1. Facilitation of procedures or diagnostic studies
(a) Endotracheal intubation
(b) Central line placement
(c) Radiological imaging (MRI, CT scanning)
2. Immobilization during interhospital or intrahospital transport
3. Intensive care indications
(a) To facilitate mechanical ventilation (especially high-frequency techniques)
(b) Control increased intracranial pressure
(c) Eliminate shivering (therapeutic hypothermia)
(d) Decrease peripheral oxygen utilization
(e) Control severe agitation unresponsive to adequate sedation
(f) Maintain immobilization after surgical procedures
(g) Decrease the risk of pulmonary vasospasm in patients with pulmonary hypertension
(h) Management of tetanus
To maintain the balance benefits between risk and benefit, NMBAs should be used only when absolutely indicated and with appropriate knowledge of and training in their pharmacology, metabolism, and adverse-effect profile. Use of the term “muscle relaxant” should be avoided. Rather, these agents should be thought of as NMBAs, thereby identifying their mechanism of action in their name. With interruption or blockade of skeletal muscle function, these agents cause cessation of respiratory function, mandating the need to provide effective airway control and mechanical ventilation. These agents should not be administered if there is any question as to the normalcy of the airway or the ability to successfully accomplish bag-mask ventilation and endotracheal intubation [31]. The inability to manage the airway including the provision of bag-mask ventilation and/or endotracheal intubation will result in hypoxia and death. These agents provide no amnestic, analgesic, or sedative properties, and should not be used without the coadministration of an amnestic agent (i.e., benzodiazepine, propofol, ketamine, inhalational anesthetic agent). Depth of neuromuscular blockade can be objectively measured using train of four (TOF) monitoring.
Classification of nondepolarizing neuromuscular-blocking agents
Aminosteroid compounds |
Pancuronium |
Rocuronium |
Vecuronium |
Pipecuronium |
Rapacuronium (no longer available) |
Benzylisoquinolinium compounds |
Mivacurium |
Atracurium |
Cisatracurium |
Doxacurium |
Vecuronium is an aminosteroid NMBA that was released for clinical use in the 1980s. In doses of 0.1–0.15 mg/kg, complete neuromuscular blockade with conditions adequate for endotracheal intubation is provided in 90 seconds with a clinical duration of action of 30–40 minutes (intermediate-acting agent) [32]. The onset of complete neuromuscular blockade can be achieved more rapidly by increasing the dose to 0.3 mg/kg. With the larger dose, the onset time is approximately 60–75 seconds, but the duration of neuromuscular blockade is prolonged to 60–90 minutes. Even at these higher doses, vecuronium is devoid of cardiovascular effects. Metabolism is both hepatic (70–80%) and renal (20–30%), so the duration of action is prolonged with hepatic or renal insufficiency.
Rocuronium is a desacetoxy analog of vecuronium. It remains one of the newer aminosteroid NMBAs, having been released for clinical use in the early 1990s. Following a dose of 0.6 mg/kg, the duration of action is 20–40 minutes. Larger doses (1–1.2 mg/kg) are frequently used during rapid sequence intubation as the onset time with these doses has been shown to approximately parallel those of succinylcholine. Clinical studies have demonstrated acceptable conditions for endotracheal intubation in the majority of older children and adolescents within 60 seconds following a dose of 1.0 mg/kg [33–35]. Given its rapid onset of action, rocuronium has been accepted as the agent of choice for endotracheal intubation and RSI by many practitioners. As with other agents, the duration of action increases when larger doses are administered so that 60–90 minutes of neuromuscular blockade occurs following a dose of 1.0 mg/kg. A mild vagolytic effect, less in intensity than that seen with pancuronium, may increase heart rate in the range of 10–20 beats per minute and mean arterial pressure following bolus dosing [36]. Rocuronium undergoes primarily hepatic metabolism, so clinical effects last longer in children with hepatic insufficiency and in infants with immature hepatic microsomal enzymes [37]. There is also a mild prolongation of the clinical effect when rocuronium is administered to patients with renal failure [38, 39].
Cisatracurium is a stereoisomer of atracurium, which itself is uncommonly used because it causes histamine release [40]. Both agents are nondepolarizing NMBA of the benzylisoquinolinium class. Acceptable conditions for endotracheal intubation are provided in approximately 2 minutes with cisatracurium [41]. Hemodynamic effects are minimal. Metabolism of cisatracurium occurs via Hofmann degradation, a pH- and temperature-dependent chemical process, to form laudanosine and the monoquaternary acrylate metabolite. The metabolic pathway is not affected by renal or hepatic function, thereby providing stable pharmacokinetics even in the setting of multisystem organ failure. Laudanosine crosses the blood-brain barrier and animal data have shown that it may cause CNS excitement and seizure activity [42]. The risk of post-ICU weakness is also less with cisatracurium than with aminosteroid agents [43].
Reversal of Neuromuscular Blockade
In the PICU setting, when there is no longer a need for neuromuscular blockade, the agent is discontinued and spontaneous recovery allowed. In certain circumstances, a clinician may desire to reverse the effects of nondepolarizing NMBAs, as is more commonly done in the operating room, with a medication that inhibits acetylcholinesterase. For these medications to effectively reverse neuromuscular blockade, some degree of residual neuromuscular function must be present such as 1–2 twitches on the TOF. Inhibition of acetylcholinesterase results in the accumulation of more acetylcholine to compete with the NMBA at the nicotinic receptor of the neuromuscular junction. The commonly used acetylcholinesterase inhibitors or “reversal agents” include neostigmine, pyridostigmine, and edrophonium. Adverse effects related to the use of reversal agents generally relate to their inhibition of acetylcholinesterase at sites away from the neuromuscular junction. These agents should always be preceded by an anticholinergic agent such as atropine or glycopyrrolate, since the inhibition of acetylcholinesterase at muscarinic receptors leads to symptoms like bradycardia and even asystole. Other adverse effects related to the reversal agents included augmentation of cholinergic function in the gastrointestinal tract (salivation, diarrhea, nausea, and vomiting) and the respiratory tract (bronchospasm). A newer agent, sugammadex, does not inhibit acetylcholinesterase, but rather encapsulates aminosteroid NMBAs (e.g., rocuronium and vecuronium), removing these agents from the plasma and thereby reversing neuromuscular blockade [44, 45].
Adverse Effects of Neuromuscular Blockade
The most devastating adverse effect is the inability to provide adequate oxygenation and ventilation. Therefore, these medications should never be used if there is any suspicion that the airway cannot be controlled. NMBAs will also eliminate routine physiologic functions such as blinking and routine self-repositioning to avoid pressure points. Eye care with the application of artificial teas at fixed intervals is necessary to avoid drying of or damage to the cornea [46, 47]. Adjunctive care includes repositioning of the patient at fixed intervals, early involvement of physical/occupational therapy, use of special mattresses, deep vein thrombosis prophylaxis, and placement of splints to avoid contractures. Ineffective coughing and clearance of secretions mandates the implication of suctioning protocols to limit the risk of nosocomial pneumonias and worsened lung mechanics [48].
Perhaps of greatest concern during the administration of NMBAs is the potential for long-term sequelae with muscle atrophy and weakness. These issues generally resolve spontaneously over time with no longer-term impairment of neuromuscular function. Of more concern, is what is now termed the acute quadriplegic myopathy syndrome (AQMS) [49]. Clinical signs and symptoms include weakness or even flaccid paralysis, relative preservation of extraocular movements, decreased deep tendon reflexes, respiratory insufficiency, intact sensory function, and normal findings in the cerebrospinal fluid. Recovery may be prolonged, requiring weeks to months, with the need for prolonged rehabilitation care, and tracheostomy with chronic ventilatory support. AQMS is associated with the coadministration of NMBAs and corticosteroids, thereby suggesting a heightened awareness in such patients [50, 51]. Critical illness polyneuropathy may be confused with AQMS. It is a combined motor and sensory neuropathy that results from ischemia of the microvasculature of the nerves, which is seen most commonly in patients with multisystem organ failure. The EMG demonstrates a pattern different from that seen in AQMS.
Neuromuscular Blockade and Acute Lung Injury
Despite the potential for adverse effects, recent work has demonstrated the potential for improved outcomes including a survival benefit when NMBAs are administered early in the course of adult patients with ARDS. Three multicenter randomized trials of cisatracurium for neuromuscular blockade in patients with ARDS [52–54] demonstrated a clinical advantage of early neuromuscular blockade manifested as improved oxygenation. A subsequent pooled analysis demonstrated reduced mortality at 28 days and at hospital discharge and a decreased incidence barotrauma [55]. A fourth study from the Chinese literature demonstrated a similar reduction in mortality in adults with ARDS with the use of a vecuronium infusion [56] Despite these findings, the mechanisms remain speculative perhaps relating to promotion of patient-ventilator synchrony, decreased barotrauma/volutrauma, and reduced shearing injury to the lung with reduction of the generation of inflammatory mediators. Based on these findings, the recent Society for Critical Care Medicine guidelines for the use of neuromuscular-blocking agents in adults suggest that “an NMBA be administered by continuous intravenous infusion early in the course of ARDS for patients with a PaO2/FiO2 less than 150.” This was listed as a weak recommendation with moderate quality of evidence. The PALICC guidelines recommend that “if sedation alone is inadequate to achieve effective mechanical ventilation, neuromuscular blockade should be considered” with a daily NMBA holiday.
Iatrogenic Withdrawal Syndrome
PICU patients may develop tolerance, increased dosing required for a consistent clinical effect, to all the commonly used sedatives and analgesics. Tolerance can be due to receptor desensitization or upregulation of the postreceptor pathways, and often results in a physiological dependency state. When the offending medication is stopped, the signs and symptoms of the iatrogenic withdrawal syndrome (IWS) can be present, including agitation, grimacing, abnormal movements, diarrhea, tachypnea, tachycardia, fever, sweating, and hypertension [57]. Risk factors for IWS include longer duration of therapy and higher dosages [58]. Infusions for more than 5 days or doses of >5 mcg/kg/hour fentanyl appear to be risk factors [59]. As children with ARDS often undergo a prolonged period of ventilation and sedation, the risk for IWS is high. IWS has been shown to delay patient recovery and prolong hospitalization [58].
To facilitate appropriate management, the clinician requires a withdrawal assessment tool to determine the presence and treatment efficacy of the IWS. The Withdrawal Assessment Tool-1 (WAT-1) was developed and validated in a PICU population based upon a population of children who received mechanical ventilation for >5 days [60]. The score is quick and easy to perform, and is often performed once a shift. A WAT-1 score of 3 or greater (maximum 12) may be considered to reflect IWS. The score has features that reflect opiate withdrawal (sweating, yawning, loose stools) as well as benzodiazepine withdrawal (agitation, tremor, uncoordinated movements). The Sophia Observational Score (SOS) has also been validated for use in the PICU [61]. It has many similarities to the WAT-1, but includes more signs of high sympathetic tone and benzodiazepine withdrawal. The intra- and interrater analyses of both scores are good and both scores appear to be useful tools.
The simplest way to prevent IWS is to slowly taper the drug over a period of days to weeks depending on the duration of the infusion. For opiates, this is usually managed by replacing the infusion with an equivalent methadone dose started initially IV, then converting to oral dosing and weaning. The duration of weaning using methadone is usually between 5 and10 days unless very high doses or prolonged infusions are used [62]. Methadone has a high oral bioavailability (80%), a long half-life (24 hours), and has less sedating effect than morphine or fentanyl. Using about three times the total fentanyl daily dose (mcg) divided in three doses per day has been shown to be effective [63]. Dexmedetomidine can also control the signs and symptoms of opiate withdrawal in PICU patients [64]. When discontinuing midazolam, the conversion to a long-acting and effective oral benzodiazepine would be attractive as with using methadone for fentanyl withdrawal. Methadone conversion rates have been well studied; however, the conversion from midazolam to long-acting benzodiazepines has been studied much less so. The total daily midazolam dose (mg) divided by 12 has been used to convert to oral lorazepam, which has a high oral bioavailability (90%) [65], which can then be weaned off.
The incidence of IWS has been reported at 35% for dexmedetomidine infusions greater than 48 hours in the PICU [66]. The more frequent signs and symptoms were agitation, fever, loose stools, vomiting and sleep disturbance, as well as tachycardia and hypertension [67]. These symptoms appear to reflect sympathetic hyperactivity, akin to those experienced during opiate withdrawal. The use of clonidine to treat dexmedetomidine withdrawal has been reported in several small series [66, 67].
Delirium in the PICU
Delirium is characterized by acute and fluctuating disturbances of consciousness and cognition, with inattention and impaired information processing and memory [68]. Children with delirium can have hypoactive, hyperactive, or mixed symptoms. The reported incidence of delirium in the PICU is 25–40% [69, 70]. Risk factors include younger age, developmental delay, and benzodiazepine usage [71, 72]. Recognizing delirium in the PICU is often best aided by the use of scoring systems as the signs and symptoms are multiple and nonspecific for delirium. The pediatric Confusion Assessment Method for Intensive Care Unit (pCAM-ICU) [73] and Pediatric Confusion Assessment Method [70] are two validated tools to assess delirium in the PICU.
Rather than treating delirium, it would be better if we could avoid, or at least minimize, the problem. Adoption of a benzodiazepine-sparing approach may reduce delirium. Dexmedetomidine in adults has been shown to reduce the incidence of ICU-related delirium [74], but the evidence supporting this in children is not as robust. Early mobilization has been reported as a strategy to reduce the incidence of delirium in a PICU [75]. Pharmacologic treatment options for delirium in the ICU include antipsychotic agents such as haloperidol [76]. However, the newer atypical antipsychotics have generally replaced haloperidol due to fewer side effects. Olanzapine and respiridone have been reported as effective in the PICU setting [77], and both are available as a tablet or an orally disintegrating tablet. Olanzapine tends to be more sedating, which may be an added benefit. Results of randomized trials of treatment of ICU delirium in adults with antipsychotic agents have been generally disappointing [78].
Immunomodulatory Effects of Sedation
It has been well documented in both in vitro and in vivo studies that sedation agents have immunomodulating properties [79]. This has been mostly demonstrated in the case of sepsis; however, ARDS and sepsis share significant immune activation pathways and there may be a significant immune effect of sedation in the PARDS setting as well. PARDS patients are frequently receiving other immune-modulating therapies and how these all interact is unknown. As such, the predicted beneficial or detrimental effect from a sedative agent alone may be unclear. Propofol has been shown to have anti-inflammatory effects possibly related to its antioxidant properties [80]. Its immune-suppressive effects stem in part from inhibition of macrophage and neutrophil function. Benzodiazepines exhibit a similar immune-suppressant profile [81]. However, a short-term, low-dose infusion of midazolam in burn patients was found to enhance the immune response and tissue-protective/tissue-repair mediators [82]. Different opioids show different effects on the immune system with both immunosuppressive and immune-stimulatory effects being reported [83]. This may reflect a direct effect on immune cells, and interaction with the hypothalamic-pituitary-adrenal axis, glucocorticoid effect, or modulation of sympathetic activity and catecholamine release. The interaction within these sites is complex and varies between agents as well as between species. Morphine demonstrates anti-inflammatory effects in vitro and increases mortality in vivo in animal models of infection. The effect on lymphocyte function may be associated with late secondary infections. Opioid administration may therefore contribute to the immunosuppression observed in the critically ill. Ketamine [84] has also demonstrated a decrease in cytokine production in experimental septic shock. Dexmedetomidine also appears to have anti-inflammatory effects [85].
Sedation Assessment
As part of the sedation management in the PICU, the depth of sedation should be evaluated on a regular basis. There are many PICU-validated scoring systems available, which can be used by the bedside caregiver to adjust the sedation dosing to the needs of the child. The use of a sedation-scoring system [86] has been shown to reduce the incidence of oversedation- as well as undersedation-related complications. However, the use of nurse-driven protocol did not reduce the number of ventilator days in the RESTORE study [87]. Children in the protocol group had more days when they were awake or only lightly sedated; however, they also had more days when they experienced high pain scores or episodes of agitation, possibly reflecting undersedation.
The Richmond Agitation-Sedation Scale (RASS) [88] and State Behavioral Scale (SBS) [89] are two commonly used tools. Older children may be calm and comfortable with either mild (RASS-1 or − 2) or moderate sedation (RASS-3), but younger children do not understand the need for the uncomfortable “lines and tubes” and deeper sedation may be required. The bispectral index (BIS) monitor may remove the subjectivity from sedation assessment. The BIS monitor measures the level of hypnosis using analysis of the EEG waveform components referenced to a proprietary waveform database, and it has been validated in the PICU [90]. It does not reflect the drug concentration of any particular drug, but the overall sedative effect. The BIS score can be helpful in paralyzed patients [91] as most of the scoring systems are unable to be performed due to a lack of movement response to stimulus. Nurses have been shown to significantly overestimate a paralyzed child’s sedation [91].
Serially assessing sedation with these tools may reduce the overall exposure to these drugs. Another potential strategy to reduce sedation is the use of sedation interruption. Although there are limited studies evaluating this strategy in children, one demonstrated no benefit with respect to ventilator days or dosing of sedation [92]. The cycling of sedation has also been evaluated; however, switching between different sedatives has of yet not be shown to reduce sedation-related complications [92]. The pattern of sedation weaning may also affect the child’s care and outcome. It appears that a continuous wean rather than an interrupted wean was associated with a quicker wean with less withdrawal [93]. This may be more related to the dosing and sedation needs rather than actual sedation weaning. Weaning of opiates or benzodiazepines may be more likely to be successful if the sedatives are weaned using appropriate doses of replacement drugs such as methadone [91]. If the wrong dose is used, then oversedation may occur and delay the weaning process, but underreplacement will result in increased withdrawal symptoms.
Apoptosis and Sedation in the PICU
There is evidence from many rodent and primate studies [94] that suggests most of the general anesthetic agents and sedatives, which are either N-methyl-d-aspartate receptor antagonists or GABAA agonists, can trigger neuronal apoptosis [95]. In 2017, the FDA issued a warning that the following agents could negatively affect brain development in children under the age of 3: fluorinated inhalational agents, midazolam, lorazepam, propofol, etomidate, and ketamine. While retrospective studies suggested that anesthesia early in life was associated with unfavorable neurocognitive outcomes, more recent prospective studies which compared general anesthesia to regional anesthesia for elective hernia repair in infancy did not demonstrate any delirious effect from the anesthesia agents at 2 years [96] and 5 years post anesthesia [97]. However, it is still unknown if exposure to GABA agonist or NMDA antagonists for prolonged periods lasting days to weeks, as is often needed in PARDS, influences neurocognitive outcomes [98].
Conclusion
Sedation for ventilated children with ARDS is required for patient’s comfort, amnesia, safety, and ventilator synchrony. Multiple agents are available, each with unique strengths and weaknesses. Though no specific strategy has been proven to improve outcomes in children with ARDS, it is recommended to use serial objective assessments to provide the lowest possible dose needed to achieve individualized goals for that patient. Considering these risks of delirium, IWS, and altered neuronal development, a combination of opiates and dexmedetomidine may appear to be the best choice. Extrapolating from adult data, use of an NMBA early in the course of severe PARDS may improve outcomes. Cisatracurium offers the advantage of no dependence on end-organ-dependent elimination and may be a more appropriate choice in patients with hepatic or renal failure, since such problems do not alter dosing requirements of this agent. Specific protocols should be in place to ensure appropriate care of the patient who is receiving neuromuscular blockade with attention toward the provision of adequate sedation and analgesia, eye care, prevention of pressure sores, and pulmonary toilet. Overall, more studies are needed to identify the optimal way to provide sedation, analgesia, and neuromuscular blockade in children with ARDS.

Full access? Get Clinical Tree
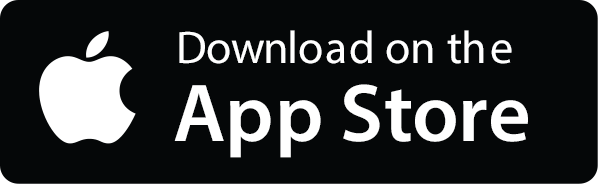
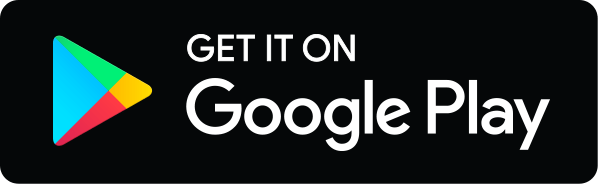