In general, the most complex forms of congenital heart disease (CHD) fall into the category of single-ventricle defects. Only in recent decades has survival beyond the neonatal period become the norm in the developed world, owing to a number of factors, namely the introduction of prostaglandin infusion, innovative surgical strategies such as the Norwood procedure, accurate postnatal noninvasive assessment through echocardiography, and improvements in prenatal diagnosis. Today, surgical palliation of single-ventricle CHD is expected to result in favorable short- and long-term outcomes into early adulthood for most patients. With the improvement in survival in recent decades, attention is now being turned toward optimizing long-term outcomes and addressing the many functional limitations still experienced by survivors of single-ventricle CHD.
Broadly speaking, single-ventricle CHD can be defined as any congenital heart defect in which there is admixture of systemic and pulmonary venous return in association with atresia (absence of a normally patent structure) or significant hypoplasia (smaller size than normal) of a cardiac valve or chamber.1 Depending on the defect, admixture can occur at 1 or multiple anatomic locations within the heart. Single-ventricle CHD results from atresia or hypoplasia of either an atrioventricular valve or semilunar valve; atresia or hypoplasia of the ventricle associated with the affected atrioventricular or semilunar valve typically exists in conjunction with the valve abnormality. For example, a common form of single-ventricle CHD affecting the right side of the heart is tricuspid atresia, wherein a hypoplastic or imperforate tricuspid valve is associated with underdevelopment of the right ventricle and right ventricular outflow tract. Pulmonary blood flow is provided by antegrade flow from the left ventricle (via a ventricular septal defect) or, in the absence of a ventricular septal defect, via the ductus arteriosus. In either situation, admixture of systemic and pulmonary venous return occurs via obligatory right-to-left shunting at the atrial level. The prototypic form of single ventricle affecting the left side of the heart is hypoplastic left heart syndrome (HLHS).2 In HLHS, atresia or hypoplasia of the mitral valve and/or aortic valves is associated with atresia or hypoplasia of the entire ensemble of left heart structures. Systemic output is provided by the right ventricle and ductus arteriosus, with admixture of systemic and pulmonary venous return occurring via left-to-right shunting at the atrial level. From an anatomic standpoint, it is important to recognize that abnormalities of the systemic and pulmonary veins with respect to their number, size, and site of drainage are frequently associated with single-ventricle CHD and that these additional defects can increase the complexity of surgical approaches and affect outcomes.3,4,
It should be noted that “true” single ventricle (ie, the situation in which there is only 1 ventricle) is quite rare.5 In most cases of single-ventricle CHD, there is a dominant ventricle of either left or right ventricular morphology, and the secondary ventricle is typically hypoplastic or rudimentary. Due to the relative rarity of anatomic single ventricle, many authors prefer the terms “functional single ventricle” or “functionally univentricular heart” when describing the physiology of single-ventricle CHD. For consistency throughout this chapter, however, the term single-ventricle CHD will be used. In addition, it should be recognized that single-ventricle CHD can result from a large number of individual defects. For the purposes of simplicity, only the most commonly encountered lesions causing single-ventricle CHD will be discussed in this chapter.
A discussion of the anatomy of single-ventricle CHD would not be complete without brief mention of the heterotaxy syndrome. Heterotaxy syndrome is a disorder of visceroatrial situs, that is, the position and orientation of the thoracic and abdominal organs. Heterotaxy syndrome has been described as the coexistence of situs solitus (normal position and orientation of the thoracic and abdominal organs) and situs ambiguus (abnormal position and orientation of the thoracic and abdominal organs) within an individual.6 Single-ventricle CHD is common in patients with heterotaxy, typically in the form of unbalanced atrioventricular canal defects or double-outlet right ventricle with pulmonary outflow tract obstruction, although up to 25% of affected patients may be candidates for biventricular repair.7 In addition to the complex underlying cardiac anatomy, abnormalities of cardiac position, systemic venous drainage, and pulmonary venous drainage occur in a sizeable proportion of patients.8 Profound rhythm disturbances such as complete heart block are seen in nearly 20% of patients with heterotaxy8 and confer a poor prognosis.9,10 A number of extracardiac abnormalities are also associated with heterotaxy, including ciliary dyskinesia, abnormal splenic function, and intestinal malrotation, all of which impact morbidity and mortality following treatment of complex single-ventricle CHD.
As discussed earlier in the previous examples, single-ventricle CHD can be conceptually grouped according to the underlying physiology. It is important to understand that the usual categorization of CHD into “cyanotic” and “acyanotic” forms does not allow for a full understanding of single-ventricle physiology. Rather, single-ventricle CHD is best characterized from a physiologic perspective by grouping the anatomic subtypes according to the presence of obstruction to either systemic or pulmonary blood flow. Within this conceptual framework, the presence or absence of cyanosis is a secondary determination that is in turn dependent on the balance of pulmonary and systemic blood flow within an individual patient. In the setting of single-ventricle physiology, the factors determining the balance of pulmonary and systemic blood flow depend on 3 factors: (1) the magnitude of pulmonary or systemic obstruction; (2) patency of the ductus arteriosus; and (3) the relative resistances between the systemic and pulmonary vascular beds. The latter 2 factors may be manipulated through pharmacologic means and therefore serve as therapeutic targets in the preoperative phase for neonates with single-ventricle physiology.
In single-ventricle physiology, the oxygen saturation in the pulmonary artery is equal to the oxygen saturation in the aorta, due to the admixture of systemic and pulmonary venous return. This is in contrast to both normal physiology, in which the pulmonary artery oxygen saturation is less than that of the aorta, and to transposition physiology, in which the pulmonary artery oxygen saturation is higher than that in the aorta. Each form of obstructed blood flow (systemic or pulmonary) in single-ventricle CHD has distinct physiology, anatomic substrates, and clinical presentation. Simultaneous obstruction to both systemic and pulmonary blood flow is quite rare and is typically incompatible with survival apart from orthotopic heart transplantation.
In lesions with obstruction to pulmonary blood flow, atresia or hypoplasia of the tricuspid valve, the right ventricle, the right ventricular outflow tract, and the pulmonary valve (alone or in combination) leads to inadequate pulmonary blood flow. This results in cyanosis in the absence of a patent ductus arteriosus or aortopulmonary collateral vessels, both of which can provide pulmonary blood flow from the aorta. Low cardiac output may occur if there is any element of obstruction to systemic venous return (described in detail later in the Clinical Presentation section). The major forms of such defects are outlined in Table 10-1. Commonly encountered lesions with this physiology include tricuspid atresia, pulmonary atresia with intact ventricular septum, double-inlet left ventricle, pulmonary atresia with right ventricular aorta, and complete common atrioventricular canal defects unbalanced favoring the left ventricle.
|
Similarly, in lesions with obstruction to systemic blood flow, atresia or hypoplasia of the mitral valve, the left ventricle, the left ventricular outflow tract, and the aortic valve (alone or in combination) leads to inadequate systemic blood flow. In the absence of a patent ductus arteriosus, low cardiac output and circulatory collapse will ensue. However, cyanosis may also be a presenting feature if there is any obstruction to pulmonary venous return (described in detail later in the Clinical Presentation section). Obstruction to pulmonary venous return occurs when either the pulmonary veins drain anomalously in an obstructed fashion or, more commonly, when there is restriction to egress of pulmonary venous return at the atrial septum. In either case, infants with single-ventricle CHD, obstructed systemic blood flow, and obstructed pulmonary venous return present with early-onset, profound cyanosis and have significant morbidity and mortality.4,11 The major forms of single-ventricle defects causing obstruction to systemic blood flow are listed in Table 10-2. Because HLHS is the most commonly encountered single-ventricle defect, much of the content in this chapter focuses on HLHS, reflecting the exhaustive efforts undertaken in recent decades to improve survival for this once uniformly fatal lesion.
|
The overall incidence of CHD reported in the literature is quite variable, due to academic variations in the categorization of the various forms of CHD and changing patterns of diagnosis in recent decades.12 It is generally recognized, however, that CHD affects approximately 0.8% of all births when bicuspid aortic valve and patent foramen ovale are excluded from consideration. The incidence of single-ventricle CHD is significantly less, although its exact incidence is once again difficult to pinpoint given the lack of a standardized definition of single-ventricle CHD in the literature and the significant anatomic variability within the subgroup of single-ventricle lesions. In the landmark “Report of the New England Regional Infant Cardiac Program,”13 published in 1980, the incidence of single-ventricle CHD, which for the purposes of this study included tricuspid atresia, HLHS, pulmonary atresia with intact ventricular septum, and undifferentiated single-ventricle lesions, was approximately 0.05% of live births (0.5 cases per 1000 live births). A narrower definition of single-ventricle CHD was used for the Baltimore-Washington Infant Study (1981-1989), wherein HLHS, tricuspid atresia, and pulmonary atresia with intact ventricular septum were excluded. In this study, the prevalence of single ventricle was far lower at 0.006% of live births, or 1.25% of all cases of CHD.14 Regardless of these varying definitions, it is unlikely that the incidence of single-ventricle CHD in live births has changed significantly over time, as asymptomatic single-ventricle CHD evading detection in the newborn period is quite rare. However, the prevalence of single-ventricle CHD continues to increase commensurate with improved survival.15 The most common form of single-ventricle CHD is HLHS, with an incidence of approximately 266 per 1,000,000 live births (approximately 1000 births/year in the United States).16
The genetic causes of single-ventricle CHD are not yet entirely understood. Although approximately 40 genes have been implicated in nonsyndromic CHD (ie, the presence of CHD in the absence of a known identified genetic syndrome), most cases of CHD appear to be sporadic with multiple risk factors.17 For single-ventricle CHD, mutations in HAND1,18 GJA1,19 and NKX2.520 have been reported in patients with HLHS, with an even larger number of individual gene mutations reported in patients with heterotaxy syndrome.17,21 Several genetic syndromes are well known to be associated with single-ventricle forms of CHD, particularly HLHS: The Turner,22 Rubenstein-Taybi,23 Holt-Oram,24 and Jacobsen syndromes25 are all observed with an increased incidence of HLHS compared to the general population. In a recent report of 240 fetuses with HLHS evaluated at our center over a 5-year period, 9.2% had a major extracardiac genetic or chromosomal abnormality with the most commonly encountered syndromes being Turner syndrome, trisomy 18, and trisomy 13.26 This incidence of major chromosomal abnormalities in HLHS is consistent with the incidence of chromosomal abnormalities (12.9%) in the large, albeit older, Baltimore-Washington Infant Study from the 1980s.27 No specific environmental, infectious, or pharmacologic agent exposure has been implicated as a cause of single-ventricle CHD.
Although most occurrences of CHD are felt to be sporadic, an emerging body of evidence is beginning to decipher the heritable nature of some forms of single-ventricle CHD, particularly HLHS. Relationships between complex single-ventricle defects and less severe manifestations of CHD are coming to attention through careful family studies of patients with HLHS. As an example, less severe yet clinically significant left-sided heart abnormalities appear to exist with increased frequency in first-degree relatives of patients with HLHS. In 2004, Lewin et al.28 reported the results of a study where the first-degree relatives of nonsyndromic patients with congenital aortic valve stenosis, coarctation of the aorta, bicuspid aortic valve, or HLHS underwent screening echocardiography for abnormalities of left ventricular outflow. In the 30 patients with HLHS in this study, 8 first-degree relatives had abnormalities of the mitral valve and left ventricular outflow tract. Recently, a study using genome-wide linkage analysis demonstrated that HLHS maps to multiple chromosomal loci, with a clear relationship of these loci to bicuspid aortic valve.29 These studies suggest that there is a certain degree of heritability to left ventricular outflow tract obstruction. It is therefore reasonable to perform echocardiographic screening of first-degree relatives with HLHS in order to evaluate for bicuspid aortic valve and other left ventricular outflow tract abnormalities.
Due to the well-known incidence of familial clustering of CHD, the recurrence risk of CHD for first-degree relatives has been studied extensively.30 Such information is obviously of great interest to parents of children with single-ventricle CHD as they assess their individual risk of CHD recurrence in future pregnancies. A recent study of a large population from Denmark sheds light on this important question. For any pregnancy affected by CHD, the relative risk of recurrence of the same form of CHD in first-degree relatives was approximately 3, with significantly higher relative risks for specific defects such as heterotaxy syndrome, atrioventricular canal defects, and left ventricular outflow tract obstruction.31 In a follow-up study from the same group, the relative risk of recurrence of another form of CHD in first-degree relatives was approximately 2 to 4 times above the background risk of CHD, depending on the specific defect found in the proband.32 Nonetheless, in both studies, the proportion of CHD accounted for by familial recurrence was less than 5%, again suggesting that most cases of CHD are sporadic. In a large fetal series of approximately 6000 pregnancies, recurrence of CHD in fetuses of first-degree relatives with CHD was 2.7%.33 Therefore, the occurrence of single-ventricle CHD in a family should prompt the performance of a fetal echocardiogram in any future pregnancies, with consideration given to early second-trimester fetal echocardiographic imaging.34
The clinical presentation of single-ventricle CHD is dependent on the specific defect and age at presentation. In the following text, common clinical presentations of single-ventricle CHD will be presented according to the following age groups: fetus, neonate, infant, and child. The clinical presentation of single-ventricle CHD is presented in additional detail in Chapter 5 (Evaluation and Therapy: Neonatal Critical Heart Disease). Signs and symptoms associated with single-ventricle CHD are listed in Table 10-3.
|
Routine ultrasonographic screening of pregnant women in the United States during the second trimester (approximately 20 weeks of gestation) allows for identification of most forms of CHD. However, the rate of prenatal detection of CHD remains surprisingly low (<50%), although the rate of prenatal diagnosis appears to be increasing in recent decades.35-37 Interestingly, single-ventricle forms of CHD, despite their relative rarity, may be more easily diagnosed than other forms of CHD (eg, transposition of the great vessels and other conotruncal defects) because single-ventricle CHD usually results in an obviously abnormal standard 4-chamber view of the heart.35,38 Figure 10-1 demonstrates a normal fetal heart on echocardiography, and Figure 10-2 demonstrates an abnormal fetal heart with HLHS. The effect, if any, of prenatal diagnosis of CHD on postnatal mortality from CHD is not yet clear. Although some studies have shown less short-term morbidity in infants prenatally diagnosed with CHD, there does not appear to be a measurable effect on survival.39 Similar findings have been borne out by other studies from the past decade.40-42
Figure 10-2

Fetal echocardiogram at 25 weeks of gestation demonstrating size discrepancy of the left and right ventricles in a fetus with hypoplastic left heart syndrome HLHS. The mitral valve is atretic (imperforate), and the left ventricle is severely hypoplastic. LA, left atrium; LV, left ventricle; MV, mitral valve; RA, right atrium; RV, right ventricle.
In the fetus, single-ventricle CHD is typically well tolerated from a hemodynamic standpoint unless there are coexisting anatomic abnormalities such as significant atrioventricular valve regurgitation, ventricular dysfunction, or dysrhythmias. However, blood flow patterns through the fetus are altered in the presence of single-ventricle CHD, as has been documented by a number of studies evaluating Doppler flow patterns in sites such as the fetal middle cerebral artery.43,44 In the case of HLHS, the most highly saturated blood from the placenta (umbilical venous return passing through the ductus venosus) is directed from the right ventricle to ductus arteriosus and descending aorta, in comparison to the normal fetus, where umbilical venous return is directed across the foramen ovale to the mitral valve, left ventricle, and ascending aorta. This may have implications for neurologic development; several recent studies have shown that fetuses45 and infants with HLHS46 have smaller brain volumes and maturation than gestational age-matched controls. Infants with single-ventricle CHD also have been shown to have a high incidence of frank microcephaly as well as head circumferences disproportionately small when compared to weight.47
Although the effects on overall mortality from CHD are debatable, a prenatal diagnosis of single-ventricle CHD clearly offers significant benefits to the fetus and parents. First, prenatal recognition of the defect allows for the timely delivery of appropriate medical care to the infant upon delivery. Most infants with single-ventricle CHD will require prostaglandin infusion to maintain patency of the ductus arteriosus. It is clearly preferable for an intravenous prostaglandin infusion to be initiated in a controlled setting, in an infant not yet compromised by profound cyanosis or low cardiac output. In addition, the fetus with single-ventricle CHD may occasionally have additional anatomic features that, if unrecognized, quickly lead to hemodynamic instability. Given the relative rarity of these complicating features layered on top of an already uncommon disease, delivery of a fetus with single-ventricle CHD at a facility unfamiliar with or unprepared for a hemodynamically compromised infant may prove fatal. Examples of these additional anatomic features include restrictive or intact atrial septum in HLHS,48 obstructed pulmonary venous return in forms of single-ventricle CHD associated with the heterotaxy syndrome,49 and complete heart block associated with heterotaxy syndrome.9 Ideally, all fetuses identified with single-ventricle CHD will be delivered at a tertiary center familiar with neonatal CHD. Early evaluation and treatment of infants with single-ventricle CHD may prevent or mitigate the potentially adverse consequences of postnatally diagnosed CHD that may manifest upon ductal closure, including profound cyanosis, circulatory collapse, shock, end-organ dysfunction, and even death. Additionally, identification of single-ventricle CHD allows the family time to prepare for the delivery of an infant with complex disease, to learn about the anatomy and anticipated postnatal treatment strategy, and importantly, to discuss options regarding continuation of the pregnancy.
Despite the increasing likelihood of prenatal diagnosis of single-ventricle CHD, a significant proportion are still identified postnatally, even following discharge from the newborn nursery.50 Late neonatal presentation (ie, following discharge from the nursery) of single-ventricle CHD can occur for several reasons. First, ductal patency is frequently prolonged in infants with single-ventricle CHD; thus, the adverse hemodynamics conferred by ductal closure may not be immediately apparent. On physical examination, many infants with single-ventricle CHD have no cardiac murmurs. Although a single second heart sound may indicate hypoplasia or atresia of a semilunar valve, the presence or absence of splitting of the second heart sound can be difficult to appreciate at the higher heart rates present in the newborn. Finally, cyanosis is generally not apparent unless the oxygen saturation is less than 85%, and this threshold will be lower if the baby is anemic. Screening of all newborns with pulse oximetry in the lower extremity prior to discharge from the newborn nursery will detect cyanosis from both left and right heart obstructive lesions; however, this relatively inexpensive modality is not yet universally practiced in the United States.36 It is important to note that the location of pulse oximetry measurement is vital in obtaining clinically relevant information. Measurement of pulse oximetry in the lower extremity is recommended because hypoxemia from lesions causing obstruction to both pulmonary and systemic blood flow will be detected. If pulse oximetry were measured from the upper extremity in a patient with duct-dependent systemic blood flow, relatively well-saturated blood from the ascending aorta may lead to a falsely reassuring clinical picture.
The neonate with duct-dependent systemic blood flow from a left heart obstructive lesion (eg, HLHS) will typically present with signs and symptoms of low cardiac output. Clinically, this often manifests as lethargy, poor feeding with vomiting, and decreased urine output. On examination, the infant may be mottled, with cool extremities, hepatomegaly, and poorly palpable femoral and pedal pulses. Cyanosis may not be a cardinal presenting symptom unless there is obstruction to egress of pulmonary venous return (ie, restrictive or intact atrial septum in HLHS and other left heart obstructive lesions). These signs and symptoms of low cardiac output can easily be missed51 and may be incorrectly attributed to sepsis; therefore, consideration of critical CHD and initiation of prostaglandin infusion must be given to any ill-appearing neonate.
In contrast, the neonate with duct-dependent pulmonary blood flow from a right heart obstructive lesion (eg, pulmonary atresia with intact ventricular septum) will present predominantly with cyanosis, which may be profound upon ductal closure. In the absence of ventricular dysfunction or atrioventricular valve regurgitation, cardiac output will typically be preserved, and acidosis is not frequently encountered.
It is rare for single-ventricle CHD to present outside the neonatal period. However, there have been case reports of older children and adults presenting with HLHS in whom ductal patency persisted.52,53 Late presentation of single-ventricle CHD may occur in instances of double-inlet left ventricle or other complex forms of CHD without systemic outflow tract obstruction in whom the balance of systemic and pulmonary artery blood flow is such that cyanosis is minimized, yet symptoms of pulmonary overcirculation are avoided as well. This group of patients can be difficult to manage surgically because they frequently have developed pulmonary vascular disease, making surgical palliative strategies along the single-ventricle pathway problematic.
The major differential diagnoses of single-ventricle CHD include sepsis (bacterial, viral, or fungal), pulmonary hypertension, cardiomyopathy, lung disease, shock, ingestion of toxic substances, and other forms of CHD. The most useful tool available to all clinicians to effectively screen for single-ventricle CHD among these conditions is the hyperoxia test. The hyperoxia test will distinguish cyanosis caused by lung disease from cyanosis caused by intracardiac mixing. The hyperoxia test is based on the principle that provision of supplemental oxygenation will improve or normalize systemic oxygenation in the setting of hypoxemia from lung disease, but will not improve or normalize in the setting of single-ventricle CHD. Specialized tools such as echocardiography, cardiac catheterization, and cardiac magnetic resonance imaging (MRI) are required to establish the specific diagnosis (see below). The major differential diagnoses of single-ventricle CHD are presented in Table 10-4. Until the diagnosis of single-ventricle CHD is excluded with certainty, it is recommended that prostaglandin infusion be initiated along with stabilization efforts in any infant in whom CHD is suspected.
|
Absent the history of a prenatal diagnosis, a history and physical examination can frequently lend important insights into the cardiac diagnosis after noncardiac disease processes are excluded. Even in the era of prenatal diagnosis, confirmation of the prenatal findings is important because the accuracy of fetal echocardiography is not 100%54 and may vary from center to center depending on the skill and expertise of those making the diagnosis.55 In cases of left heart obstruction, as outlined earlier, the history often elicits symptoms of poor feeding, lethargy, a weak cry, mottling, and cool extremities. Right heart obstructive lesions are notable for cyanosis of a varying degree. The physical examination should involve a complete set of vital signs, including 4-extremity blood pressures and an oxygen saturation measurement in the lower extremity. The cardiac physical examination may demonstrate several significant findings. The precordium is typically hyperdynamic on palpation, due to 1 ventricle pumping both the systemic and pulmonary output. The first heart sound is typically normal, but in cases of single-ventricle CHD in which there is atresia or hypoplasia of a semilunar valve, the second heart sound is typically single. Normal splitting of the second heart sound virtually rules out single-ventricle CHD. As stated previously, murmurs are unusual; holosystolic murmurs may indicate clinically significant atrioventricular valve regurgitation.
The chest radiograph may be beneficial, although no specific shape or contour to the cardiothoracic silhouette is specific for single-ventricle CHD. The lung fields frequently appear oligemic in patients with right heart obstructive lesions, whereas congestion predominates in those with left heart obstructive lesions, particularly in the setting of a closing ductus. The electrocardiogram is rarely if ever diagnostic, but can confirm sinus rhythm and eliminate dysrhythmias that may complicate the clinical picture. In the current era, most if not all forms of single-ventricle CHD can be accurately diagnosed with 2-dimensional echocardiography with color flow and spectral Doppler analysis. Echocardiography has the advantage of being noninvasive and portable and can delineate anatomic information with high fidelity. Additionally, echocardiography can noninvasively assess ventricular function, and Doppler color flow analysis lends important information regarding valve function. In previous decades, most forms of CHD required cardiac catheterization with angiography for confirmation of diagnosis. Cardiac catheterization remains an important tool for obtaining hemodynamic data and performing interventions and is necessary for diagnostic purposes in rare instances in which there are multiple sources of pulmonary blood flow (eg, major aortopulmonary collaterals in the setting of tetralogy of Fallot with pulmonary atresia), uncertainties with respect to the systemic or pulmonary venous anatomy, or unexpected physiology. The role of cardiac MRI in the diagnosis of CHD remains an evolving process. Cardiac MRI has several advantages over echocardiography as a noninvasive diagnostic tool, particularly with respect to obtaining hemodynamic data and imaging of regions not well imaged by echocardiography (eg, distal branch pulmonary arteries). However, it requires specialized, nonportable equipment; in young children, it requires deep sedation and/or general anesthesia. A generalized algorithm for the diagnosis of single-ventricle CHD is presented in Figure 10-3.
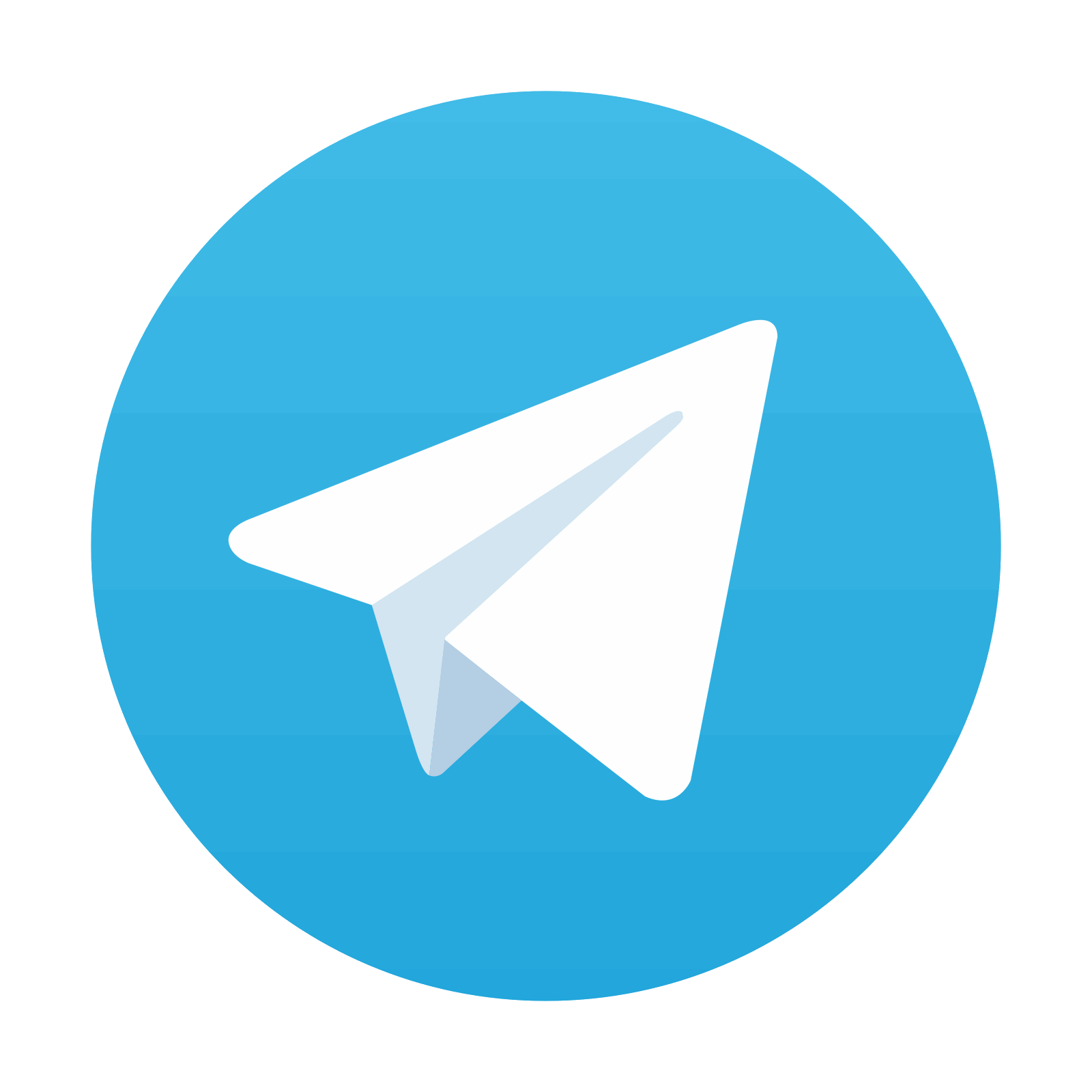
Stay updated, free articles. Join our Telegram channel

Full access? Get Clinical Tree
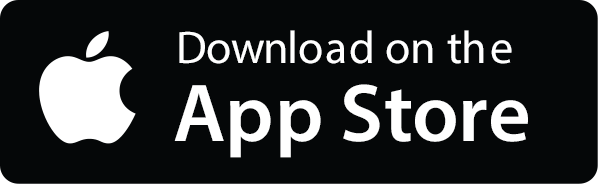
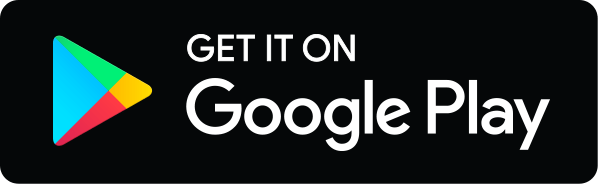