nocturnal oxygenation by 2% vs. 0.4%, increased the LV ejection fraction by 2% vs. 0.4%, decreased norepinephrine concentrations by 1. vs. 0.02 nmol/L, and increased the distance walked in six minutes to 20. vs. –0.8 m. The trial demonstrated that CPAP can attenuate central sleep apnea, improve nocturnal oxygenation and left ventricular systolic function, and lower plasma norepinephrine concentrations, and showed that these effects can be sustained over a mean of two years. However in this study, CPAP did not increase patient survival. In a post-hoc analysis of the CANPAP trial, an increase in the LV ejection fraction and transplant-free survival occurred in 57% patients in whom AHI was reduced to <15/h. No benefits were observed in 43% of patients in whom CPAP did not reduce the AHI to <15/h.
In patients with CSA with Cheyne-Stokes respirations that are poorly responsive or unresponsive to CPAP treatment, bilevel positive airway pressure (BPAP), which cycles between two levels of continuous positive airway pressures, is an alternative treatment option but should not be used with a back-up breathing rate. ,
Experience with adaptive servo ventilation (ASV) in the treatment of patients with CSA and heart failure has been disappointing based on the results of three large trials. The multicenter Adaptive Servo Ventilation for Central Sleep Apnea in Systolic Heart Failure (SERVE-HF) trial involving 1325 participants showed that ASV decreased the AHI but increased the risk of cardiovascular mortality, possibly due to a decrease in the cardiac output from positive airway pressure. Results from the Cardiovascular Improvements with minute ventilation targeted adaptive servo ventilation Therapy in Heart Failure (CAT-HF) Trial involving 125 participants also demonstrated a reduction in the AHI but no improvement in 6-month cardiovascular outcomes in patients receiving ASV plus current optimal medical therapy compared with current optimal medical therapy alone. Similarly in the Effect of ASV on Survival and Hospital Admissions in HF (ADVENT-HF) Trial, ASV and standard of care therapy in 375 patients with SDB over 3.6 years had no significant effect on the composite endpoint of all-cause mortality, first admission to hospital for a cardiovascular problem, new onset atrial fibrillation or flutter, or delivery of an appropriate cardioverter-defibrillator shock and no significant effect on a secondary endpoint of all cause mortality compared with standard of care alone. Nevertheless in the ASV group, the mean AHI decreased to 3–4 events per hour over the course of the trial and was associated with improvements in sleep quality.
CSA can be eliminated by cardiac transplantation, which suggests that severe heart failure may be causative of CSA. However within several months following cardiac transplantation, more than one-third of patients develop OSA due to weight gain, lack of physical exercise, decreased quality of life, and possibly heart rejection.
Alternative treatments
Nasal oxygen is an alternative treatment for patients with central apnea and heart failure. , In patients with CSA with heart failure with reduced ejection fraction, nasal oxygen can improve CSA, LV ejection fraction, exercise capacity, and quality of life, and decrease catecholamines and ventricular dysrhythmias. , However, clinicians must be aware of the fact that hyperoxia can increase the systemic vascular resistance and decrease left ventricular function in patients. Clinical research studies with new oxygen delivery systems which maintain a normal oxygen saturation without hyperoxia hold promise for future alternative treatments for patients with CSA.
For patients with moderate-to-severe CSA, an additional treatment option includes an implantable device and electrode (Zoll remede system), which stimulates the phrenic nerve to contract the diaphragm and restore a normal breathing pattern. The electrode is inserted into either the right brachiocephalic vein or the left pericardiophrenic vein and stimulates the phrenic nerve through the venous wall. . In 151 patients with CSA who were randomized to phrenic nerve stimulation or no stimulation for 6 months, 51% of the patients treated with phrenic nerve stimulation had an AHI reduction of 50% from baseline compared with 11% in the control group. The beneficial effects were associated with improved quality of life and better sleep quality. Side effects of phrenic nerve stimulation such as infection and electrode dislodgment, occurred in 13 of 151 (9%) of patients with the device. ,
Medications
Patients with persistent daytime sleepiness may benefit from medications such as modafinil, to promote wakefulness. Modafinil binds to the dopamine transporter and inhibits dopamine reuptake and removal in the brain. However, modafinil must be used with caution in patients with cardiac disease because of possible hypertension, tachycardia, extrasystoles, and drug interactions with propranolol/metoprolol. Beta-adrenergic antagonists, such as carvedilol, can decrease the nocturnal cardiac sympathetic hyperactivation that occurs in patients with SDB due to repetitive nocturnal arousals and hypoxemia. Angiotensin receptor-neprilysin inhibitors and loop diuretics, such as furosemide or torsemide, can decrease nocturnal water shifts and cause lung and neck decongestion, decrease the plasma volume, and increase the circulation time, which can be beneficial in CSA and OSA. Acetazolamide, which causes mild metabolic acidosis and results in increased respiratory drive, can decrease the frequency of central apneas and the AHI and can increase oxygen saturation in patients with CSA. In this manner, acetazolamide can improve functional capacity and sleep quality. Theophylline, which can inhibit adenosine receptors in medulla and increase ventilatory stimulation, can be used in the treatment of CSA in patients with heart failure. However the central nervous system stimulating actions of theophylline can disrupt sleep time and efficiency and limit the use of this drug in CSA treatment. Other potential adverse effects from theophylline include cardiac arrhythmias and gastrointestinal irritation. Since theophylline has a narrow therapeutic index, close monitoring of theophylline serum concentrations is necessary.
Summary and conclusions
Obstructive and central sleep apnea are common and dangerous cardiac risk factors. Unfortunately, these problems are currently underdiagnosed and untreated in many individuals due to the challenges in the prediction and detection of sleep apnea. Polysomnography has the highest sensitivity and specificity for the diagnosis of sleep apnea however portale sleep testing for OSA can be used in patients with a high pretest probability. Chronic CPAP therapy for > four hours/night, especially during early morning hours when rapid-eye-movement sleep and severe hypoxemia dominates, is beneficial in the treatment of patients with OSA. Hypoglossal nerve stimulation is a successful alternative treatment of in patients with OSA that cannot tolerate positive airway pressure therapy. CPAP Improves sleep quality, reduces the AHI, augments cardiac output and increases oxygen delivery to brain and heart, reduces resistant hypertension, decreases cardiac dysrhythmias, and reduces daytime sleepiness.
In patients with CSA with Cheyne-Stokes respirations that are poorly responsive or unresponsive to CPAP or BPAP treatment, nasal oxygen is an alternative treatment option. An additional therapeutic option for patients with CSA is phrenic nerve stimulation which causes diaphragm contraction and restores a normal breathing pattern. Sleep apnea is a chronic disorder that requires ongoing follow-up and adjustment of therapy over time to optimize patient symptoms. In order to facilitate the diagnosis and long-term, effective treatment of sleep apnea in patients, close cooperation is necessary among cardiovascular specialists, pulmonary specialists, and respiratory therapy/ rehabilitation specialists. Patient education and engagement using real-time electronic feedback, telemedicine, and peer support are promising strategies for improving adherence to treatments.
Large collaborative clinical studies are required for new forms of treatment for sleep apnea with well defined outcome parameters in order to promote more effective treatments and decrease the significant risks of cardiovascular morbidity and mortality among more then the one billion people throughout the world with sleep apnea.
Compliance with ethical standards
The authors have no potential conflicts of interest. This is a Review Article. No human studies or animal studies were carried out by the authors for this article.
Ethical approval
This article does not contain any studies with humans or with animals performed by the authors.
Financial & competing interests disclosure
Work in the cardiovascular research laboratory of RJH is supported by a grant from the Cardiomyopathy Foundation. The author has no other relevant affiliations and no financial involvement with any organization or entity with a financial interest in or financial conflict with the subject matter or materials discussed in the manuscript. No writing assistance was utilized in the production of this manuscript
Declaration of competing interest
The author declare that he has no known competing financial interests or personal relationships that could have appeared to influence the work reported in this paper.
Acknowledgement
The Authors thank Ms. Meg Blount, MLIS, Ms. Mary Katherine Haver, MLIS and all the librarians at the Moffitt Hospital/University of South Florida for their assistance in providing many of the articles cited in this manuscript. The Authors also thank the General Hospital Respiratory and Critical Care Department for their contributions to this article.
Due to space limitations, not every pertinent paper could be included in the References.
References
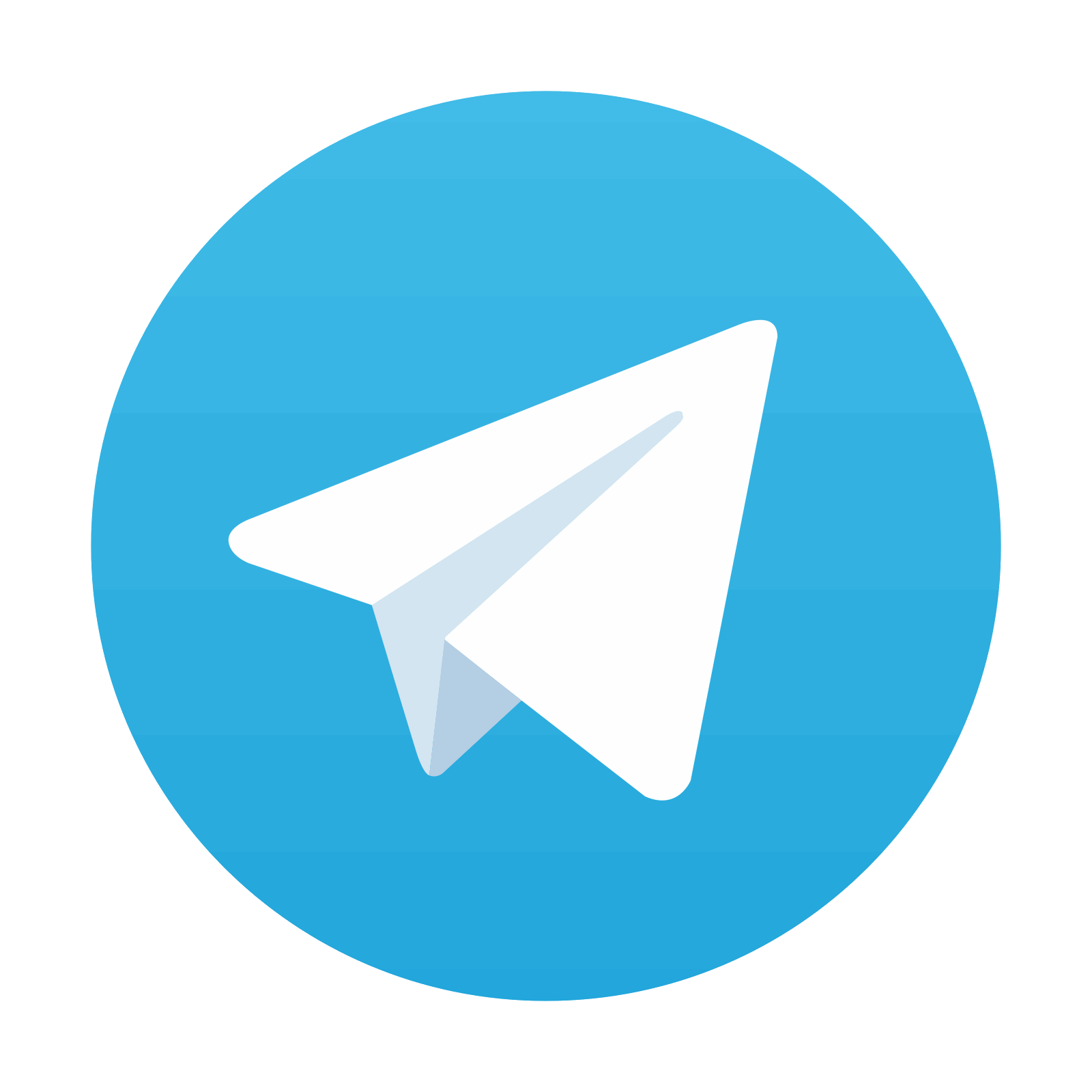
Stay updated, free articles. Join our Telegram channel

Full access? Get Clinical Tree
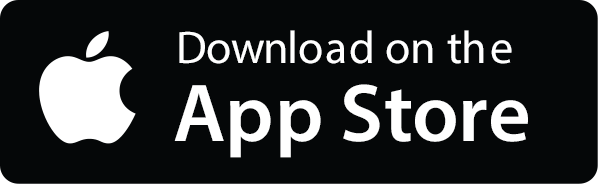
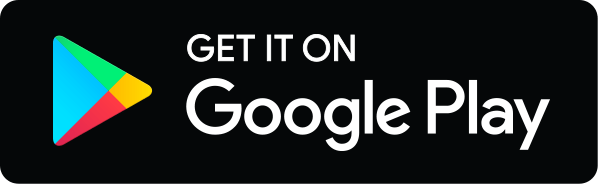
