Abstract
There is robust information that confirms the enormous contribution of inflammation to plaque development, progression and vulnerability. The presence of plaques with inflammatory components associates with a greater likelihood of future cardiovascular events. The inflammatory cascade has been implicated during the entire plaque formation, from the early stages of endothelial dysfunction to the development of acute coronary syndromes (ACS). The presence of macrophages, T lymphocytes, dendritic cells, and mast cells in atherosclerotic lesions; the detection of HLA class II antigen expression; and the finding of secretion of several cytokines point to the involvement of immune inflammatory mechanisms in the pathogenesis of atherosclerosis. Serum biomarkers reflecting the activity of biological processes involved in plaque growth or destabilization may provide great help in establishing the appropriate clinical management, and therapeutic interventions. Evidence for a role of inflammation in plaque rupture has been demonstrated by localization of inflammation at plaque rupture sites. However, the focus of inflammation may not precisely reside within the coronary vessel itself but rather in the injured myocardium distal to the disrupted plaque. These observations outline the potential benefits of therapies targeting inflammation in the arterial wall and cardiovascular system. Emerging anti-inflammatory approaches to vascular protection have the potential to benefit patients by marked reductions in serum biomarkers of inflammation and reduce vascular events. With ongoing technical advances, percutaneous coronary interventions (PCI) will continue to play a critical role in the evaluation of novel compounds designed to modulate inflammation. The constant refinements in the different therapeutic strategies, the combination of scientific understanding in the adequate utilization of novel inflammatory markers, the new pharmacologic agents, and the new techniques in PCI will ameliorate our therapeutic management in ACS based on medical evidence.
1
Introduction
Strong scientific evidence indicates that inflammatory pathway activation is important in the initiation, maintenance and progression of atherosclerosis. The inflammatory cascade has been implicated during the entire plaque formation, from the early stages of endothelial dysfunction to the development of acute coronary syndromes (ACS). This is in accord with the presence of elevated circulating biomarkers of inflammation, which independently predicts the likelihood of adverse cardiovascular events . Atherosclerosis is the focal expression within the artery of a systemic disease, in which traditional cardiovascular risk factors and immune factors play a key role. In addition to outline the importance of inflammation in atherosclerosis progression, these findings enhance the potential of anti-inflammatory therapeutic approaches to prevent and stabilize atherosclerotic disease.
The therapeutic management of patients with ACS in the last decade has shown a dramatic evolution in the understanding of reperfusion. The constant changes in the methodology of reperfusion invite to integrate the recent findings for a better management in the contemporary clinical practice . Serum biomarkers reflecting the activity of biological processes involved in plaque growth or destabilization may provide great help in establishing the appropriate clinical management, and therapeutic interventions. The medicine based evidence strongly suggests the importance of an inflammatory etiology in the ACS. The traditional coronary risk factors are known to terminate in a common final pathway that develops an inflammatory process within the artery wall . Recent evidence indicates that the first steps in atherosclerosis are inflammatory in nature. The presence of macrophages, T lymphocytes, dendritic cells, and mast cells in atherosclerotic lesions; the detection of HLA class II antigen expression; and the finding of secretion of several cytokines point to the involvement of immune inflammatory mechanisms in the pathogenesis of atherosclerosis.
Evidence for a role of inflammation in plaque rupture has been demonstrated by localization of inflammation at plaque rupture sites. However, the focus of inflammation may not precisely reside within the coronary vessel itself but rather in the injured myocardium distal to the disrupted plaque. These observations outline the potential benefits of therapies targeting inflammation in the arterial wall and cardiovascular system. Emerging anti-inflammatory approaches to vascular protection have the potential to benefit patients by marked reductions in serum biomarkers of inflammation and reduce vascular events. In this context, it will be discussed the different therapeutic strategies, the combination of scientific understanding in the adequate utilization of novel inflammatory markers and the new pharmacologic agents, in order to ameliorate our therapeutic management in ACS based on medical evidence.
2
The influence of inflammation in atheroma progression to vulnerable plaques
Several studies have investigated the physiopathological features of those plaques at high risk of rupture and thrombosis, namely, vulnerable plaques. In order to predict which plaques are at risk of precipitating thrombosis and to understand the mechanisms leading to their formation, much effort has been put into recognizing these vulnerable plaques. The process of inflammation develops plaque vulnerability exerting certain changes within the atheromatous plaque . There is a thinning of the fibrous caps, an enhancement of the influx of lipids, an expansion of the lipid core, and stimulation of neoangiogenesis ( Fig. 1 ). Plaque inflammation can promote necrotic core formation, and, in turn, plaque necrosis can worsen inflammation. Necrotic cores contain dead cells mostly consisting of foamy macrophages and their detritus. Necrotic cores likely contribute to plaque rupture and ACS as they possess inflammatory, proteolytic, and thrombogenic properties . Therefore, a vulnerable plaque is characterized as having a large lipid core, thin fibrous cap, macrophage infiltration, spotty calcification and neovascularization ( Fig. 2 ). Progression of atherosclerotic disease with plaque enlargement results in intraplaque hypoxia, which contributes to local inflammation and triggers local neovascularization . Indeed, the presence of neovessels within atherosclerotic lesions was shown not only to promote plaque growth, but also to contribute to its vulnerability . Knowledge of what causes certain changes and modifications within the plaque tissue as the fibrous cap thinning and plaque rupture is incomplete, and major gaps still remain in our deep understanding of the mechanisms leading to thrombosis of nonruptured plaques with erosion ( Fig. 3 ).


Inflammation of the artery wall is a critical component of atherosclerosis and brings about several pathological changes within the vessel wall such as edema, vasa vasorum dilation and proliferation, and immune cells infiltration. This atherosclerotic plaque formation is a chronic process starting early in life. Luminal narrowing is determined by gradual plaque growth and arterial remodeling. Plaque accumulation can be compensated for by expansive remodeling of the vessel wall, however, failure to enlarge and even constrictive remodeling also frequently occurs . The risk of plaque rupture depends on plaque composition rather than on plaque size. Lesions with a large lipid core and increase macrophage infiltration may have a higher risk for disruption than sclerotic plaques. It is now known that a soft lipid-rich core, a thin cap and inflammation in cap and shoulders of the plaque make it vulnerable for rupture . When plaque ruptures, the cap collagen and the highly thrombogenic lipid core are exposed to the thrombogenic factors of the blood and thrombus develops.
Furthermore, atherosclerotic lesions contain immunoglobulin deposits and complement, strongly suggesting the involvement of complement activation in atherogenesis . Bacterial and viral infections have been implicated as potential initiating factors . Infections are known to increase blood viscosity, cause hypercoagulability, and influence the serum lipid profile. Endotoxin may also contribute to endothelial cell production of free radicals which may oxidize LDL-cholesterol . In this inflammatory status several substances are liberated, namely, cytokines, C-reactive protein, and tissue factors, that facilitate the development of arterial thrombus. Therefore, several inflammatory markers are elevated in ACS. The systemic levels of inflammatory marker in patients with stable angina are somewhat lower than those found in the ACS. A systemic inflammatory response often accompanies ACS, and its presence has been widely recognized as a marker of further coronary events . Accumulating evidence suggests that inflammation within the atherosclerotic plaque contributes to its destabilization and subsequent disruption . Although debatable, the widely held view is that systemic inflammation in unstable angina originates from inflammatory process within the arterial wall after plaque disruption ( Fig. 4 ).
2
The influence of inflammation in atheroma progression to vulnerable plaques
Several studies have investigated the physiopathological features of those plaques at high risk of rupture and thrombosis, namely, vulnerable plaques. In order to predict which plaques are at risk of precipitating thrombosis and to understand the mechanisms leading to their formation, much effort has been put into recognizing these vulnerable plaques. The process of inflammation develops plaque vulnerability exerting certain changes within the atheromatous plaque . There is a thinning of the fibrous caps, an enhancement of the influx of lipids, an expansion of the lipid core, and stimulation of neoangiogenesis ( Fig. 1 ). Plaque inflammation can promote necrotic core formation, and, in turn, plaque necrosis can worsen inflammation. Necrotic cores contain dead cells mostly consisting of foamy macrophages and their detritus. Necrotic cores likely contribute to plaque rupture and ACS as they possess inflammatory, proteolytic, and thrombogenic properties . Therefore, a vulnerable plaque is characterized as having a large lipid core, thin fibrous cap, macrophage infiltration, spotty calcification and neovascularization ( Fig. 2 ). Progression of atherosclerotic disease with plaque enlargement results in intraplaque hypoxia, which contributes to local inflammation and triggers local neovascularization . Indeed, the presence of neovessels within atherosclerotic lesions was shown not only to promote plaque growth, but also to contribute to its vulnerability . Knowledge of what causes certain changes and modifications within the plaque tissue as the fibrous cap thinning and plaque rupture is incomplete, and major gaps still remain in our deep understanding of the mechanisms leading to thrombosis of nonruptured plaques with erosion ( Fig. 3 ).
Inflammation of the artery wall is a critical component of atherosclerosis and brings about several pathological changes within the vessel wall such as edema, vasa vasorum dilation and proliferation, and immune cells infiltration. This atherosclerotic plaque formation is a chronic process starting early in life. Luminal narrowing is determined by gradual plaque growth and arterial remodeling. Plaque accumulation can be compensated for by expansive remodeling of the vessel wall, however, failure to enlarge and even constrictive remodeling also frequently occurs . The risk of plaque rupture depends on plaque composition rather than on plaque size. Lesions with a large lipid core and increase macrophage infiltration may have a higher risk for disruption than sclerotic plaques. It is now known that a soft lipid-rich core, a thin cap and inflammation in cap and shoulders of the plaque make it vulnerable for rupture . When plaque ruptures, the cap collagen and the highly thrombogenic lipid core are exposed to the thrombogenic factors of the blood and thrombus develops.
Furthermore, atherosclerotic lesions contain immunoglobulin deposits and complement, strongly suggesting the involvement of complement activation in atherogenesis . Bacterial and viral infections have been implicated as potential initiating factors . Infections are known to increase blood viscosity, cause hypercoagulability, and influence the serum lipid profile. Endotoxin may also contribute to endothelial cell production of free radicals which may oxidize LDL-cholesterol . In this inflammatory status several substances are liberated, namely, cytokines, C-reactive protein, and tissue factors, that facilitate the development of arterial thrombus. Therefore, several inflammatory markers are elevated in ACS. The systemic levels of inflammatory marker in patients with stable angina are somewhat lower than those found in the ACS. A systemic inflammatory response often accompanies ACS, and its presence has been widely recognized as a marker of further coronary events . Accumulating evidence suggests that inflammation within the atherosclerotic plaque contributes to its destabilization and subsequent disruption . Although debatable, the widely held view is that systemic inflammation in unstable angina originates from inflammatory process within the arterial wall after plaque disruption ( Fig. 4 ).
3
Source of inflammation in acute coronary syndromes
Inflammation of the cap is considered as an important mechanism underlying cap destruction. Evidence for a role of inflammation in plaque rupture has been demonstrated by localization of inflammation at plaque rupture sites . The information for local immunological activation has been provided by the demonstration of activated T lymphocytes and macrophages and extensive expression of human leucocyte antigen class II molecules in the atherosclerotic plaque . However, the focus of inflammation may not precisely reside within the coronary vessel itself but rather in the injured myocardium distal to the disrupted plaque. Therefore, the precise location and stimulus for the inflammatory response in ACS remains to be determined. Microscopic multifocal myocardial infarction associated with embolized platelet microthrombi has been well described in ACS and is believed to be the mechanism for the elevation in troponin T found in these patients . On the other hand, several elevated systemic markers of inflammation were found to predict adverse events in patients with ACS. C-reactive protein, a non-specific marker of inflammation that also has a direct inflammatory activity in atherosclerosis has been associated with adverse cardiovascular outcomes in patients with coronary artery disease.
It has been shown clear evidence between systemic and coronary levels of inflammation associated with vulnerable coronary morphology in the setting of ACS . These authors examined systemic and culprit coronary levels of three inflammatory mediators such as high sensitive C-reactive protein (hs-CRP), interleukin-6 (IL-6), and matrix metalloproteinase-9 (MMP-9) in patients with the early phase of acute myocardial infarction (AMI). The measurements of culprit coronary levels of inflammatory markers were performed in the thrombus retrieved by the rescue percutaneous thrombectomy device. The morphology of the plaque was assessed with intravascular ultrasound. It was found a nearly equivalent amount between systemic and culprit coronary levels of hs-CRP, but significantly higher concentrations of coronary levels of both IL-6 and MMP-9. These findings suggest a systemic production of acute phase CRP at the onset of ACS, and local production of both IL-6 and MMP-9 in culprit coronary lesions. They also found a positive relation of systemic levels of hs-CRP with coronary levels of IL-6 and suppose that systemic elevation of acute phase protein in response to culprit coronary production of inflammatory cytokines such as IL-6 may be the underlying mechanism of the link between systemic and coronary inflammation in the setting of ACS. Although, these inflammatory markers were measured at the early phase of AMI with minimal elevation of serum creatine kinase levels in order to minimize the influence of AMI in both systemic and coronary levels of inflammation, it is not known to what extent and the exact influence that myocardial cell damage might have exerted on the inflammatory markers .
CRP is an extremely sensitive, nonspecific, acute-phase reactant produce in response to most forms of tissue injury, infection, and inflammation, and regulated by cytokines, including IL-6, IL-1 and TNF-alpha . There is substantial evidence that CRP may contribute directly to the pathogenesis of atherothrombosis. CRP is ligand binding protein that binds to the plasma membranes of damaged cells. Aggregated but not soluble native CRP selectively binds LDL and VLDL-cholesterol from whole plasma and could thereby participate in their atherogenic accumulation . Complexed CRP also activates complement and can be proinflammatory.
In 1997 C-reactive protein was linked to future cardiovascular events in otherwise healthy individuals . Since then no alternative biomarker has proved to be a superior surrogate for vascular inflammation in the prediction of global cardiovascular risk. This observation is re-affirmed 15 years later by Kaptoge S et al. from the Danish Research Center of Prevention and Health . They found that basal levels of the pro-inflammatory cytokines IL-6, IL-18, and TNF-a, were all associated with future vascular events in an approximately log-linear manner, as were levels of matrix metalloproteinase-9. However, the magnitude and degree of these effects were smaller than that associated with the inflammatory biomarker, C-reactive protein .
However, there are conflicting reports about the presence of CRP in atheromatous lesions, and claims that CRP affects platelet functions are also controversial . The capacity of CRP to enhance tissue factor production suggests a possible causative link between increased CRP values and coronary events. The stimuli responsible for the generally modest elevations in plasma CRP predictively associated with coronary events are not known. They may arise in the atheromatous lesions themselves and reflect the extent of atherosclerosis and the local inflammation that predisposes to plaque instability, rupture, and occlusive thrombosis. On the other hand, increased CRP production may result from inflammation elsewhere in the body that is somehow proatherogenic and procoagulant. This latter alternative is in accord with the results of Suzuki et al. , since they found no significant differences in systemic or culprit coronary lesion of hs-CRP levels, suggesting rather a systemic production of acute phase CRP at the initiation of the ACS.
The complex interplay between factors intrinsic to the plaque and extrinsic events leading to coronary thrombosis is not yet completely understood. Coronary instability is thought to reflect local disruption of the vulnerable plaque. Postmortem studies in patients dying of AMI have consistently found inflammatory cell infiltration at the site of rupture of the culprit atherosclerotic plaque, thus suggesting that it might play a key role in determining plaque disruption . The demonstration of a higher prevalence of inflammatory cells in patients with ACS confirms the evidence accumulated over the past few years that atherosclerosis is an inflammatory disease . It was reported that there is a significant and transient increase in activated T lymphocytes in the peripheral blood of patients with unstable angina , and Caligiuri et al. found a specific proliferative response to proteins contained at the atherectomy specimens of unstable angina patients but not stable patients, thus suggesting that the antigenic triggers might be located at the site of the culprit lesion. These findings are in accord with those of Suzuki et al., since they found a significantly greater level of IL-6 and MMP-9 in culprit coronary lesion than in systemic levels . Spagnoli et al. suggested that acute MI is associated with activation of T lymphocytes, which in turn, with the release of interferon-gamma and other cytokines results in diffuse activation of various cellular types, including smooth muscle cells and monocytes and macrophages. Several observations support the concept that plaque instability is not merely a local vascular accident but probably reflects more generalized pathophysiologic processes with the potential to destabilized atherosclerotic plaques throughout the coronary tree. Cell activation in atherosclerotic plaques can cause severe detrimental effects through a variety of different mechanisms, including thrombogenicity due to tissue factor expression, matrix degradation caused by enhanced release of matrix metalloproteinases, and vasoconstriction caused by enhanced release of endothelin . The triggers responsible for diffuse cell activation throughout the whole coronary circulation of patients with ACS are likely to be multiple and may have a coronary or even non-coronary location.
In a very interesting and well performed investigation, Cusack et al. demonstrated that there is an intracardiac inflammatory response in unstable angina that appears to be the result of low-grade myocardial necrosis. The ruptured plaque does not appear to contribute to the acute phase response. They performed measurements of inflammatory markers in blood sampled at the aortic root, at the coronary sinus, and distal to the culprit coronary lesion. There was no difference in the levels of tumor necrotic factor-alpha (TNF-alpha) or IL-6 between the proximal and distal coronary artery despite the presence of a transcardiac cytokine gradient between the aortic root and coronary sinus. The rise in the level of both IL-6 and TNF-alpha between the aortic root and coronary sinus in patients with unstable angina suggests an intra-cardiac synthesis of these substances. They found no gradient in cytokine concentrations between the aortic root and the coronary vessel distal to the culprit lesion suggesting that the inflammatory response appears to lie within the downstream myocardium. The relationship they found between intracardiac cytokine synthesis and troponin T elevation further suggests that the inflammatory response is related to necrosis within the myocardium . Interestingly, patients with ACS and elevated levels of IL-6 experiment a further significant level increase post-angioplasty. Percutaneous coronary intervention in ACS patients is known to be associated with distal embolization within the coronary artery of platelet microthrombi and a significant risk of periprocedural AMI . Therefore, this further significant increase of IL-6 after angioplasty might be related to myocardial microinfarction from platelet microaggregate embolization. The elevation of inflammatory markers in this setting would suggest that the inflammatory response is related to necrosis within the myocardium.
4
Molecular imaging of arterial vessel wall inflammation
Molecular imaging modalities have been implemented for non-invasive diagnosis and the investigation of the progression of atherosclerosis . These imaging modalities include the use of magnetic resonance imaging (MRI) or computed tomography (CT) for visualizing anatomical structures, positron emission tomography (PET) for the observation of molecular and cellular activities, and PET associated with radio-tracers such as 18F-fluorodeoxyglucose (FDG) . The combined utilization of these imaging modalities, namely, the co-registration of anatomical and functional images from combined PET/CT is a plausible and reliable method for evaluating the progression of atherosclerosis and inflammation in vivo . PET/CT has been used for detection, location, and quantification of atherosclerotic plaques within the carotids, aorta, and coronary arteries . In vivo detection of inflammation within affected blood vessels provides a reliable tool to assess disease activity, leading to better clinical management of the patient. It gives us useful information of ongoing pathology, quantifying residing inflammation and predicting future outcome . Greater knowledge and better understanding of the molecular and cellular events paves the way to identify therapeutic targets that would regulate the course of inflammation and improve outcome before irreversible damage ensues.
Molecular imaging allows visualizing complex pathologies as various inflammatory events that underlie or precede tissue morphological changes can be imaged in a serial and quantitative fashion. Oxidative stress and inflammatory cytokines generated from inflammatory cells, especially monocytes and macrophages, contribute to cellular infiltration, atherosclerotic plaque expansion, thrombosis and adverse cardiovascular events leading to ACS, ventricular remodeling and heart failure . Therefore, there is a growing necessity to go beyond the capabilities of conventional imaging techniques; to image not only whole organs but also to probe at the cellular and molecular levels. This procedure would permit envisaging various in vivo biological events that govern tissue structural integrity and functional homeostasis. One of the cells that have been targeted is the macrophage due to its intense metabolism. Macrophage imaging will help to expand this effort to study cellular and molecular events and downstream inflammatory effects for improving our understanding of biological events in cardiovascular diseases . Since macrophages consume glucose at a high rate, radioactively labeled glucose analogs have been designed to detect their presence and the extent of damage within diseased vessels. Even though the macrophages involved in the formation of atherosclerotic plaque and inflammation can use fatty acids as an energy source, their high-energy rate restricts them to anaerobic metabolism for the most part. Consequently, glucose becomes the favorable source of energy for these cells through the glycolytic pathway . The way that 18F-FDG enters the cells is equivalent to the way glucose does through the glucose transporter protein system. Higher accumulation of 18F-FDG results in higher release of PET photons, generating the contrast that is observed in the final PET image. There is a rapidly expanding field for identifying and characterizing distinct macrophage cell phenotypes which orchestrate divergent pro- and anti-inflammatory functions. Macrophage imaging can track disease progression, monitor treatment response, and predict prognosis noninvasively in a variety of inflammatory cardiovascular diseases to improve patient management and outcome.
The goal of molecular imaging is to identify and quantify disease process before it has advanced to the point that available therapeutic interventions do not act effectively anymore. This fact translates into identifying patients with inflamed atherosclerotic plaques about to rupture but before an episode of ACS begins. Molecular imaging techniques targeting activated macrophages, neovascularization, or increased cellular metabolic activity can represent effective means of non-invasive detection of vascular inflammation. These include contrast-enhanced ultra-sonography, which allows detection of neo vessels within the wall of inflamed arteries; contrast-enhanced cardiac MRI that can detect increased thickness of the arterial wall, usually associated with edema, or mural enhancement using T2 and post-contrast T1-weighted sequences, respectively; and PET associated with radio-tracers such as 18F-FDG and the new 11C-PK11195 in combination with computed tomography angiography to detect activated macrophages within the vessel wall . Imaging techniques are useful in the diagnostic work-up of large-and medium-vessel vasculitis, to monitor disease activity, and the response to treatments. In addition, molecular imaging targets can provide new clues about the pathogenesis and evolution of immune-mediated disorders involving arterial vessels.
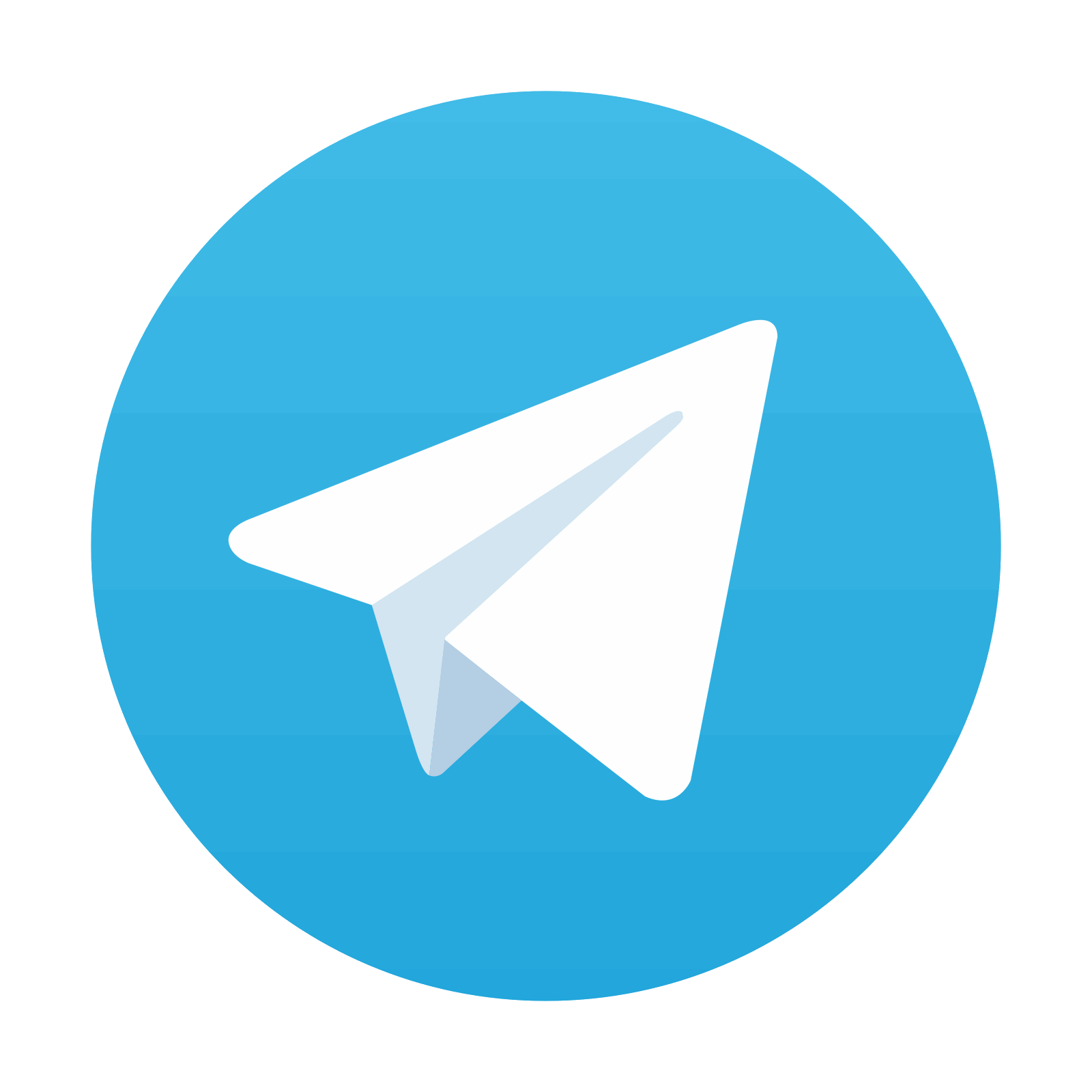
Stay updated, free articles. Join our Telegram channel

Full access? Get Clinical Tree
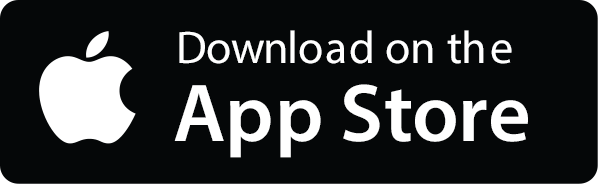
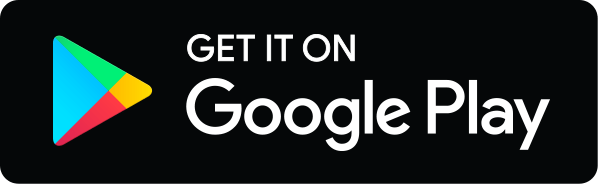