Fig. 4.1
Prevalence of asthma in females and males at various ages . Graph is adapted from Carey, M. et al. [2]
Women with asthma also have increased asthma-related mortality and morbidity , more severe asthma phenotypes, less responsiveness to corticosteroid medications, and more fluctuations in asthma symptoms, corresponding to the menstrual cycle, compared to men with asthma [5, 7, 8]. As children, boys are twice as likely as girls to be hospitalized for an asthma exacerbation (as reviewed in [9]). After the age of 15, however, girls and women are three times more likely than boys and men to be hospitalized for an asthma-related event [10–12]. The sex differences in hospitalizations of adult men and women with asthma are independent of controller medication use or length of time before seeking medical attention [9]. Combined, these data suggest that asthma prevalence and the severity of asthma symptoms and exacerbations of asthma are increased in women compared to men. In this chapter, we will discuss the findings from epidemiological, clinical, and molecular studies exploring the role of sex hormones and asthma.
Classifications Systems for Patients with Asthma
Asthma is a heterogeneous disease with many different phenotypes responsiveness to current therapies, and triggers for exacerbations [13]. Patients with asthma were classified as extrinsic (allergic) and intrinsic (nonallergic) based on clinical symptoms, IgE serum levels, atopy status, and age of onset [14]. Using this classification system, women had an increased prevalence in both allergic and nonallergic asthma compared to men. However, other phenotypic variables, such as forced expiratory volume in one second (FEV1) pre- and post-bronchodilator, blood and sputum eosinophil and neutrophil counts, responsiveness to corticosteroids, age of onset, and asthma triggers, were not included when categorizing patients [14]. Not accounting for multiple variables resulted in patients with varying clinical symptoms, pathophysiology, and molecular markers being classified as having either allergic or nonallergic asthma. This categorization system created difficulty in determining the appropriate clinical trial populations for therapeutics targeting a specific mechanism for airway inflammation .
Recently a more sophisticated systems biology approach utilizing cluster modeling classification systems was used to categorize patients with asthma. Cluster analysis uses algorithms and a predefined set of asthma variables from patients with asthma and healthy controls to define asthma phenotypes [15–19]. Common variables used in these studies are shown in Table 4.1, and several groups have reported a female dominance in the clusters listed below [15–19]:
Age of onset |
Gender |
BMI |
Lung function testing |
Allergies |
Serum IgE levels |
Sputum eosinophil and neutrophil counts |
Medication use |
Asthma symptom score questionnaires |
Use of health care for asthma symptoms |
Hospitalization for asthma |
1.
Early-onset atopic asthma
2.
Later onset atopic asthma
3.
Later onset asthma in obese individuals
4.
More severe asthma with increased sputum neutrophils and decreased lung function
As denoted in the list above, cluster analysis statistical approaches have categorized women-dominated asthma phenotypes spread across the asthma spectrum; from early-onset mild, intermittent asthma to late-onset, severe asthma. To better understand these cellular and molecular variables used in cluster analysis studies, we will first review the basic immune mechanisms and cell types involved in asthma and then discuss findings from the literature. Defining the mechanisms and properly phenotyping patients will target patient populations that may benefit from various therapeutics when conducting clinical trials and designing treatment regiments.
Host Immune Responses in Asthma
Sir William Osler described asthma in the 1892 edition of Principles and Practice of Medicine as a disease with a genetic component , originating early in life that resulted in spasms of the bronchial muscles, swelling of bronchial mucous membrane, inflammation in bronchioles, and various circumstances that induced symptoms [20]. Approximately 70–80 % of patients with asthma are sensitized to allergens, pollen, dust mites, cockroach antigen, mold, etc., and asthma symptoms and exacerbations are increased with exposure to these allergens in these patients. However, other environmental stimuli , including viral infections, cigarette smoke, and air pollution, also may result in asthma symptoms through different innate and adaptive immune responses . In this chapter, we will primarily discuss findings focused on the role of sex hormones in allergic asthma immune pathways (as shown in Fig. 4.2 ), but we will also introduce alternative, nonallergic immune pathways involved with asthma pathogenesis.
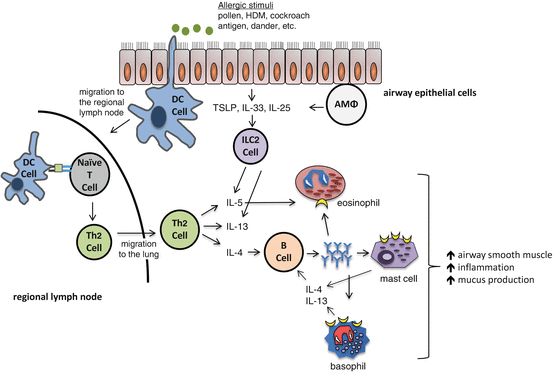
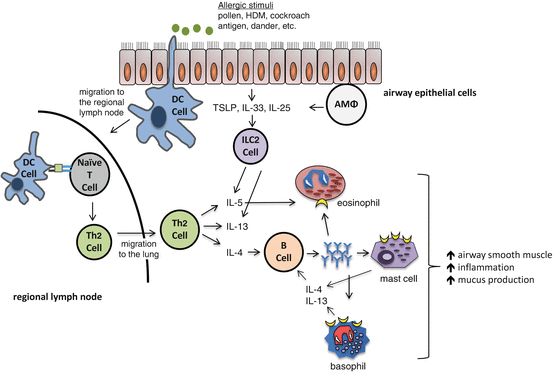
Fig. 4.2
Immune response to allergic stimuli resulting in increased AHR, allergic airway inflammation, and mucus production. Alveolar macrophage (AMΦ), dendritic cell (DC), house dust mite (HDM)
The allergic airway immune response is characterized by increased CD4+ Th2 cells, eosinophils, mast cells, and basophils in the airway. Airway epithelial cells are the first point of contact for airway allergens, and airway epithelial cells are important for regulating antigen-presenting cells (APCs) . APCs, including dendritic cells (DCs), alveolar macrophages (AMΦ), and B lymphocytes, engulf antigens and process them for presentation to the T cells, initiating the adaptive immune response [21, 22]. Antigens processed by APCs, through the endocytic pathway, are presented by major histocompatibility complex (MHC) class II molecules to CD4+ T lymphocytes. Naïve CD4+ T lymphocytes are activated when two events occur: (1) the antigen/MHC class II complex on the APC engages the T cell receptor, and (2) co-stimulation molecules, such as CD80 or CD86, on the APC bind to co-stimulation molecules, such as CD28, on the CD4+ T cells. DCs are the most potent APCs in activating naïve T cells [23, 24], and DCs also secrete chemokines that attract CD4+ Th2 differentiated cells and eosinophils to the lung [25].
Many cell types are involved in the allergic response, but the key cell types in initiating and amplifying the allergen-induced inflammatory cascade are the antigen-presenting DC and T helper 2 (Th2) lymphocytes. Th2 lymphocytes are CD4+ T cells that are differentiated from activated naïve T cells. Initiation of Th2 cell differentiation is not completely understood, but interleukin-4 (IL-4) is also required for Th2 cell differentiation [26, 27]. Th2 cells, as well as mast cells and basophils, secrete IL-4, and IL-4 is required for B lymphocytes to isotype switch antibody production to IgE [26, 27]. Th2 cells additionally produce a variety of other pro-allergic inflammatory cytokines such as IL-5, IL-9, IL-10, and IL-13. IL-5 is an important eosinophil regulatory factor and increases eosinophil maturation and proliferation [28]. IL-13 is a central mediator in AHR and also induces mucus hypersecretion from goblet (mucous) cells in the airway [27–29]. IL-9 is important for mast cell growth, T cell proliferation, IgE secretion from B cells, and mucus hypersecretion from goblet cells [30]. IL-10 is an anti-inflammatory cytokine that is secreted by Th2 cells as well as other cells, including T regulatory cells and Th17 cells. In summary, IL-4, IL-5, IL-9, and IL-13 are important for establishing the allergic airway response, and IL-10 is an important anti-inflammatory cytokine in the airway allergen immune response.
The allergic response to an aerosolized allergen is also characterized by antigen-specific immunoglobulin E (IgE) production by B lymphocytes whereupon IgE can bind to the high-affinity IgE receptor, FcεR1, on tissue mast cells and peripheral blood basophils. Antigen cross-linking of the antigen-specific IgE/FcεR1 complex ignites degranulation of the mast cells and basophils, causing release of soluble mediators , such as histamine and tryptase [13, 31]. Other mediators, including prostaglandins and leukotrienes, are also released in response to an allergen [32, 33]. Release of these mediators causes vasodilation and recruitment of inflammatory cells into the airway, including eosinophils and lymphocytes. Therefore, antigen cross-linking to FcεR1 is an important component of the immune response to allergens.
Recently a new cell type, group 2 innate lymphoid cells (ILC2) , was described to be residing in the lung in small numbers and be important in airway immune responses. ILC2 are activated by IL-33, IL-25, and thymic stromal lymphopoietin (TSLP) which are secreted from airway epithelial cells and other immune cells, in response to allergens and other stimuli, such as infections with fungi, helminthes, and viruses [34–36]. ILC2 lack lineage markers for T cells, B cells, DCs, and macrophages [34, 37]. ILC2 express CD25 (IL-2Rα) and CD127 (IL-7Rα) as well as receptors for the ILC2 activating cytokines IL-25, IL-33, and/or TSLP [34, 36, 37]. Once activated, ILC2 quickly secrete large amounts of IL-13 and IL-5 compared to Th2 cells [37]. Therefore, while ILC2 are rare within the lung, ILC cytokine expression increases eosinophil infiltration into the lung and mucus production. Collectively, the immune response to aerosolized allergens causes an increase in airway inflammation, airway mucus hypersecretion by goblet cells (predominantly through IL-13), and increased airway smooth muscle constriction.
Understanding the basic immune pathways in allergic asthma is a first step in determining the role of sex hormones on asthma. Another important step is determining the expression of estrogen receptors (ERs), progesterone receptors (PRs), and androgen receptors (ARs) on immune cells. Nuclear ERs, PRs, and ARs are readily expressed in inflammatory cells involved with asthma (as reviewed in [38]). Membrane-bound ERs are not found on T cells or cells of hematopoietic origin, but membrane-bound ERs are found on other cell types [38]. Since sex hormone receptors are found on many different immune cells, sex hormones may affect several steps in allergic airway disease, including antigen uptake, antigen processing and presentation, adaptive immune effector function, antibody isotype switching to IgE, and mast cell degranulation. Throughout this chapter we will discuss the role of sex hormones in the allergic immune response.
Changes in Sex Hormones during Puberty, Pregnancy, and Menopause Adversely Affect a Subset of Women with Asthma
As demonstrated in Fig. 4.1 the prevalence of asthma increases about the age of menarche, suggesting a role for sex hormones in asthma prevalence. Salam and colleagues reported girls who reached menarche prior to age 12 had a 2.08 higher risk for asthma compared to girls who reached menarche at age 12 or later [39]. These data suggest that ovarian hormones increase the risk for development of asthma. In the following subsections, we will discuss how various stages in life and fluctuations of hormones affect asthma prevalence and severity.
Pre- and Perimenstrual Asthma Symptoms
Twenty to thirty-five percent of women with asthma have pre- or perimenstrual worsening of asthma, but asthma exacerbations were independent of cyclic hormonal changes [40–44]. Estrogen and progesterone concentrations oscillate throughout with menstrual cycle, and these fluctuations in hormonal concentrations are thought to be potentially linked to changes in asthma symptoms. As shown in Fig. 4.3, estrogen concentrations peak on day 13 of a 28-day menstrual cycle, just prior to ovulation, and progesterone concentrations peak at days 22–24, four to six days prior to menses. Asthma symptoms are increased in the premenstrual phase of the cycle (days 25–28) when both estrogen and progesterone concentrations are low (Fig. 4.3). Clinical studies tracking asthma symptoms and peak expiratory flow rates in women through at least two menstrual cycles found increased symptoms and decreased peak flow rates in the morning during the premenstrual and perimenstrual phases of the cycle compared to other phases of the cycle [40, 43]. Women with premenstrual asthma symptoms had increased fractional exhaled nitric oxide (FeNO) , a noninvasive measure of epithelial induced nitric oxide that correlates with eosinophilic inflammation [45], and eosinophils in the sputum in the premenstrual phase compared to the seventh day of their cycle [46]. However, no difference in the phase of the menstrual cycle of women requiring emergency department visits for asthma symptoms was reported [44]. Combined, these studies suggest a subset of women with asthma have increased premenstrual asthma symptoms and decreased peak expiratory flow rates, but no change in asthma exacerbations during premenstrual phase of their cycle.
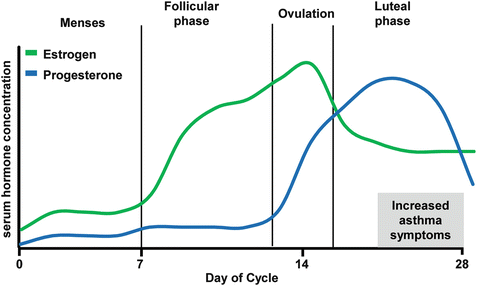
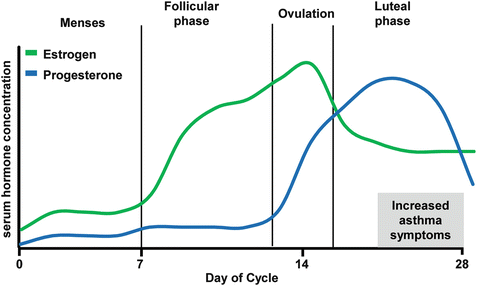
Fig. 4.3
Flucuations in estrogen and progesterone serum concentrations through a 28-day menstrual cycle. Estrogen and progesterone serum concentrations vary throughout the menstrual cycle
Use of certain hormonal birth controls pills reduced the fluctuations of hormone levels and could potentially reduce pre- and perimenstrual asthma symptoms in women with asthma.
However, only a few studies, with small patient sizes, have been conducted to determine the effect of hormonal birth control medications on asthma symptoms through the menstrual cycle. One study followed 18 women with asthma through two menstrual cycles. Women not taking oral contraceptives (n = 9) had increased AHR to adenosine monophosphate during the luteal phase and reduced diurnal peak expiratory flow rates. However, women taking oral contraceptives medications (n = 9) did not have a significant increase in AHR to adenosine monophosphate or significant changes in morning and afternoon peak flow rates [47]. Another study followed 28 women with asthma for 12 weeks (2–4 menstrual cycles) and found no difference in asthma symptoms in women taking oral contraceptives versus women not taking oral contraceptives [48]. Therefore, additional studies, with larger patient populations that stratify different types of hormonal birth control medications, are needed to fully delineate if asthma symptoms are reduced in women taking hormonal contraceptives.
Pregnancy and Asthma
Asthma is a common chronic condition present during pregnancy with a 3.7–8.4 % prevalence among pregnant women [49]. Variations in asthma symptoms and asthma control during pregnancy are common. Two studies from the late 1980s reported that in pregnant women with asthma approximately one-third had decreased asthma symptoms, one-third had increased asthma symptoms, and one-third had no change in asthma symptoms [50, 51]. Since that time, there has been little improvement on predicting if asthma symptoms will increase, decrease, or remain the same in pregnant women. Researchers have shown that compared to women with mild asthma phenotypes, women with severe asthma were more likely to experience increased asthma symptoms later in the pregnancy, in the second and third trimesters [52]. Pregnant women with asthma were also more susceptible to respiratory viral infections, including rhinovirus and influenza, and viral infections increase asthma exacerbations [53, 54]. Variations in asthma symptoms and control in pregnant women are not fully understood, but are likely related to various phenotypes of asthma, hormonal changes during pregnancy, and adherence to asthma medications. Many pregnant women with asthma decide, either individually or as advised by their medical provider, to reduce or stop asthma medications to prevent adverse side effects on the fetus. However, National Heart , Lung, and Blood Institute and the Global Initiative for Asthma (GINA) guidelines indicate that pregnant women should maintain their current regimen of asthma medications, including inhaled corticosteroids, long-acting beta agonist, leukotriene modifiers, theophylline, and oral corticosteroids [55, 56]. Use of asthma medications during pregnancy was not associated with fetal abnormalities [57], and maintaining asthma control during pregnancy is important. More severe asthma, poorly controlled asthma, and asthma exacerbations during pregnancy are associated with increased risk for development of preeclampsia and gestational diabetes in the mother and preterm birth, low birth weight, and perinatal mortality for the baby [55, 57, 58].
Menopause and Asthma
As described above, ovarian hormones are associated with asthma. Therefore, one would predict that the decline in circulating ovarian hormones that occurs with menopause leads to decreased asthma in women. However, studies in menopausal women report variable findings, with asthma symptoms increasing, decreasing, or remaining the same when compared to premenopausal women or postmenopausal women on hormone replacement therapy (HRT) (as reviewed in [59]). The US Nurses’ Health Study was a prospective study which followed 41,202 premenopausal women and 23,035 postmenopausal women from 1980 until 1990 [6]. Women were reclassified as pre- or postmenopausal every 2 years based on questionnaire responses. At the end of the study, 582,135 person-years were documented with 726 new cases of asthma. The US Nurses’ Health Study determined that postmenopausal women who never used HRT had a significant decrease in the risk of developing asthma compared to premenopausal women [6]. Further, postmenopausal women who used HRT had an increased age-adjusted risk for asthma compared to postmenopausal women who never used HRT [6]. The European Community Respiratory Healthy Surveys (ECRHS I) also conducted a study and reported no difference in self-reported asthma between premenopausal and postmenopausal women not taking HRT [60]. However, the ECRHSII cross-sectional study reported increased asthma symptoms in women during the menopause transition (amenorrhea for 6+ months) compared to premenopausal women [61]. Further, the ECRHSII study reported increased asthma severity in women diagnosed with asthma during or after menopause [62]. Combined, these studies suggest menopause and HRT are involved in asthma pathogenesis, but the mechanisms are not completely understood. Co-variables, including BMI, genetic factors, estrogen production from adipose tissue, and insulin sensitivity, need to also be considered in future study design.
Summary
Changes in circulating sex hormones occur throughout life for women and therefore it is challenging to design clinical studies that determine the roles of sex hormones in asthma pathogenesis. Further complicating clinical trials is sex hormones regulate AHR, airway inflammation, and metabolism, important aspects in asthma pathogenesis. Therefore to define the mechanisms by which sex hormones regulate asthma pathogenesis researchers use asthma-like rodent (mainly mouse) models. We will discuss animal models of asthma in the next section.
Sex Hormones in Mouse Models of Asthma
Animal models which mimic asthma with increased airway inflammation, AHR, and mucus hypersecretion are vital for determining the mechanisms associated with asthma pathogenesis. Mouse models are used most readily due to reagent availability, knockout strains of mice, and genetically similar immune systems within inbred mouse strains. Sex differences and the role of sex hormones in mouse models of asthma have variable findings based on mouse strain, protocol used for inducing allergic airway inflammation, and endpoints analyzed. The subsections below describe the different findings in mice with regard to sex hormones at baseline (prior to inducing inflammation), during allergic airway inflammation, and during nonallergic airway inflammation.
Baseline AHR and Airway Physiology in Female and Male Mice
At baseline naïve mice have very few lymphocytes, neutrophils, and eosinophils in the bronchoalveolar lavage (BAL) fluid [63], and there is no sexual dimorphism in the percentages of baseline leukocytes in naïve mice [64]. However, baseline AHR is increased in male mice compared to female mice in both the BALB/c and C57BL/6 strains of mice [64]. ER-α-deficient mice (ER-α KO) have increased AHR compared to WT female mice [65, 66], and using an estrogen antagonist increased AHR compared to vehicle control [67]. Conversely, administering 17β-estradiol to male mice decreased AHR compared to vehicle control [67].
Researchers speculated that the increased AHR in male mice is due to male mice having decreased numbers of alveoli and decreased alveolar surface area compared to female mice [68]. To test this hypothesis, female mice were ovariectomized just after weaning (approximately 21 days) and similar alveolar structures were seen in ovariectomized female and male mice, suggesting sex hormones were important in the development of alveoli [68, 69]. Additional studies in ER-α and ER-β KO mice showed ER-α and ER-β signaling is required for fully developing alveolar structures in female mice, and that ER-β KO mice have decreased elastic recoil compared to WT female mice [69]. Increased numbers of alveoli and alveolar surface area may be responsible for the observed sex difference in AHR, and normalization for lung volume and size in a statistical model resulted in no difference in AHR between males and females.
Other factors including variations in vagal nerve-mediated pathways and smooth muscle contractility may also contribute to sex differences in AHR after stimulation. Vagal nerve-mediated pathways are increased in response to methacholine and carbachol, chemicals known to increase smooth muscle contractility, in male mice compared to female mice [70]. Gonadectomized male mice also had similar vagal nerve responses as intact female mice, and restoring androgens to gonadectomized male mice increased the vagal nerve response and AHR to methacholine [70], suggesting androgens increased the vagal nerve response. However, no difference in ex vivo smooth muscle contractility assays to carbachol was reported in tracheal rings from male and female mice. Further, ER-α KO mice and WT female mice had similar smooth muscle contractility to carbachol [70]. Combined, these results suggest sex hormones affect baseline AHR in mice independent of smooth muscle constriction.
Dryness of the throat and vaginal dryness are symptoms reported by women during menopause. These symptoms suggest that sex hormones may affect mucous cell metaplasia and mucus production in the airway and the reproductive system. Therefore, researchers became interested in the role of estrogen and progesterone on mucus production in the nasal and airway epithelial cells . In 1975, Helmi and colleagues administered 10 μg/day ethynyl estradiol, a downstream hormone of the 17β-estradiol, to guinea pigs and measured mucous cell hyperplasia and metaplasia in nasal epithelial cells by histochemical staining. Guinea pigs administered ethynyl estradiol had increased mucous cell hyperplasia and metaplasia compared to vehicle-treated animals [71]. In vitro studies in human airway or nasal epithelial cells also showed that estrogen or progesterone increased mucus production and the expression of the mucus proteins, Muc5AC and Muc5B, compared to vehicle-treated cells [72, 73]. In summary, sex hormones regulate baseline airway responsiveness to methacholine as well as mucus production. Acknowledging baseline differences is vital for experiment design and interpretation of data as normalizing experimental results to percent baseline may not provide the most accurate interpretation of data.
Establishment of Allergic Airway Inflammation in Mice
During ongoing allergic airway inflammation the role of sex hormones varies based on the protocol used to induce allergic airway inflammation and the endpoint measured. The results discussed in the paragraphs below are summarized in Table 4.2. Two widely used models of allergic airway inflammation are: (1) ovalbumin (OVA) sensitization followed by OVA challenge and (2) exposure to house dust mite (HDM) antigen. Both these models increase airway inflammation, AHR, and mucus hypersecretion through a CD4+ Th2 cell-mediated mechanism. Female mice undergoing the OVA sensitized and challenged protocol or the HDM protocols had increased eosinophilic infiltration and IgE serum levels compared to male mice undergoing the same protocol [74–76]. Female mice with OVA-induced allergic airway inflammation also had significantly increased lung IL-4, IL-5, and IL-13 protein expression, mucous cell metaplasia, and airway remodeling compared to male mice [74–76]. Further, castration of male mice prior to OVA sensitization and challenge increased eosinophils and lymphocytes in the BAL fluid, mucous cell metaplasia, and airway inflammation compared to sham-operated mice [75]. However, an ovariectomy prior to OVA sensitization decreased OVA-induced airway inflammation and AHR compared to intact female BALB/c mice [77]. These results suggest that ovarian sex hormones increased, while testosterone decreased, allergic airway inflammation, AHR, and mucous cell metaplasia.
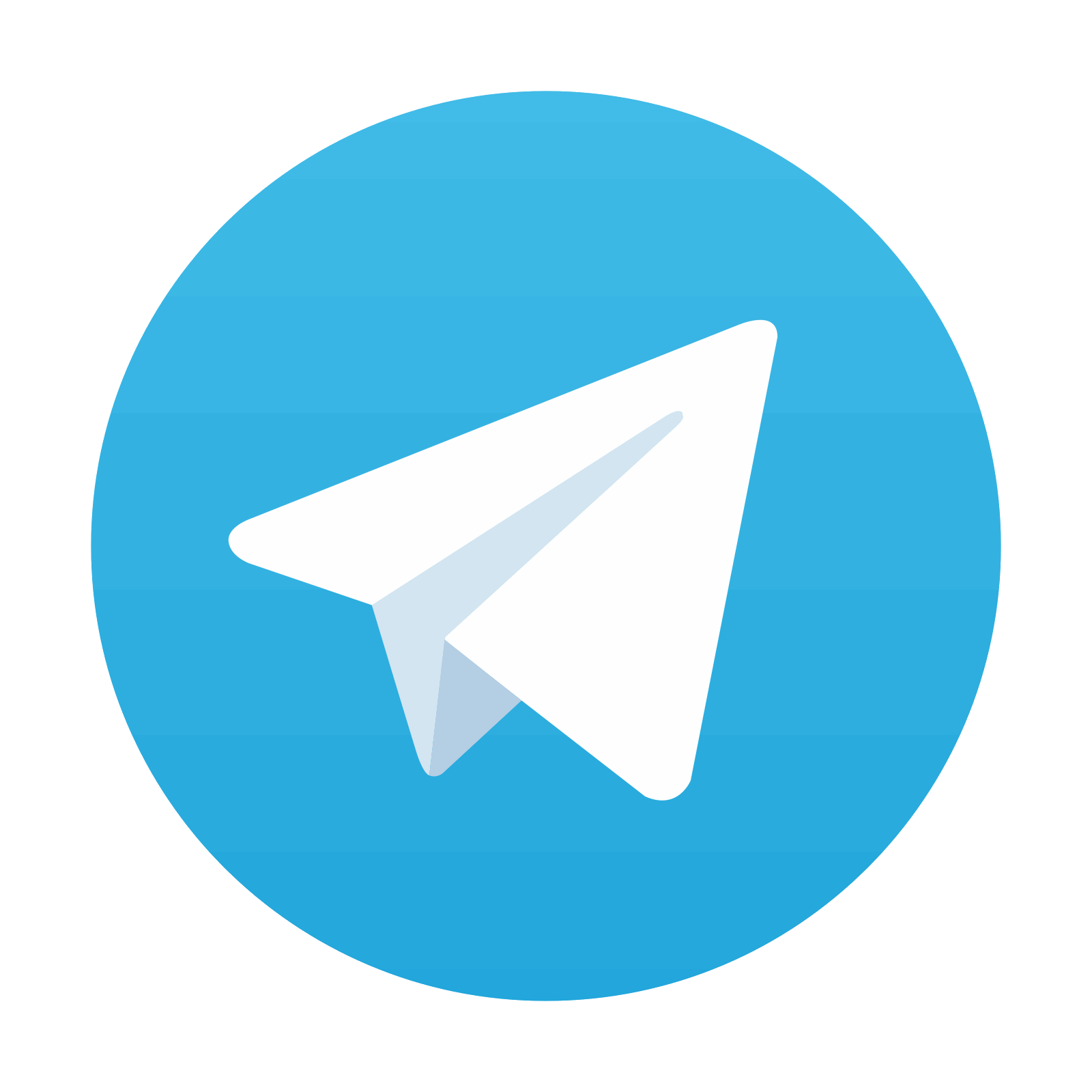
Table 4.2
Summary of results for the role of sex hormones in mouse models of allergic airway inflammation
< div class='tao-gold-member'>
Only gold members can continue reading. Log In or Register a > to continue
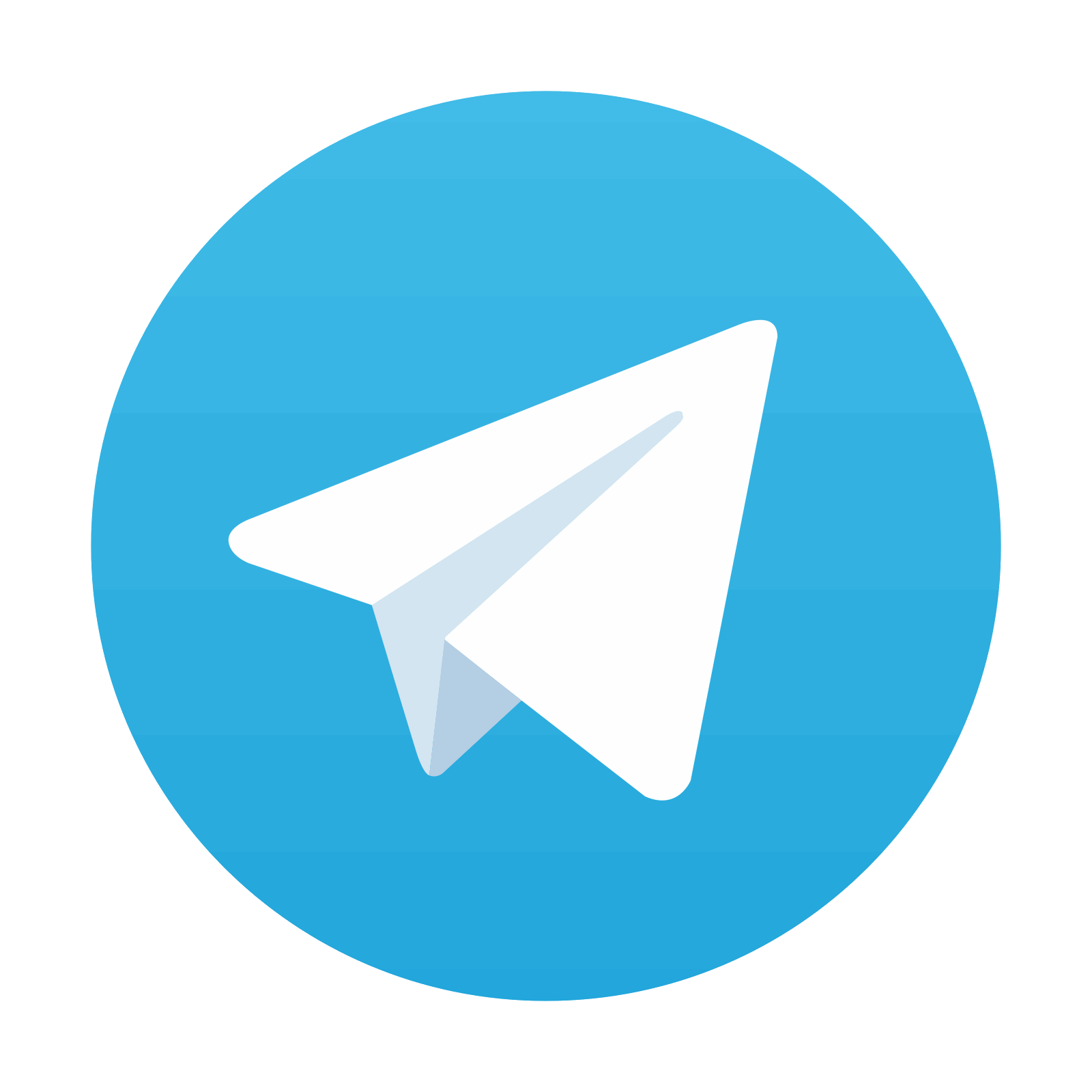
Stay updated, free articles. Join our Telegram channel

Full access? Get Clinical Tree
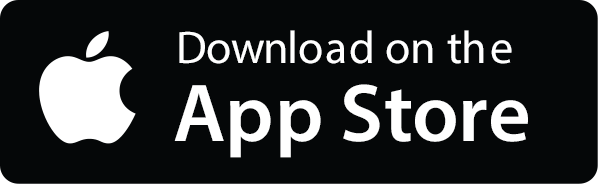
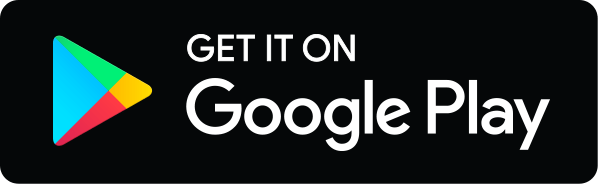
