Fig. 11.1
A lo-fidelity mechanical airway model for bronchoscopy teaching
Domenico et al. were able to build a real-scale anatomically accurate bronchoscopy model that cost less than $30 by utilizing iron wires, newspaper sheets, and glazier putty. Goldberg and colleagues built a hybrid model, connecting a plastic tongue and larynx to a porcine trachea and main-stem bronchi as a teaching tool for transbronchial needle aspiration (TBNA) for <$200; the model was viewed, by novice and experienced learners, as realistic and helpful in improving TBNA skills.
In the only study that compared lo- and hi-fidelity simulator as teaching tool in bronchoscopy, the author of this chapter and his colleagues performed a prospective randomized crossover design to train study participants in three methods of conventional TBNA using lo- and hi-fidelity models. Learners felt the models were equally enjoyable and enthusiasm generating but preferred lo-fidelity models in terms of realism and ease of learning. Instructors shared this sentiment with the students and regarded the lo-fidelity model as a more effective teaching instrument for TBNA. This preference for lo-fidelity simulation in this study is not generalizable to all aspects of bronchoscopy learning, but it highlights the effectiveness of lo-fidelity models in procedures that require some tactile feedback such as TBNA (needle insertion through tissue).
Hi-fidelity Simulation
Hi-fidelity simulators are computer-based and rely on the same technology as video games. The bronchoscopy simulator consists of a proxy bronchoscope, a robotic interface device, and a personal computer with a monitor (Fig. 11.2). The proxy bronchoscope is inserted into a plastic face and is maneuvered on the computer screen into a 3-D image recreation of the airways (Fig. 11.3). The robotic interface device tracks the motions of the bronchoscope and reproduces the force felt during an actual bronchoscopy. The “virtual” patient on the screen breathes and coughs, and the vital signs are monitored in real time. Various standardized scenarios are offered, and the learner can choose to examine normal airways, intubate difficult airways, perform brushing or biopsy on an endobronchial tumor, or sample an enlarged lymph node via TBNA (Fig. 11.4). The bronchoscopy simulator also offers anatomic labels on the airway branches and the structures adjacent to the airways to help the learner to become skilled at recognizing anatomy of normal structures; this feature can be turned on or off based on the learner’s level of knowledge and educational needs.
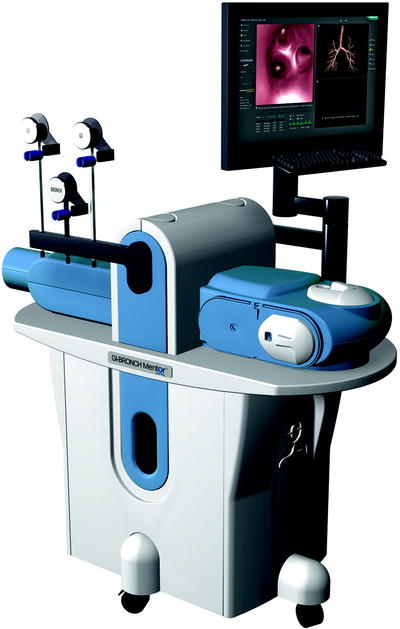
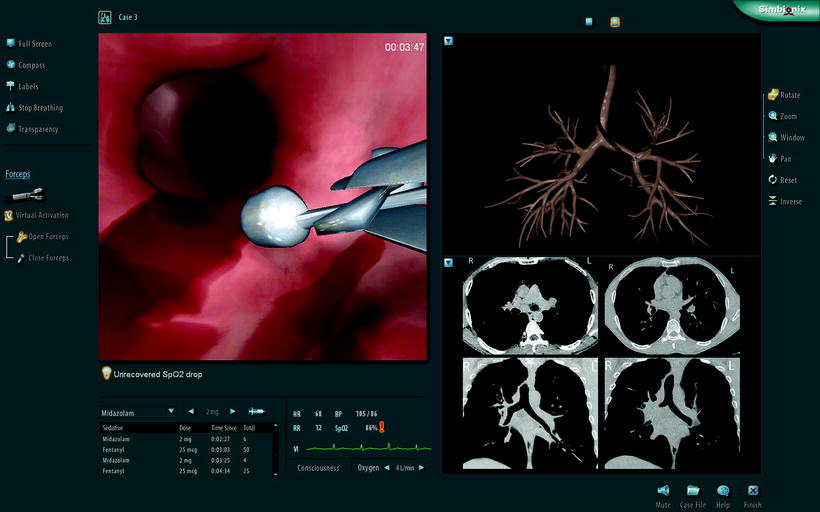
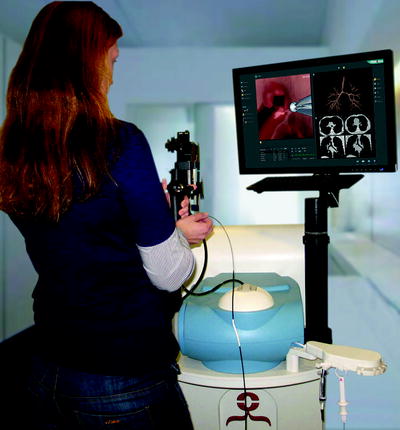
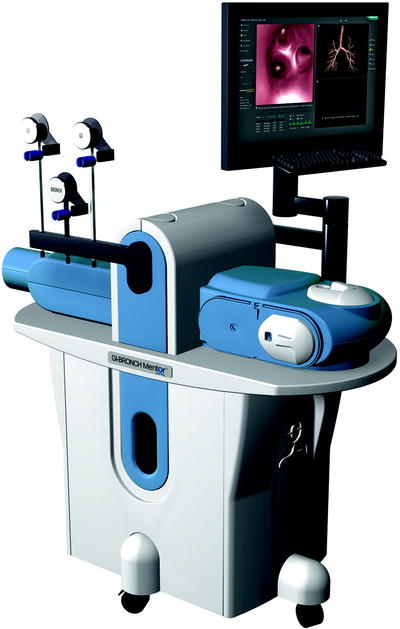
Fig. 11.2
A hi-fidelity computer-based simulator for bronchoscopy teaching (Pictures taken using Simbionix’ BRONCH Mentor simulator)
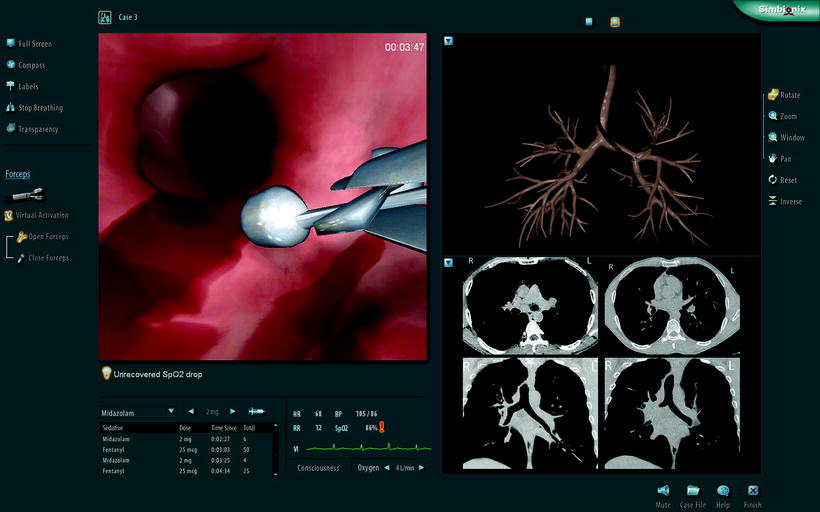
Fig. 11.3
The monitor screen of a hi-fidelity simulator showing a 3-D virtual image of the airways (Pictures taken using Simbionix’ BRONCH Mentor simulator)
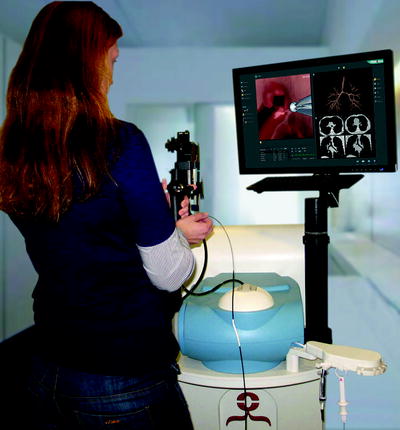
Fig. 11.4
A learner practices her bronchoscopy skills on a hi-fidelity computer-based simulator (Pictures taken using Simbionix’ BRONCH Mentor simulator)
The software tracks performance metrics such as time of procedure, amount of used lidocaine, incidence of wall collision, percentage of segments entered, and success in obtaining a sample from a targeted lymph node.
There are two commercially available hi-fidelity systems in the USA: the Endoscopy VR simulator (CAE Healthcare, Montreal, Quebec, Canada) and the GI-BRONCH Mentor (Simbionix Ltd, Israel).
Hi-fidelity simulation offers numerous advantages including repetitive practice, training in a safe stress-free environment, exposure to rare or difficult scenarios, and receiving immediate feedback on performance.
The data for hi-fidelity simulation in the learning of various medical and surgical procedures are abundant; there have been numerous publications showing the efficacy, cost-effectiveness, and increase in patients’ comfort and safety when simulation-based training is undertaken by trainees.
The first reports of the efficacy of the bronchoscopy hi-fidelity simulator were published in 2001. Colt and colleagues reported the outcomes of five novice bronchoscopists who received 4 h of training on a bronchoscopy simulator and then spent 4 h practicing on their own without supervision; bronchoscopy skill sets obtained by the novices (dexterity, speed, and accuracy) reached those of a control group of skilled bronchoscopists (who had performed at least 200 bronchoscopies) after only 8 h of training. Ost et al. validated the bronchoscopy simulator as an assessment tool and demonstrated its ability to discriminate among bronchoscopists with varying levels of bronchoscopy skills; the study found that expert bronchoscopists (>500 bronchoscopies) performed better than intermediates (25–500) who in turn performed better than novices.
Most recently, the author of this chapter reported the first prospective multicenter study of performance-based metrics and educational interventions in the learning of bronchoscopy among starting pulmonary fellows. In this study, two successive cohorts of starting pulmonary training were enrolled in the study.
At prespecified milestones, validated tools were used to test their bronchoscopy skill and knowledge: the Bronchoscopy Skills and Tasks Assessment Tool (BSTAT), an objective validated evaluation of bronchoscopy skills with scores ranging from 0 to 24, and written multiple-choice question examinations.
The first cohort of fellows received training in bronchoscopy as per the standards set by each institution, while the second cohort received training in simulation bronchoscopy and was provided an online bronchoscopy curriculum. There was significant variation among study participants in bronchoscopy skills at their 50th bronchoscopy, the number previously set to achieve competency in bronchoscopy. An educational intervention of incorporating simulation bronchoscopy enhanced the acquisition of bronchoscopy skills, as shown by the statistically significant improvement in mean BSTAT scores for seven of the eight milestone bronchoscopies (p < 0.05). The online curriculum did not improve the performance on the written tests; however, compliance of the learners with the curriculum was low.
Simulation for Advanced Bronchoscopic Procedures
There has been a rapid expansion in the available technology in pulmonary medicine over the last two decades. Although effective application of most of these technologies requires advanced training and therefore utilization by interventional pulmonologists, some have started to make their ways into general pulmonary practice due to their practical indication and relative ease of use. A prime example is endobronchial ultrasound (EBUS), a technology that allows the real-time guidance of mediastinal lymph node sampling with an average sensitivity and specificity of 93% and 100%, respectively. As the benefit of EBUS-guided TBNA over conventional TBNA in many patients has become clearer, the demand for training, both in fellowship programs and for established clinicians, has continued to increase. Beyond bronchoscopy skills and core knowledge of airway and mediastinal anatomy, working with an EBUS bronchoscope requires the acquisition of specific skills, including driving the scope with reduced optics and an oblique angle of view, acquiring and interpreting the ultrasound images, understanding how to operate the equipment and the needle, and performing the TBNA. A virtual reality simulator with a view mimicking the EBUS bronchoscope would be successful in teaching novice operators to handle the scope effectively and learn the anatomical relationship between airways and surrounding vessels and nodes. Recently, an EBUS capability was added to one of the available bronch simulators (CAE Healthcare, Montreal, Quebec, Canada). Though it has not yet been validated, it holds promise in providing clinicians with a realistic training experience. Alternatively, a lo-fidelity inanimate airway model could serve a similar purpose if simulators are not available. One available airway model (Olympus Inc., Center Valley, Pennsylvania, USA) has a tubular structure with surrounding silicone balls, simulating lymph nodes and allowing a realistic tactile feeling of how the EBUS scope and needle operate. Interpretation of ultrasound images could be learned using computer-based tutorials based on an atlas of CT and ultrasound images. Learning to obtain clear ultrasound images presents additional challenges, as simulators may be unable to mimic real-life challenges of an actual procedure.
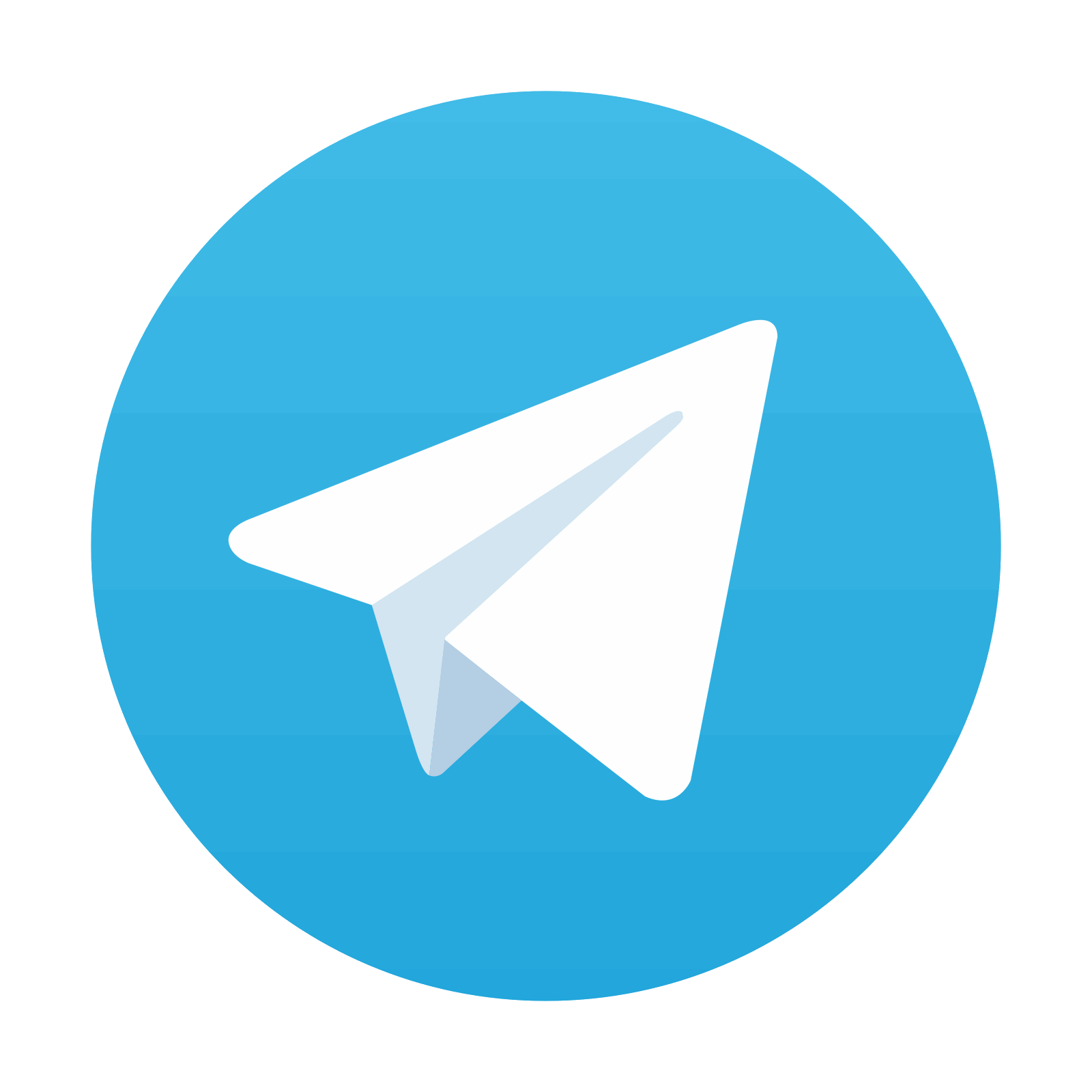
Stay updated, free articles. Join our Telegram channel

Full access? Get Clinical Tree
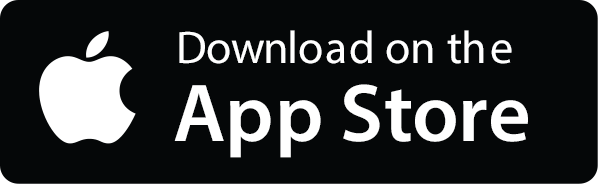
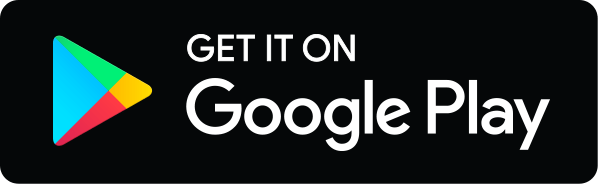