Fig. 7.1
Trends i n death rates overall and for selected sites by sex, United States, 1930–2010. (Reprinted with permission from Siegel et al. [1])
Although the risk of death from lung cancer in current smokers appears to be similar in men and women, several early studies suggested that women may be more susceptible to tobacco carcinogens [6–12]. Further supporting this hypothesis, several studies have found higher levels of tobacco carcinogen induced DNA adducts in lung tumors from women smokers in comparison to male smokers despite lower levels of cigarette exposures [13–15]. Despite this biologic evidence and early case–control studies which suggested an increased susceptibility in female smokers to tobacco carcinogens, several other studies have failed to demonstrate an excess risk for women [16–21]. Therefore, the hypothesis that female smokers are more susceptible to carcinogenic effects of smoking remains controversial.
Sex Differences in Lung Cancer Presentation
Age and Histology
Although the role of sex in lung cancer risk is unclear, a substantial body of literature exists to support the notion that the epidemiology and biology of lung cancer is distinct between the sexes including age, histology, smoking changes, molecular alterations, and the possible role of viral infection (Fig. 7.2). A series of large studies have found that women are diagnosed with lung cancer at an earlier age than men including a large Polish study which included over 20,000 lung cancer patients (age 60.02 versus age 62.18 years; p value <0.001) and a significantly higher percentage of women compared to men were diagnosed under the age of 50 [22]. Although adenocarcinoma (AC) of the lung is the most common non-small cell lung cancer (NSCLC) histology in both men and women, AC is more common in women (41.4 %) than men (34.1 %) [22–24]. Conversely squamous cell carcinoma (SCC) is more common in men [25]. This difference in histology is attributed at least in part to the differences in tar content and filters in the cigarettes smoked by men and women which lead to more central (associated with SCC) or peripheral inhalation of cigarette smoke (associated with AC) [26, 27].
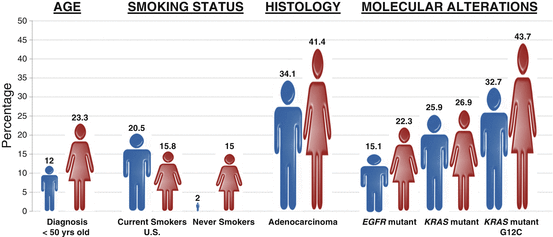
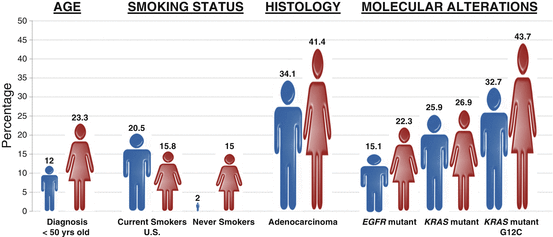
Fig. 7.2
Sex differences in the epidemiology and biology of lung cancer. Compared to men, women with lung cancer are more likely to be younger at diagnosis, never smokers, adenocarcinoma histology, and EGFR mutation positive. See text for details
Never-Smoking Status
Although smoking is the causative factor in the majority of lung cancers, between 16,000 and 24,000 never smokers die of lung cancer each year [28]. Interestingly, the vast majority (70–80 %) of these patients are women [29]. In the United States, approximately 15 % of women compared to 2 % men with lung cancer are never smokers and these numbers rise sharply in Asian populations (reviewed in [30]). A study by Wakelee et al. which examined six large, prospective cohort studies found that the age-adjusted incidence of lung cancer was significantly higher in female versus male never smokers [31]. Remarkably, the death rate from never-smoking lung cancer is 25 % higher in men compared to women suggesting a distinct biology based on sex [32]. In addition to the differences noted above, distinct molecular alterations including targetable and untargetable oncogene drivers are more common in female lung cancer patients .
Molecular Alterations: Oncogenic Drivers and Tumor Suppressors
Over the last decade, much of the progress that has been made in lung cancer therapy has come from the recognition that lung cancer, especially lung cancer in never smokers, is driven by oncogenic drivers which in some cases can be potently targeted. Epidermal Growth Factor Receptor (EGFR) mutant lung cancer was the first oncogene driver-dependent lung cancer to be targeted successfully in the clinic. Mutations in EGFR occur in 21 % of metastatic AC of the lung and predict a 70 % response rate to EGFR tyrosine kinase inhibitors (TKIs) [33]. Shortly after the discovery of EGFR mutations in 2004 [34–36], it quickly became apparent that EGFR mutations were more common in AC of the lung, women (22.3 %) compared to men (15.1 %), and never smokers compared to ever smokers [37, 38]. As these mutations are predictive for response to EGFR TKIs, this may in part explain the increased survival advantage of women with lung cancer. The second most frequent observed targetable oncogene driver in AC of the lung is the anaplastic lymphoma kinase (ALK ). Translocations involving the ALK gene result in a constitutively active fusion protein and are found in 8 % of lung ACs [33]. Similar to EGFR mutant disease, ALK TKIs result in a 70 % response rate in ALK translocation positive lung cancer [39, 40]. ALK translocations are also more common in AC of the lung, never smokers, and younger patients and until recently were thought to be more common in men [41]. However, a recent large meta-analysis of 17 studies and 4511 NSCLC cases has suggested that ALK translocations are more frequent in women than men [42]. A second larger meta-analysis in 1178 ALK rearranged cases from 20,541 NSCLC patients also found that ALK translocations were more frequent in women than men but only in Asian women [43]. Interestingly, the investigators found that in Caucasian populations, men were more likely to have ALK fusions than women. Regardless of ethnicity, there is clearly a significant difference in frequency of ALK translocations between men and women. Finally, the most frequent oncogene driver in AC of the lung, mutant KRAS, also differs according to sex. KRAS mutations are found in 25 % of ACs and portend a poor prognosis in the metastatic setting and KRAS mutant NSCLC is currently untargetable [44]. KRAS mutations were previously reported to be more common in women (26.2 %) compared to men (17.4 %) [45]. However, a more recent and larger study found that KRAS mutations were only slightly more common in women than men overall, but the frequency of distinct KRAS mutations was different in men and women. The smoking associated and most frequently observed KRAS G12C variant was significantly more common in female smokers (43.7 %) versus male smokers (32.7 %) [38]. Interestingly, this higher frequency of the KRAS G12C allele occurred despite females having a lower pack-year smoking history and younger age, suggesting an increased susceptibility to acquire smoking-induced KRAS mutations [38].
In addition to the significant differences in the observed frequency of oncogenic driver mutations, a significant difference in the frequency of mutations in the most common tumor suppressor, p53 , has been observed between men and women. Several studies have demonstrated that smoking-related p53 mutations are more common in women versus men [13, 15, 46]. These findings are speculated to be related to an increased susceptibility of tobacco carcinogen-induced DNA adducts which has been observed in female smokers [13–15].
Role of HPV in Female Lung Cancer
Several epidemiologic studies have suggested a potential role of HPV in lung tumorigenesis in never-smoking women [47, 48] and reviewed in [49]. Most recently, a large meta-analysis of nine studies with a total of 1094 lung cancer cases and 484 noncancer controls found that HPV infection in lung tissue was associated with lung cancer. Similar results were found in with “high-risk” HPV16 and HPV18 positive cases. As previously reported, the association was stronger with SCC than AC of the lung [50]. Two potential mechanisms of HPV infection of the lung have been proposed. The first route of transmission is through the primary infection of the female cervix followed by hematogenous transmission to the lung. Supporting this route of transmission is the fact that women with anogenital malignancies have a higher risk of lung cancer as compared to those without anogenital malignancies [51, 52]. The second proposed route of transmission is through the high-risk sexual activity leading to infection of the oral cavity and subsequent transmission to the lungs. Although there is substantial evidence for the association between oral sex, HPV infection, and head and neck cancer, to date, little data exists to support the transmission of oral HPV infection to the lung resulting in a subsequent lung cancer [49]. The role of HPV in lung cancer and its routes of transmissions remain an unanswered question which requires further investigation .
Sex as a Prognostic Factor in Lung Cancer Outcome
Female sex has been repeatedly demonstrated to be a favorable prognostic factor for lung cancer. Women display superior lung cancer survival rates independent of other factors such as stage, histology, treatment, and smoking status, even after taking into account sex-specific life expectancy [53–55]. Multiple studies have shown better overall survival rates in women with NSCLC following surgical resection [53, 56–63]. This trend has also been observed in female patients with small cell lung cancer (SCLC) [64, 65]. Although the causes of these differences are not well defined, the etiology behind these observations may be linked to genetic or molecular factors discussed in other sections of this chapter. Nakamura et al. recently conducted a meta-analysis of 39 published studies on NSCLC survival differences between men and women which included 32,701 women and 54,099 men and reported that survival of women with NSCLC was significantly better than that for men [66]. This survival advantage was significant regardless of type of statistical analyses used, stage, histology, and smoking status. Biological behaviors, such as differential smoking habits between men and women, have been hypothesized to potentially affect such comparisons and thus a definitive conclusion about the prognostic influence of sex cannot be made. However, the death rate in female never smokers with lung cancer is 25 % lower than male never smokers suggesting a distinct biology based on sex [32].
Because lung cancer prognosis differs between males and females, the male/female ratio has been compared to clinical treatment outcome for lung cancer. Several studies have suggested that sex may be considered not only a prognostic factor but a predictive factor as well. An improved benefit from chemotherapy has been observed for women with SCLC compared to men in multiple studies [64, 67, 68]. However, the data regarding an increased benefit from chemotherapy in females with NSCLC have been conflicting with some studies showing no difference in response rates [69] while others demonstrating a survival benefit for women [70]. In addition, women have been reported to experience greater toxicity from certain chemotherapeutic drugs [67, 68]. However, the choice of chemotherapy is currently not influenced by patient’s sex based on the limited data and observed modest differences in chemotherapy efficacy for lung cancer patients.
Targeted therapies also have shown differential sex outcomes, particularly for the EGFR TKIs, gefitinib and erlotinib [71–73]. As mentioned previously, EGFR mutations are more frequently observed in lung tumors from female patients and the presence of these mutations confers a response to EGFR TKIs which is thought to account for the observed female response benefit [37, 38]. It is unknown whether there is a sex difference in response rate or overall survival in patients with EGFR TKI sensitizing mutations. Interestingly, a recently published pooled analysis of the EGFR TKI, afatinib, versus chemotherapy in the first-line setting in patients with advanced EGFR mutant demonstrated for the first time an overall survival benefit for an EGFR TKI. However, there was no difference in overall survival between men and women with EGFR mutations (exon 19 del or L858R), suggesting that there may not be a sex difference in terms of response or survival for EGFR mutant NSCLC patients [74]. Similarly, other targetable oncogenes such as ALK also display differential sex-specific alterations; however, improved response to ALK-targeted treatment in women has not been observed [75]. Vandetanib is a TKI with dual activity against both the VEGFR and EGFR pathways that failed to demonstrate a survival improvement in NSCLC. However, a trend for greater benefit in females was found in all treatment arms containing vandetanib across different trials [76, 77]. Sex may also influence radiation treatment outcomes. A meta-analysis demonstrated that female sex is associated with better survival following radiation treatment for lung cancer with male mortality rates 1.23 times higher than the mortality rate for women [78]. This was subsequently confirmed in another study of Stage IIIB NSCLC patients treated with radiotherapy, with or without chemotherapy [79]. The variation in response rates between men and women as observed with chemotherapeutic drugs, targeted TKIs, and radiotherapy is interesting but insufficient to allow the sex of the patient to guide therapeutic choices. All large trials in lung cancer should stratify patients according to sex, and as we move forward in an era of personalized medicine, understanding how lung cancer in men and women differs will be a critical factor in therapeutic choice .
Influence of Sex-Related Genetic Factors
Tobacco Carcinogen Metabolism
While a family history of lung cancer is an independent risk factor for lung cancer, genetic factors such as germline gene variants may play a role in the observed differential lung cancer survival rates between men and women. Pro-carcinogenic tobacco smoke constituents require activation by phase I enzymes (encoded by the CYP family of genes) for conversion to highly reactive carcinogens. Conversely, the phase II enzymes, including the glutathione S-transferases , are then responsible for detoxifying the activated forms of these polycyclic aromatic hydrocarbons. The reactive carcinogens that are not detoxified may bind DNA to form DNA adducts capable of inducing mutations and initiating the carcinogenesis. An appropriate balance between the phase I and phase II enzymes is necessary for optimum cellular protection from carcinogens. Metabolic activity of these enzymes can be altered by genetic polymorphisms and numerous studies have been reported examining their association with lung cancer yielding variable results [80]. In addition, these genetic polymorphisms may have distinct phenotypic outcomes in men and women.
Among the carcinogen-metabolizing enzymes, the sex-specific roles of genetic polymorphism in cytochrome P450 1A1 (CYP1A1) and glutathione S-transferase M1 (GSTM1 ) have been the most widely studied. Several studies have suggested that polymorphisms in these genes may help explain the significantly higher level of pulmonary DNA adducts found in female lung cancer patients compared to men [13–15]. Female lung cancer patients with an isoleucine to valine substitution in exon 7 of the CYP1A1 gene or a GSTM1 null phenotype had an increased lung cancer risk compared to men with these variants [81, 82]. In addition, female lung cancer patients were more likely than male lung cancer patients to have both variant genotypes (CYP1A1/GSTM1 null) and this combination resulted in an odds ratio of 6.54 for women compared to only 2.36 for men, independent of age and smoking history [81]. Clearly, regulation of carcinogen-metabolizing genes expressed in the lung is impacted by sex-related factors.
Interestingly, the CYP1A1 and GSTM1 genes not only are key players in tobacco carcinogen metabolism pathways but also play a role in estrogen metabolism [83]. Estrogen metabolism to the highly oxidative catechol estrogens is a known cause of DNA damage. The association of polymorphisms in estrogen and tobacco metabolism and DNA repair pathway genes was reported by Cote et al. [83, 84]. In this large population-based study, female smokers carrying at least two at-risk alleles had an increased risk of lung cancer compared to those without any of the risk alleles suggesting a complex interaction between altered estrogen biosynthesis, tobacco carcinogen metabolism, and the inability to repair DNA damage. Not only can estrogens act as substrates for the phase I enzymes and cause DNA damage by way of estrogen oxidation products, but estrogens can also stimulate lung tumor proliferation and cellular responses.
DNA Repair Capacity
Another possible explanation for the better survival outcome in women is the decreased DNA repair capacity (DRC) in women leading to lung cancers in females being more responsive to platinum-based chemotherapies . Supporting this explanation, DNA repair machinery has been shown to be more defective in women, contributing to the hypotheses that women are more susceptible to tobacco carcinogens and also more responsive to systemic chemotherapies. Suboptimal DRC has been linked to increased lung cancer risk and a lower DRC has been observed in younger female patients and in patients with a family history of lung cancer [85]. These findings were subsequently confirmed in a larger case–control study where the mean DRC was significantly lower in women compared to men [86]. This variation in DRC is most likely attributed to genetic variants in DNA repair genes and several suggestive genetic determinants affecting the DRC phenotype have been identified [87]. Clearly, further characterization of the functional relevance and sex-specific phenotypes of these genetic variations is warranted.
Recent studies have focused on identification of associations between polymorphisms in carcinogen metabolism and DNA repair genes and the development of EGFR and other targetable gene mutations. It has been shown that the exon 19 EGFR deletion is associated with polymorphisms in a number of genes related to mutagen detoxification as well as DNA repair in never-smoking lung adenocarcinoma patients [88]. Furthermore, the number of risk alleles in these genes correlated to an increase in the frequency of the EGFR exon 19 deletion, particularly in female lung adenocarcinoma patients.
Gastrin-Releasing Peptide Receptor
Another potential genetic factor that has been hypothesized to contribute to the increase of lung cancer in women is the gastrin-releasing peptide receptor (GRPR) gene. GRPR is activated through bombesin-like peptides to induce cell proliferation in bronchial epithelial cells which contributes to the lung carcinogenic process. Interestingly, GRPR is found on the X chromosome and is known to escape X inactivation so that women have two actively transcribed alleles [89]. Shriver et al. reported that GRPR mRNA expression in bronchial tissue was associated with an increased risk of lung cancer in female never smokers compared to male never smokers in a study of 78 subjects [90]. However, in a more recent larger case–control study which included 331 subjects, GRPR was reported to be increased in normal bronchial epithelia in lung cancer cases compared to cancer-free controls and this association was most profound in never smokers or former smokers with similar risk to both sexes [91]. While GRPR overexpression in normal epithelial mucosa appears to be a candidate risk factor for lung cancer with limited tobacco exposure, this genetic factor does not likely contribute to sex-related lung cancer biology as originally hypothesized.
Role of Estrogen in Lung Cancer Risk
Hormone Replacement Therapy and Lung Cancer Risk
The observations of sex differences in lung cancer presentation and survival suggest a role of estrogens in lung cancer. Support for a role for estrogens in lung cancer comes from several population studies which examined the use of hormone replacement therapy (HRT) and risk of lung cancer incidence and death. The link between HRT use and increased lung cancer risk was first suggested in the late 1980s in a small population-based case–control study of 672 women [92] followed by a larger population study of 23,244 women [93]. Additional evidence of an increase in lung cancer risk in women using HRT was a small case–control study that demonstrated a positive interaction between HRT use, smoking, and lung cancer development [94]. Ganti et al. reported a significant association with a shorter OS in lung cancer patients who received HRT around the time of diagnosis versus those who did not and the effect was most pronounced in women who smoked suggesting an interaction between estrogen and tobacco carcinogens [95]. Several studies reported either no association between HRT use and increased lung cancer risk or a protective effect [96–102]. There are many possible explanations for the inconsistencies in these studies including the type of HRT used, duration of use, time of use relative to lung cancer diagnosis, or genetic variation in study populations.
Recent studies have examined specific HRT type including combined estrogen plus progestin therapy versus estrogen alone. A randomized double-blind placebo-controlled trial in over 16,000 postmenopausal women who either received estrogen plus progestin HRT or placebo for 5 years was conducted by the Women’s Health Initiative. In this study, a significant negative effect on survival was observed for the HRT group with a trend toward in increased lung cancer diagnoses compared to the placebo group [103]. The combined hormone therapy significantly increased mortality from NSCLC; however, there was no effect on deaths from SCLC ([103] and Fig. 7.3). In a separate study conducted by the same group, the use of estrogen alone did not increase lung cancer incidence or death in more than 10,000 postmenopausal women who were randomized to receive estrogen alone or placebo [104]. In the Vitamins and Lifestyle Study, a large prospective population-based cohort of 36,588 peri- and postmenopausal women, combined estrogen plus progestin HRT use was associated with increased lung cancer risk that was duration dependent with the highest risk observed with 10 or more years of HRT use [105]. Although the association of estrogen plus progestin with cancer death was not analyzed in this study, a positive association between combined HRT use and advanced stage disease was found. Estrogen use alone had no effect on lung cancer risk in this study. The biological mechanisms responsible for this association are highly complex, most likely involving genetic and environmental interactions, and warrant further investigation.
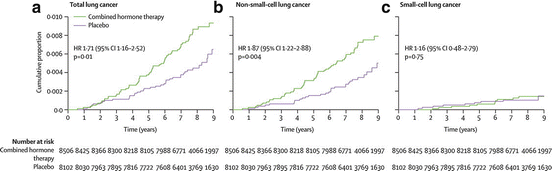
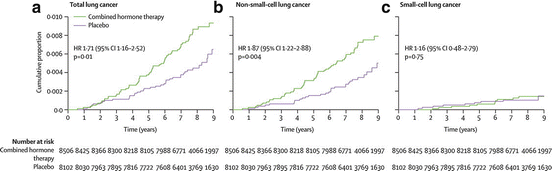
Fig. 7.3
Kaplan–Meier cumulative hazard for death from lung cancer by category, randomization group, and time in the trial. Hazard ratios (HR), 95 % confidence interval (CI), and P-values are from Cox proportional hazards regression models, stratified by age and dietary modification randomization group. (Reprinted with permission from Chlebowski et al. [103]
Anti-estrogens Protect Against Lung Cancer Mortality
Further evidence for a role of estrogens in lung cancer is that anti-estrogen usage may influence survival in lung cancer patients. The association of lung cancer incidence and mortality with anti-estrogen use was evaluated in 6655 women with breast cancer registered in the Geneva Cancer Registry [106]. This study reported a fivefold lower lung cancer mortality in breast cancer patients who received anti-estrogen treatment compared to the expected mortality rate in the general population while lung cancer incidence was not affected [106]. A separate retrospective population-based study using data from the Canadian Manitoba Cancer Registry reported similar results [107]. In this study of 2320 women diagnosed with NSCLC, use of anti-estrogens both before and after lung cancer diagnosis was significantly associated with a decrease in lung cancer mortality [107]. Early phase clinical trials evaluating the use of anti-estrogen therapy for lung cancer treatment are discussed below .
Estrogen Receptors and Local Estrogen Synthesis in Lung Carcinomas
Estrogen receptors (ERs) mediate the biological effects of estrogen. The two main ER isoforms, ERα and ERβ, are encoded by separate genes and have differential tissue distribution and functions [108]. Increasing evidence demonstrates that these and other steroid hormone receptors are present and functional in many tissues outside of the reproductive system, including lung. In contrast to the predominant expression of ERα in breast cancer, ERβ is the predominant ER expressed in lung tumors from both males and females [98, 109–114]. Reported expression of ERα in lung tumors is highly variable but is generally expressed less frequently compared to ERβ [110, 111, 113–116]. Furthermore, comparison of ERα and ERβ selective agonists demonstrates that the biological effects are predominantly mediated by ERβ in the lung [117]. ER expression is localized to both nuclear and cytoplasmic compartments in lung tumors and is found in tumors from both male and female patients. Expression in both cellular locations is thought to be important because estrogen exerts its effects through both genomic and non-genomic mechanisms in the lung (Fig. 7.4; Reviewed in [118]). Genomic effects of estrogen include ligand-dependent activation of nuclear ERs followed by modulation of estrogen-responsive genes including genes involved in cell proliferation. Non-genomic extranuclear estrogen signaling occurs through rapid activation of the EGFR and subsequent activation of the ERK and AKT downstream signaling pathways.
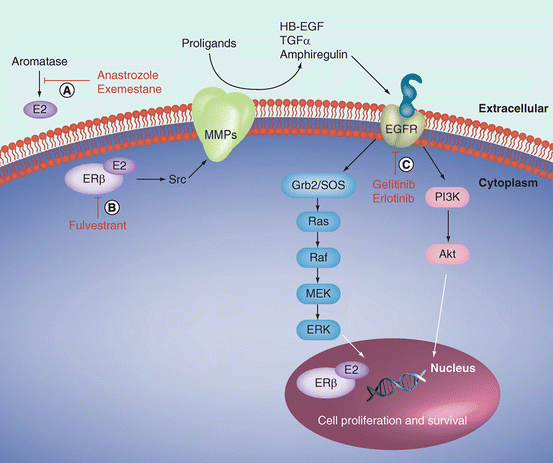
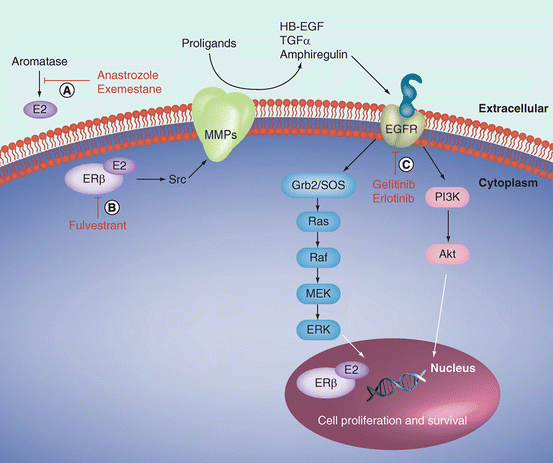
Fig. 7.4
Genomic and non-genomic estrogen signaling in lung cancer. Nuclear ERs are activated in a ligand-dependent manner by E2 binding to nuclear ERs at estrogen-responsive elements or AP-1 sites in the promoters of estrogen-regulated genes. Non-genomic estrogen action occurs through rapid activation of EGFR in the cell membrane, which occurs through activation of Src and MMP cleavage of the EGFR proligands to activate EGFR and the ERK and Akt downstream signaling pathways. Both of these mechanisms of estrogen action in lung cancer lead to cell proliferation and survival. Therapeutic approaches to target the estrogen signaling pathway include (a) inhibition of estrogen synthesis with aromatase inhibitors, such as anastrozole or exemestane; (b) downregulation of ERs using antiestrogens such as fulvestrant; and (c) targeting growth factor pathways that are activated by estrogens with agents such as gefitinib or erlotinib. These strategies can be used as single agents or in combination. (Reprinted with permission from Burns and Stabile [118])
While numerous studies have examined ER tumor expression status in relation to lung cancer survival, there is still not a clear consensus. Nuclear ERβ expression in lung tumors was found to be a positive prognostic indicator in some studies [111–113, 115, 119]. In some reports, the prognostic significance of ERβ was only observed in male patients [111, 115] or was limited to a subset of lung cancer patients with EGFR mutations [119]. The discovery of sub-isoforms and distinct cellular localizations of both ERα and ERβ has further complicated our understanding. Isoform specificity was reported in a study which demonstrated that ERβ-1, but not ERβ-2, was related to poor prognosis of female early stage lung cancer patients [120]. Furthermore, cytoplasmic ERβ-1 expression was found to be a strong negative prognostic indicator for both males and female lung cancer patients [110]. Conversely, nuclear ERβ-1 was also linked to poor survival but only in the metastatic setting [121]. Furthermore, high cytoplasmic expression of other ERβ isoforms, ERβ-2 and ERβ-5, was positively correlated with survival [122]. High ERβ-mediated bioactivity in patient sera also predicted poor lung cancer survival; however, isoform specificity of this effect is not known [123]. ERβ has also been shown to localize to the mitochondria in lung cancer cells and a novel ligand-independent anti-apoptotic role of mitochondrial ERβ has been identified [124]. The role of ERα in lung cancer outcome is also controversial with some reports demonstrating that ERα has no effect while others report a correlation of high expression with poor prognosis [110, 112, 113]. Standardization of immunohistochemical procedures and criteria for positivity will be necessary to completely understand the role of ERs in lung cancer.
Lung cancer cells also have the ability to synthesize estrogen locally through the action of the aromatase enzyme which catalyzes the conversion of androgens to estrogen and β-estradiol [125, 126]. Several studies have suggested a role for intratumoral estrogen synthesis in lung tumorigenesis. Intratumoral estradiol levels were positively correlated with aromatase expression as well as lung tumor size and estradiol concentrations were significantly higher in lung tumor tissue compared to normal lung tissue from the same patient [125]. In a separate report, aromatase expression was higher in metastases than in the corresponding primary lung tumor [127]. Furthermore, in postmenopausal women with early stage lung cancer, high aromatase tumor expression predicted poor survival [128]. A high occurrence of co-expression of aromatase and ERβ has also been observed in lung tumors [129] and this co-expression was a strong negative lung cancer survival predictor in both men and women, especially strong in the postmenopausal female group [130]. In a separate study that included both women and men with all stages of lung cancer, aromatase expression was not predictive alone but was correlated with poor survival when combined with other hormonal markers including ERβ and progesterone receptor [110]. Interestingly, aromatase protein has been found to be localized to both the epithelial cells in lung tumors as well as in infiltrating macrophages, suggesting that release of estrogen might affect the microenvironment [131]. Other enzymes involved in intratumoral estrogen production and metabolism have also been investigated and 17β-hydroxysteroid dehydrogenase type 1 was identified as negative prognostic indicator of lung cancer surviva l [132].
Targeting the Estrogen Pathway for Lung Cancer Therapy
Preclinical Rationale for Targeting the Estrogen Pathway in Lung Cancer
A series of observations have supported a role for estrogen receptor in lung cancer tumorigenesis both in vitro and in vivo. First, NSCLC proliferation is increased with estrogen treatment both in vitro [109, 133] as well as in human NSCLC xenografts [109], and in a genetically defined mouse model of lung adenocarcinoma [134]. Furthermore, pharmacologic inhibition of the estrogen pathway with either the anti-estrogen fulvestrant or aromatase inhibitors inhibited NSCLC tumor growth in vitro and in human NSCLC xenograft models [109, 126, 127]. In addition, activity has also been observed with combination therapies targeting the estrogen pathway and other growth factor receptor pathways in cancer.
The estrogen receptor through primarily non-genomic mechanisms can positively regulate the EGFR, insulin-like growth factor 1 receptor (IGFR-1 ), and vascular endothelial growth factor receptor (VEGFR) signaling pathways in lung cancer (reviewed in [118] and Fig. 7.4 .). Several studies have demonstrated that EGFR TKIs can synergize with the anti-estrogen fulvestrant or aromatase inhibitors in vitro and in vivo [133, 135–138]. Furthermore, similar efficacy was seen with the combination of fulvestrant with IGF-1 TKIs or the multitargeted VEGFR TKI, vandetanib [127, 139, 140].
In addition to the treatment studies above, the role of anti-estrogen therapy in lung cancer prevention has also been examined [131]. In a recent study, a tobacco carcinogen-induced animal model of lung cancer was utilized to address two critical questions. First, could the combination of the aromatase inhibitor, anastrozole, and anti-estrogen, fulvestrant, inhibit precancerous changes? Second, could this combination prevent progression of precancerous lesions to lung carcinoma? Although single-agent activity was observed with either agent, the combination was superior both in reducing precancerous lesions and in preventing progression. These preclinical studies as well as the correlative and epidemiologic studies described above support a critical role for the estrogen signaling pathway in lung tumorigenesis. As such, targeting the estrogen signaling pathway may represent a novel and effective therapeutic strategy for lung cancer. Although the use of anti-estrogen therapy in lung cancer is novel, anti-estrogen therapy in cancer is not a new concept but rather a well-accepted standard of care in hormone positive breast cancer.
Clinical Trials of Anti-estrogen Therapy in Lung Cancer
The large clinical experience with anti-estrogens in breast cancer has served as a model for the current ongoing development in NSCLC. The use of anti-estrogen therapy in hormone positive breast cancer in both the adjuvant and metastatic setting is standard of care and, since the late 1980s, has led to an almost 30 % decrease in breast cancer mortality rate [141, 142]. A number of studies over several decades have led to the development of several classes of anti-estrogens which are being tested in lung cancer (Fig. 7.4.). The first class of anti-estrogens, selective estrogen receptor modulators (SERMs ) such as tamoxifen, are used in both adjuvant and metastatic setting [141]. The second class of anti-estrogens, aromatase inhibitors, work through inhibition of the peripheral conversion of androgens to estrogen [143]. As such, use of AIs in the premenopausal setting without ovarian suppression is ineffective and likely detrimental. In addition to SERMs and AIs, fulvestrant represents a third class of anti-estrogen therapy which leads to the direct degradation of ER and is utilized in the second-line setting for advanced breast cancer. The use of this compound has increased significantly in recent years following the recognition that it is much more clinically efficacious at a higher dose than its original FDA-approved dose [144].
The first clinical trial examining the role of anti-estrogen therapy in lung cancer opened in 2004 and over the last decade the study of anti-estrogen therapy in lung cancer has evolved and expanded. To date, two early phase clinical trials NSCLC have been completed [145, 146] and several more are under way (NCT01556191, NCT01664754) or in the planning stages. Based on the preclinical data suggesting that dual inhibition of the estrogen and EGFR pathways led to increased efficacy, these initial trials have looked at the combination of the anti-estrogen, fulvestrant, with the EGFR TKIs, gefitinib or erlotinib, respectively.
Results of the first Phase I trial of anti -estrogen therapy in lung cancer were published in 2009 and demonstrated safety and potential efficacy [146]. This trial tested the combination of fulvestrant and the EGFR TKI, gefitinib, in postmenopausal women with advanced NSCLC regardless of histology or lines of therapy. Of the 20 patients evaluated for response, the therapy was well tolerated and three partial responses (PRs) were observed (response rate of 15 %, 95 % CI: 5–36 %). However, one of these responses was found in a patient with a TKI sensitizing EGFR mutation which predicts for response to gefitinib alone. The observed median progression-free survival (PFS) of 12 weeks, overall survival (OS) of 38.5 weeks, and estimated 1-year OS of 41 % (95 % CI: 20–62 %) were encouraging given that this was a heavily pretreated population. Furthermore, a preliminary biomarker analysis suggested that high ERβ nuclear staining may predict for increased survival (OS 65.5 weeks ≥60 % ERβ nuclear IHC staining vs. 21 weeks if <60 % ERβ nuclear IHC staining) although these results did not reach statistical significance in this small trial.
< div class='tao-gold-member'>
Only gold members can continue reading. Log In or Register a > to continue
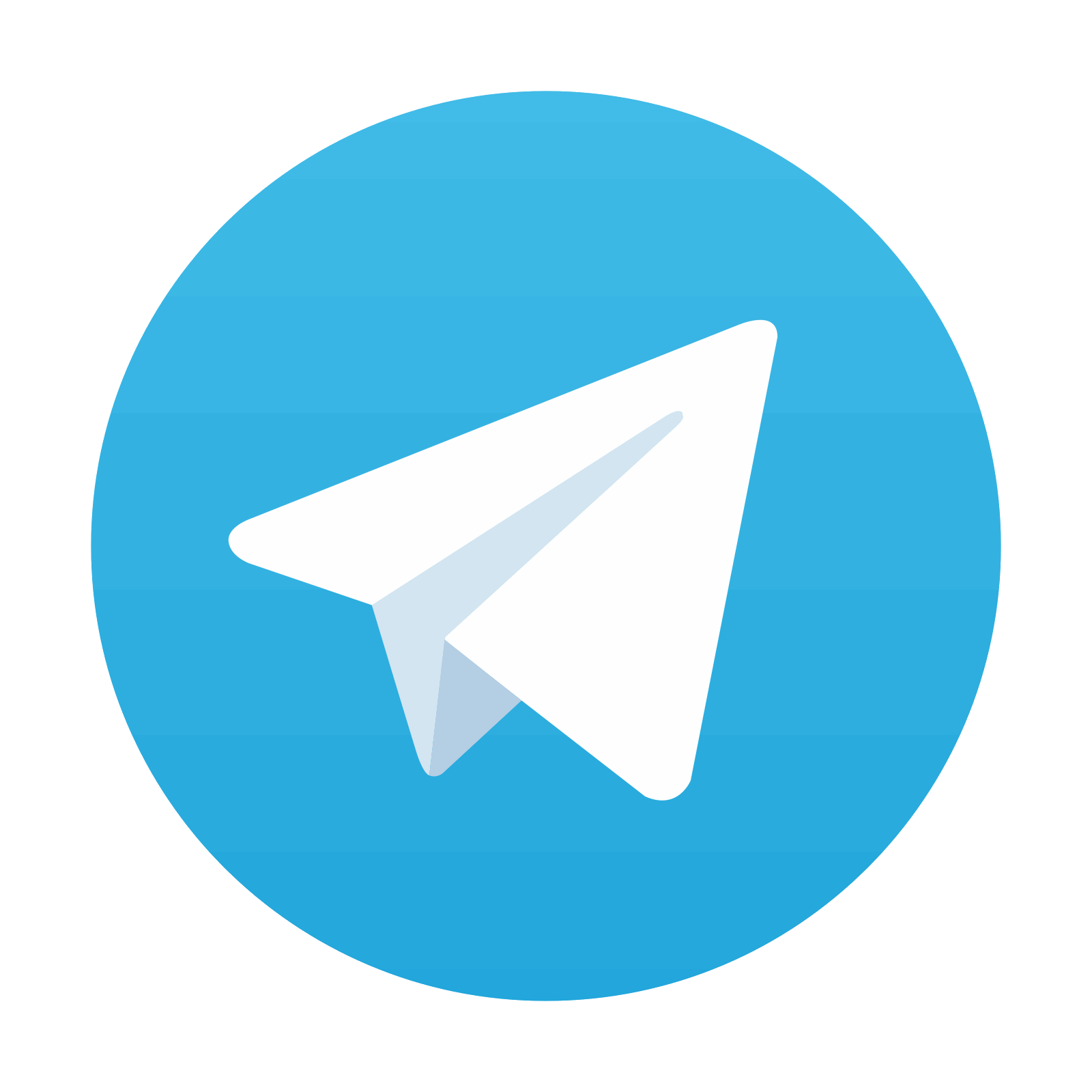
Stay updated, free articles. Join our Telegram channel

Full access? Get Clinical Tree
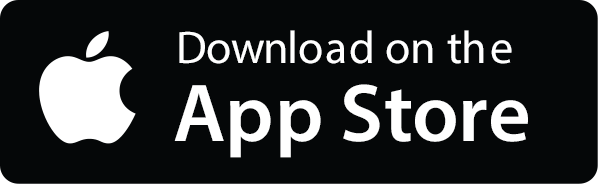
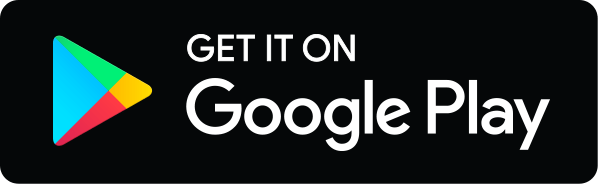