Background
Significant hemodynamic changes, including preload and afterload modifications, occur during the transition from the fetal to the neonatal environment. The ductus arteriosus closes, pulmonary vascular resistance decreases, and pulmonary blood flow increases. Strain rate (SR) and strain (ϵ) have been proposed as ultrasound indices for quantifying regional wall deformation. This study was designed to determine if these indices can detect variations in regional deformation between early and late neonatal periods.
Methods
Data were obtained from 30 healthy neonates (15 male). The initial study was performed at a mean age of 20.1 ± 14 hours (exam 1) and the second at 31.9 ± 2.9 days (exam 2). Apical and parasternal views were used to quantify regional left ventricular (LV) and right ventricular (RV) longitudinal and radial SR and ϵ, and systolic, early, and late diastolic values were calculated from these curves. A paired-samples t test was performed comparing the two groups.
Results
Compared with exam 1, LV radial deformation showed significant reductions in peak systolic ϵ in the basal and mid segments (51 ± 15% vs 46 ± 9%, P < .01). LV longitudinal deformation behaved similarly, showing significant peak systolic ϵ reductions in all measured segments. Systolic SR showed reductions only in the basal and apical segments of the lateral wall and in the mid portion of the inferior wall (−1.9 ± 0.5 vs −1.7 ± 0.3 s −1 and −1.9 ± 0.4 vs −1.7 ± 0.2 s −1 , respectively, P = .03). RV longitudinal free and inferior wall systolic SR and ϵ values were significantly higher in exam 2.
Conclusions
LV peak systolic ϵ decreases in exam 2 were possibly due to afterload increase and preload decrease. The lower RV initial deformation indices could be attributed to increased afterload caused by physiologic pulmonary hypertension or immature RV contractile properties. SR seemed to be a more robust index than ϵ and less influenced by preload and afterload hemodynamic alteration.
One of the most dynamic circulatory changes in human life occurs during the transition from the fetal to the neonatal environment. At this time, the ductus arteriosus closes, and pulmonary blood flow increases as pulmonary vascular resistance decreases. Blood flow returning to the left ventricle relatively increases preload, and systemic arterial blood pressure rises, increasing left ventricular (LV) afterload. Postpartum, right and left stroke volumes become equivalent. Right ventricular (RV) afterload decreases proportional to neonatal pulmonary vascular resistance decrease.
Within 4 weeks of delivery, pulmonary artery pressure decreases, reaching adult levels. As previously demonstrated, the ratios of the RV muscle volume to the body surface area and to the end-diastolic volume decrease during the neonatal period. This finding indicates that volume and pressure overload alterations may change RV muscle.
Myocardial deformation results from a complex interaction of intrinsic contractile forces and extrinsic loading conditions applied to tissues with various elastic properties. Strain rate (SR) and strain (ϵ) have been proposed as ultrasound indices for quantifying regional wall deformation, and reference values for healthy neonates have been recently published. These indices seem to measure heart muscle shape alterations and appear to be true indicators of local contractile function. As some authors have demonstrated in an animal study, preload and afterload as well as myocardial fiber intrinsic tension changes can be important determinants of deformation pattern magnitude. Our aims in the present study were to determine if SR and ϵ ultrasound-based deformation indices could detect variations between early and late neonatal periods and to investigate potential causes for these variations.
Methods
Study Population
From an original population of 55 healthy term neonates, who were the subjects of our earlier study, in the present study we focused on 30 of those healthy subjects who were examined both within 24 hours postpartum and also 1 month later. Follow-up evaluations were performed for the purposes of this study; all neonates remained healthy during the study period. These neonates showed neither abnormalities in clinical examination nor histories of gestation disturbances. Resting electrocardiography showed normal sinus rhythm, and heart rate varied from 90 to 135 beats/min. Fifteen neonates (50.0%) were male, with a mean gestational age of 39.1 ± 1.2 weeks and a mean birth weight of 3174 ± 374 g. The mean 5-minute Apgar score was 9.1 ± 0.4. The results of 2-dimensional standard grayscale and color Doppler examinations were within normal limits. All data were obtained from neonates born at Maternidade Hilda Brandão, Santa Casa de Belo Horizonte (Belo Horizonte, Brazil), and parents gave informed consent. All studies were performed in accordance with the rules and regulations of the hospital ethics committee. The initial examination was performed at a mean age of 20.1 ± 14 hours (exam 1). The second examination was performed on the same neonates at 31.9 ± 2.9 days (exam 2). The mean body weight of neonates at exam 2 was 4,399 ± 601 g.
Color Doppler Myocardial Imaging
All echocardiographic studies were performed using Vivid 7 equipment (GE Vingmed Ultrasound AS, Horten, Norway; 5.0-MHz to 8.0-MHz transducer) during both exams for all neonates. No sedatives were given, and the neonates rested for 10 minutes on examination beds. Conventional LV dimension measurements were obtained from standard M-mode grayscale images. The ejection fraction was calculated using the Teichholz method. Pulsed-wave Doppler velocities were measured from different segments of LV and RV short and longitudinal axes.
Real-time two-dimensional color Doppler myocardial imaging (CDMI) data were recorded from the left and right ventricles using standard parasternal and apical views at 300 ± 50 frames/s. An appropriate velocity scale to prevent CDMI data aliasing was used. The narrowest image sector angle was chosen to obtain the maximum color Doppler frame rate. For parasternal views, the posterior LV wall was kept perpendicular to the ultrasound beam to align at 0° to radial motion. For apical views, each wall was kept central to the ultrasound sector, guaranteeing alignment as near as possible to 0°. During normal respiration, 3 complete consecutive cardiac cycles were recorded and subsequently analyzed.
Offline Analysis
CDMI data were digitally stored and transferred to a computer workstation using dedicated software (EchoPAC version 3.0.0, build 1375; GE Vingmed Ultrasound AS). The software permitted the computation of regional velocities and SR and ϵ values. Only one component of the 3-dimensional velocity vector, aligned to the ultrasound beam direction, was measured. Strain is defined as the deformation of an object normalized to its original shape. SR measures local tissue rate deformation. Both can be mapped along the ultrasound beam for any myocardial segment and can be measured throughout the cardiac cycle.
In radial deformation, a thickening segment produces positive SR and ϵ values, whereas a thinning segment produces negative SR and ϵ values. In longitudinal deformation taken from apical views, the negative SR and ϵ tracing values represent shortening in systole, whereas positive SR and ϵ values represent lengthening in diastole.
Analysis was performed for regional longitudinal peak SR and ϵ using apical views for the basal, mid, and apical segments of the LV septum and lateral, inferior, and anterior walls. Analysis was also performed using the free RV lateral and inferior walls. The latter were obtained from an apical 2-chamber RV view. Only basal segments were interrogated.
The timing of mitral and aortic valve opening and closure was obtained using pulsed-wave Doppler traces of aortic and mitral valve to properly define LV filling onset. Similarly, the timing of pulmonic and tricuspid valve opening and closure was determined by pulsed-wave Doppler when analyzing the right ventricle. These timings allowed precise systolic and diastolic determination. Data were then assigned to individual cardiac cycles (systolic ejection, early and late diastole). The computation area was set at 6 mm to improve signal-to-noise ratio. Data were taken from 3 consecutive beats and subsequently averaged.
Statistical Analysis
Data were analyzed using SPSS version 13.0 (SPSS, Inc, Chicago, IL) and are presented as mean ± 2 SDs. Comparisons were performed among basal, mid, and apical segments in each wall and between the left and right ventricles. Paired wall segments of the same neonates were measured at exam 1 and exam 2. A paired-samples t test was conducted to evaluate the two groups, and a P value < .05 was considered statistically significant. Two independent investigators measured SR and ϵ to detect interobserver variability. Each performed 36 measurements in different wall segments of 10 randomly chosen neonates. For intraobserver variability, one investigator measured 36 segments and remeasured them 4 weeks later. Agreement was verified as suggested by Bland and Altman. These analyses consisted of comparing the differences and the means between paired measurements. The relative bias was calculated as difference divided by mean and is shown as a percentage. In a previous publication, data showed encouraging reproducibility results.
Results
In exam 1, patent foramen ovales were present in 91% of the neonates, and 67.3% had open ducti arteriosi. In all cases, however, the shunts were hemodynamically insignificant. No enlargement of cardiac chambers was detected by conventional echocardiography. In exam 2, foramen ovales were patent in 4 neonates (13.3%), and all ducti arteriosi had closed. LV global cardiac function, measured by ejection fraction, was within normal limits in both examinations (exam 1: 71.4 ± 6.1%; exam 2: 68.6 ± 13.5%). Tricuspid regurgitation was present in 33% of the subjects in exam 1, and mean pulmonary artery systolic pressure was calculated as 30.9 ± 6.4 mm Hg using the simplified Bernoulli equation, assuming right atrial pressure of 5 mm Hg. In exam 2, only 3 neonates had tricuspid regurgitation, and mean pulmonary artery systolic pressure was calculated as 23.6 ± 11.4 mm Hg using the same method. Table 1 shows clinical, demographic, and standard echocardiographic characteristics of the exam 1 and exam 2 study populations.
Variable | Exam 1 | Exam 2 |
---|---|---|
Male/female | 15/15 | 15/15 |
Weight (g) | 3,174 ± 374 | 4,399 ± 601 |
Age at echocardiography | 20.1 ± 14 h | 31.9 ± 2.9 d |
Patent foramen ovale | 28 | 4 |
Patent ductus arteriosus | 20 | 0 |
Heart rate (beats/min) | 110 ± 25 | 115 ± 16 |
Tricuspid regurgitation | 10 | 3 |
Peak velocity, tricuspid regurgitation (cm/s) | 254.7 ± 40 | 215.9 ± 53.3 |
Peak velocity, pulmonary valve (cm/s) | 71.7 ± 9.7 | 85.7 ± 12 |
Aorta (M-mode, mm) | 10.3 ± 0.8 | 12.4 ± 0.8 |
Left atrium (M-mode, mm) | 13 ± 1.6 | 14.1 ± 1.2 |
Left ventricle, systole (M-mode, mm) | 10.9 ± 2.5 | 13.1 ± 2.2 |
Left ventricle, diastole (M-mode, mm) | 18.1 ± 2.2 | 21 ± 2.3 |
Ejection fraction (Teichholz) (%) | 71.4 ± 6.1 | 68.6 ± 13.5 |
Fractional shortening (%) | 38.1 ± 4.8 | 39.3 ± 8.9 |
CDMI data sets were obtained from all neonates measured at early and late neonatal periods. The data were of uniformly good quality, allowing final analysis in 97% of the segments. The remaining 3% of the anterior, lateral, and inferior wall segments were unacceptable and therefore excluded.
Regional LV Radial Function
Data from the same neonate were compared in 2 examinations on different occasions (exams 1 and 2). Table 2 shows that systolic ϵ decreased significantly in exam 2 in the basal and mid segments (51.03 ± 14.86% vs 45.87 ± 8.92%, P < .01). However, no significant differences were detected between SR systolic values between exam 1 and exam 2.
SR (s −1 ) | ϵ (%) | ||||||
---|---|---|---|---|---|---|---|
Component | Segment | Exam 1 | Exam 2 | P | Exam 1 | Exam 2 | P |
Systolic | Basal | 2.90 ± 0.87 | 2.94 ± 0.83 | .688 | 51.03 ± 14.86 | 45.87± 8.92 | .009 ∗ |
Mid | 2.73± 0.68 | 2.92 ± 0.69 | .073 | 56.13 ± 13.14 | 51.60 ± 13.69 | .000 ∗ | |
Early diastolic | Basal | −5.72 ± 1.86 | −5.81 ± 1.36 | .828 | |||
Mid | −6.30 ± 2.28 | −7.18 ± 2.13 | .123 | ||||
Late diastolic | Basal | −3.77± 1.81 | −4.41 ± 1.86 | .177 | |||
Mid | −3.70 ± 1.58 | −4.86 ± 1.78 | .012 ∗ |
Regional LV Longitudinal Function
When comparing data obtained at the basal septum in the apical LV 4-chamber view in both exams, a significant systolic ϵ reduction appeared in all segments in the second exam. Systolic SR showed no significant difference between the two exams ( Table 3 , Figure 1 ).
SR (s −1 ) | ϵ (%) | ||||||
---|---|---|---|---|---|---|---|
Component | Segment | Exam 1 | Exam 2 | P | Exam 1 | Exam 2 | P |
Systolic | Basal | −2.02 ± 0.77 | −1.84 ± 0.33 | .113 | −26.42 ± 5.56 | −23.63 ± 3.65 | .000 ∗ |
Middle | −1.90 ± 0.58 | −1.85 ± 0.29 | .498 | −25.58 ± 3.39 | −23.10 ± 2.55 | .000 ∗ | |
Apical | −1.62 ± 0.26 | −1.65 ± 0.24 | .471 | −24.94 ± 3.38 | −22.97 ± 2.03 | .001 ∗ | |
Early diastolic | Basal | 3.32 ± 1.92 | 3.11 ± 1.23 | .614 | |||
Middle | 2.97 ± 1.57 | 3.29 ± 1.17 | .335 | ||||
Apical | 3.33 ± 1.56 | 4.21 ± 1.31 | .021 ∗ | ||||
Late diastolic | Basal | 2.40 ± 0.96 | 2.84 ± 1.73 | .167 | |||
Middle | 2.16 ± 0.96 | 2.62 ± 1.39 | .142 | ||||
Apical | 2.51 ± 1.48 | 3.03 ± 1.62 | .191 |

Regional RV Longitudinal Function
In exam 2, a significant increase was noticed in systolic SR and ϵ in the basal, mid, and apical segments of the RV free lateral wall. Additionally, systolic SR and ϵ showed similar patterns in the RV inferior wall basal segment ( Table 4 , Figure 2 ).
SR (s −1 ) | ϵ (%) | ||||||
---|---|---|---|---|---|---|---|
Component | Segment | Exam 1 | Exam 2 | P | Exam 1 | Exam 2 | P |
Apical 4-chamber free lateral wall | |||||||
Systolic | Basal | −2.00 ± 0.64 | −2.25 ± 0.42 | .013 ∗ | −28.45 ± 5.23 | −37.03 ± 5.42 | .000 ∗ |
Middle | −1.95 ± 0.59 | −2.25 ± 0.59 | .002 ∗ | −33.21 ± 6.68 | −42.56 ± 4.76 | .000 ∗ | |
Apical | −2.23 ± 0.61 | −2.25 ± 0.60 | .878 | −33.33 ± 4.83 | −41.08 ± 5.51 | .000 ∗ | |
Early diastolic | Basal | 2.76 ± 0.72 | 4.28 ± 1.21 | .000 ∗ | |||
Middle | 3.07 ± 1.13 | 5.03 ± 1.58 | .000 ∗ | ||||
Apical | 3.99 ± 1.53 | 4.61 ± 1.80 | .115 | ||||
Late diastolic | Basal | 1.74 ± 0.60 | 3.06 ± 1.12 | .000 ∗ | |||
Middle | 2.54 ± 0.98 | 3.94 ± 1.43 | .000 ∗ | ||||
Apical | 3.72 ± 1.50 | 3.40 ± 1.80 | .368 | ||||
Apical 2-chamber inferior wall | |||||||
Systolic | Basal | −1.83 ± 0.50 | −2.05 ± 0.47 | .022 ∗ | −27.74 ± 4.29 | −35.43 ± 5.23 | .000 ∗ |
Early diastolic | Basal | 2.96 ± 1.35 | 3.78 ± 1.22 | .020 ∗ | |||
Late diastolic | Basal | 2.27 ± 0.91 | 2.85 ± 1.44 | .090 |
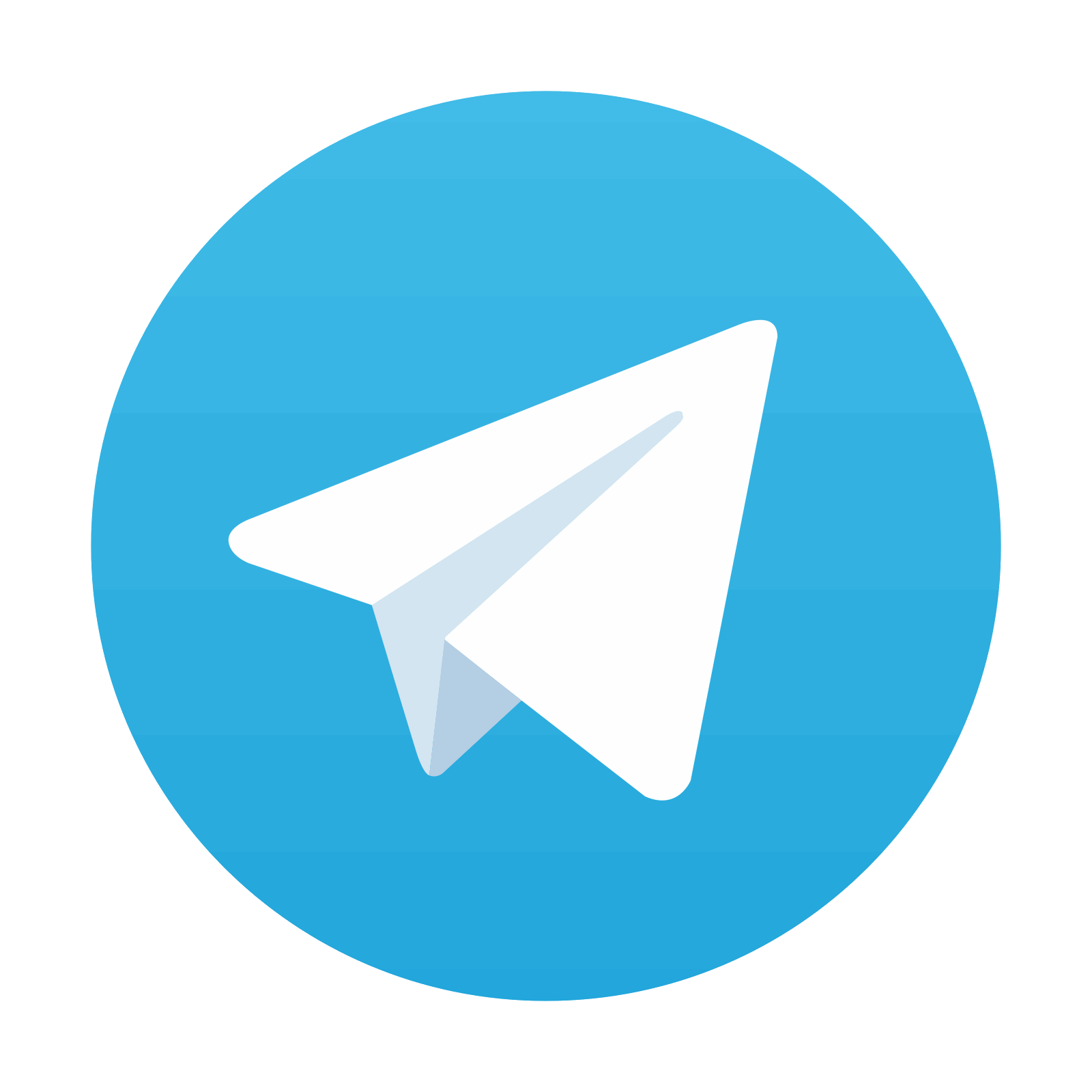
Stay updated, free articles. Join our Telegram channel

Full access? Get Clinical Tree
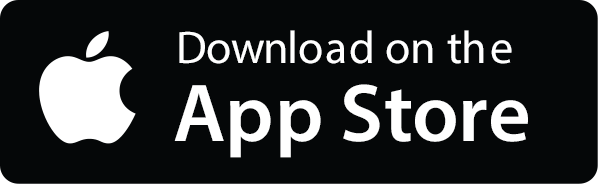
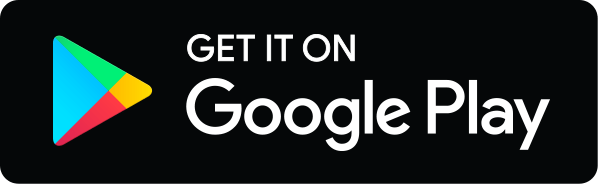
