Introduction
“Screening” in the field of pediatric cardiology is currently implemented in many different areas. The interest and opportunities for screening have increased and evolved along with the increase in our knowledge base. Screening currently includes in utero screening for congenital heart disease (CHD), pulse oximetry after birth screening for CHD, screening for at-risk entities for sudden cardiac death, screening for neurodevelopmental issues, screening for familial forms of CHD, and screening for other familial, genetic, and preventive issues such as the hyperlipidemias, as well as environmental issues such as hypertension and metabolic disorders. This chapter reviews these complex issues and suggests potential future endeavors in these areas.
Fetal Echocardiographic Screening (see also Chapters 7 and 8 )
Malformations of the cardiovascular system are some of the most common birth defects. The incidence of CHD in the live-born population is estimated at slightly less than 1%. However, the incidence of CHD in the fetus may be significantly higher, as cardiac defects are estimated to be much more frequent in stillborn infants.
Detecting a cardiac defect, if present, in the fetus is dependent on the timing of the examination, the training and experience of the ultrasonographer and interpreting physician, and the equipment use. At minimum, two-dimensional, color and spectral Doppler should be used. As the echocardiographic appearance of some congenital heart defects can change throughout gestation, one exam at one time point may not be sufficient.
Accurate and sensitive fetal diagnosis is important as the prenatal diagnosis of CHD can affect not only the family’s desire to continue with the pregnancy but can allow for planning delivery at or near a cardiac surgical center, psychological preparation of the family prior to delivery, as well as surgical planning. Over recent years, criteria for fetal echocardiographic screening of fetuses at higher risk of CHD have been established ( Table 89.1 ). For these higher-risk pregnancies, a thorough fetal echocardiogram should be performed in the second trimester.
Indication for Fetal Echocardiography | Risk of Congenital Heart Disease (%) |
---|---|
MATERNAL/FAMILIAL INDICATIONS | |
Maternal pregestational diabetes mellitus (diabetes mellitus diagnosed in the first trimester) | 3%–5% |
Maternal phenylketonuria | 12%–14% |
Maternal autoantibodies (SSA/SSB+) | 1%–5% (increased to 11%–19% if prior child affected with congenital heart block or neonatal lupus) |
Maternal medications | |
Angiotensin-converting enzyme inhibitors | 2.9% |
Retinoic acid | 8%–20% |
Nonsteroidal antiinflammatory drugs | 1%–2% structural CHD, 5%–50% ductal constriction |
Anticonvulsants | 1.8% |
Lithium | <2% |
Vitamin A | 1.8% |
Paroxetine | 1%–2% |
Maternal rubella infection | 1%–2% |
Maternal infection with suspicion of fetal myocarditis | 1%–2% |
Assisted reproduction technology | 1.1%–3.3% |
CHD in first-degree relative of fetus | 2%–18% |
First- or second-degree relative with disorder with mendelian inheritance with CHD association | ≤50% |
CHD in second-degree relative of fetus (less risk, may be indicated) | <2% |
FETAL INDICATIONS | |
Cardiac abnormality suspected on obstetrical ultrasound | >40% |
Extracardiac abnormality suspected on obstetrical ultrasound | 20%–45% |
Fetal karyotype abnormality | Varies. May be as high as 90% |
Fetal tachycardia or bradycardia | Tachycardia 1% for structural CHD, 50%–55% bradycardia |
Frequent or persistent irregular heart rhythm | 0.3%–2% |
Fetal increased nuchal translucency (≥3 mm) | 3%–60% (risk increased with increased thickness) |
Monochorionic twinning | 2%–10% |
Fetal hydrops or effusions | 15%–25% |
However, approximately 80% of children with CHD are born to mothers who have no risk factors for CHD in their offspring and are therefore low risk. While ideal prenatal ultrasound can provide detection rates as high as 85%, the population detection rate of CHD is 30% to 50% in most developed countries. Barriers to diagnosis include later initiation of prenatal care, maternal residence in an area with a high incidence of poverty, and a higher number of previous pregnancies. Cost-effective analysis simulations have identified that the most cost-effective strategy for prenatal screening of CHD is a four-chamber and outflow tract view in the second trimester with direct referral to a pediatric cardiologist if abnormalities are found. To maximize the prenatal diagnosis of CHD, the American Institute of Ultrasound Medicine has recommended that anatomic screening for all pregnancies include a four-chamber view and outflow tract views (American Institute of Ultrasound in Medicine practice parameter). The addition of outflow tract views to the four-chamber view has an estimated sensitivity of 67% with a specificity of 99%.
Recently, research has been evaluating whether screening for congenital heart defects is possible in the first trimester. First-trimester screening has been shown to reliably visualize single ventricle lesions as well as transposition of the great arteries. Some studies have suggested that the vast majority of major CHDs can be detected during a first-trimester ultrasound if performed by an experienced fetal sonographer and obstetrician as early as 11 to 13 weeks’ gestation. Analysis of first-trimester screening shows that cardiac abnormalities identified in the first trimester were more commonly associated with chromosomal abnormalities (50% to 73%) compared to 17% to 21% in second-trimester screening. Some authors suggest that early prenatal diagnosis may alter the natural outcome of pregnancies with CHD as it may increase early termination of first-trimester pregnancies. However, caution should be exercised when counseling families in the first trimester as it is not always possible to detect cardiac abnormalities in enough detail to provide accurate estimation of the natural history of the suspected lesion. Furthermore, some cardiac lesions may not be fully diagnosable in early pregnancy, and first-trimester screening should not replace second-trimester screening.
Extracardiac and chromosomal abnormalities are frequently present when CHD is diagnosed prenatally. For that reason, when CHD is diagnosed prenatally, consideration should be given to chromosomal testing and secondary anatomic screening if these have not already been completed ( Table 89.2 ).
Congenital Heart Disease | Chromosomal Anomaly (%) | Extracardiac Anomaly (%) |
---|---|---|
AVSD | 35–47 | 30–50 |
VSD | 37–48 | 30–37 |
ASD | 1–3 | 16 |
TOF | 27 | 25–30 |
DORV | 12–45 | 19–20 |
HLHS | 4–10 | 1 |
Truncus arteriosus | 14–33 | 15–21 |
Transposition of the great arteries | 0–3 | 15–26 |
Coarctation of the aorta | 21–30 | 12–20 |
Tricuspid atresia | 2–9 | 15–34 |
Ebstein anomaly | 0–3 | 6 |
Aortic stenosis | 1–15 | 13 |
Pulmonary stenosis/atresia | 4–5 | 20–26 |
Pulse Oximetry Screening
The calculated prevalence of critical CHD (requiring intervention in the first year of life) varies from study to study, but based on most recent estimates is approximately 2 infants per 1000 live births, with an overall prevalence of any type of CHD of 9 infants per 1000 births. Early diagnosis, particularly of critical CHD, can prevent significant morbidity and mortality. Newborns with critical CHD can have profound and rapid deterioration in clinical status in the first days or weeks of life, usually related to closure of the ductus arteriosus and physiologic changes in pulmonary vascular resistance. Identification of these newborns prior to hospital discharge, and prior to the development of significant hemodynamic compromise, can allow for timely intervention and medical management such as prostaglandin therapy to maintain ductal patency. The relatively high prevalence combined with a period of time where critical CHD can be diagnosed prior to clinical deterioration makes critical CHD an ideal subject for population-based screening.
Early studies evaluating the use of pulse oximetry in neonates demonstrated that lower-extremity oxygen saturation in infants with critical CHD was lower than age-matched control subjects without critical CHD. In determining the most effective screening strategy, sensitivity and specificity should be maximized. False-positive results can be a public health concern as they require subsequent testing, which in some settings may delay hospital discharge of the infant or require transfer to another institution, resulting in added familial anxiety, stress, and cost. However, increasing specificity at the expense of sensitivity will increase the false-negative rate, possibly resulting in a less effective screening regimen overall. The American Heart Association and American Academy of Pediatrics convened a writing group to compose a scientific statement in 2009 to evaluate the evidence on the routine use of pulse oximetry in newborns to detect critical CHD. They analyzed ten studies, including over 123,000 infants total. Overall, the sensitivity of pulse oximetry screening for critical CHD ranged from 0% to 100% with specificity ranging from 95.5% to 100%. False-positive screens were found in a mean of 0.9% of infants with a decrease in the false-positive rate to 0.04% after 24 hours. The writing group suggested that a single lower-extremity pulse oximetry reading at an age greater than 24 hours with a threshold of 95% or greater was the most appropriate large-scale screening strategy. Further study is necessary to determine if the threshold to maximize sensitivity and specificity is different at moderate or greater altitude, as the normal saturation of neonates at altitude is around 95%.
In September 2011, the United States Secretary of Health recommended that pulse-oximetry screening before discharge should be added to the newborn screening panel for the early detection of critical CHD for all infants born in the United States. By 2014, 43 of 50 states had instituted legislation or regulations mandating pulse-oximetry screening with seven states and the District of Columbia supporting pulse-oximetry screening as the standard of care with no legislation in place. There have not been many studies assessing the accuracy of screening since the implementation of universal pulse-oximetry screening. An initial study in the UK in 2011 showed a false-positive rate of 0.8% with a negative predictive value of 99.7%. Of 20,055 newborn infants, 24 had critical CHD. Of these 24, 6 were not identified by pulse-oximetry screening. The critical lesions most likely to be missed by using pulse oximetry as a screening method were obstructive arch lesions such as coarctation and interrupted aortic arch. In recent studies in the United States, pulse oximetry screening has been shown to be cost effective.
Screening and Sudden Cardiac Death
Primary Prevention of Sudden Cardiac Death: Screening With History and Physical Examination, Electrocardiography, and Echocardiography
A fair amount of controversy exists with regard to primary strategies for the prevention of sudden cardiac arrest (SCA) and sudden cardiac death (SCD). The accepted standard had been for a thorough comprehensive and uniform screening history, family history, and physical examination as suggested by the American Heart Association with the documentation of 12 important points. The 12 important points that need to be reviewed are as follows:
Family history
Premature sudden death
Heart disease in surviving relatives
Personal history
Heart murmur
Systemic hypertension
Fatigability
Syncope
Exertional dyspnea
Exertional chest pain
Physical examination
Presence of a heart murmur
Femoral pulses
Stigmata of Marfan syndrome
Blood pressure measurement
This approach underscores the importance of the discovery of signs and symptoms that may ultimately uncover underlying at-risk abnormalities for sudden cardiac arrest. However, the utility and success of this strategy is less than optimal. Specifically, it has been difficult to thoroughly and uniformly achieve the above strategy. Studies have suggested that, overall, the majority of states in the United States have not been able to adopt a strategy for covering the important points recommended by the American Heart Association. As a result, several organizations, including the American Academy of Pediatrics, have suggested a uniform preparticipation form whereby the key questions are covered prior to sports participation. Several points must be emphasized. Firstly, prevention of SCA and SCD cannot be discussed in the context of athletic participation only and cannot be directed only to athletes. An “athlete” may be somewhat difficult to truly define specifically. In addition, in that underlying and undiagnosed cardiac abnormalities, both structural and electrical, have the potential to cause SCA in any at-risk young person, the protection as well as the strategies for prevention should include all youth, not only those arbitrarily defined as athletes. Secondly, the efficacy of the history, family history and physical examination is far from perfect. Though there are some retrospective studies that suggest that as many as 25% to 50% of those who experienced SCD had antecedent symptoms such as syncope, palpitations, or chest pain, the majority of studies have shown a relatively low yield for this strategy. And of course the individual who truly has no symptoms, has a negative family history, and a normal physical examination will not be uncovered by this strategy for discovery and prevention.
In 2006, Corrado published a study outlining the results of 25 years of a mandatory electrocardiography (ECG) screening program for competitive athletes in the Veneto region of Italy. Corrado’s study showed a 90% reduction in the incidence of SCD in the athletic population by prospectively identifying those athletes with arrhythmogenic right ventricular dysplasia (ARVD) and hypertrophic cardiomyopathy (HCM) and subsequently excluding them from athletic participation. However, studies by other investigators were not able to reproduce this experience. Maron did not find similar outcomes in a study reported from Minnesota in 2009 and Steinvil (2011) did not find a reduction in the risk of SCD in athletes in Israel with their mandatory ECG screening program in 2011. However, tremendous controversy has been generated by this work. Concerns include reproducibility of the data, ethical considerations regarding selecting specific populations and autonomy, generalizability to heterogeneous populations, effects of false positives and negatives, and cost considerations. The American Heart Association has not yet embraced and recommended a strategy for mass ECG screening for young athletes in the United States although the European Society of Cardiology (ESC) has indeed made that recommendation. Although multiple grass roots local screening organizations in the United States have undertaken ECG screening endeavors in order to identify at-risk individuals, this strategy has not yet been endorsed by the American Heart Association or other screening organizations in the United States as a mass screening strategy/plan. Ongoing work is continuing in this area. Further refinement of ECG criteria in this setting in order to define true “normals” in a multicultural society in the United States is ongoing in an effort to identify abnormalities and in order to minimize false positives, a variable but important issue in the setting of mass ECG screening. So although mass ECG screening has not been endorsed by all, as described above, there continues to be a refinement of ECG criteria and a collection of screening data by multiple organizations. There are unquestionable examples of this strategy resulting in lives saved. This strategy is still debated and discussed. As these data are collected, debated, and discussed, this approach hopefully will be sorted out with perhaps more definitive data with regard to its efficacy. In the meantime, the logistics and resources needed to adopt an effective strategy on a mass basis will ideally be worked out as we determine the optimal strategy for primary prevention of sudden cardiac arrest and sudden cardiac death.
Echocardiography as a primary screening tool has also been suggested as either an independent approach or in combination with ECG screening. Just as with ECG screening, it is important that the echocardiographic studies be performed by an experienced technician and that the studies are interpreted by an experienced echocardiographer. It is unlikely that a screening echocardiogram will uncover all of the at-risk anatomic abnormalities including and especially congenital coronary artery abnormalities. Certainly, an echocardiogram will not allow for the diagnosis of electrical abnormalities/channelopathies. The resources necessary and the performance and interpretation of screening echocardiograms has not made this strategy one that is easy to carry out and is certainly not currently recommended as a mass screening approach in and of itself.
Finally, and beyond the scope of this chapter, it is important to consider the importance of secondary prevention studies with regard to sudden cardiac death. Although it would be the hope that the above strategies could prevent sudden cardiac arrest in all cases, it is very unlikely that such will be the case. Therefore it is critically important that we advocate for education in cardiopulmonary resuscitation and use automatic external defibrillator (CPR-AED) for as many people as possible, particularly high school students and staff. Such a strategy will allow for a population that will be able to recognize cardiac arrest and intervene by doing CPR and using an AED. Such a strategy has been shown to increase the disappointingly low incidence of lay-rescuer CPR, as well as the very poor outcomes associated with out-of-hospital cardiac arrest.
Screening First-Degree Relatives After Sudden Cardiac Death in the Young
The incidence of sudden death has been reported between 2.7 and 7.5 events per 100,000 patient-years among young people (age 1 to 40 years) who were previously thought to be well. Exact numbers remain unknown, but these rates would result in 10,000 children and young adults experiencing sudden unexplained death in the United States every year. Italy, France, the Netherlands, and international communities have rolled out screening programs and registries. Most of these deaths ultimately will be attributed to cardiac causes. A large, prospective population-based study from Australia and New Zealand diagnosed cardiomyopathy on autopsy in 16% of cases. This correlates closely with a Danish population-based registry with autopsy-based cardiomyopathy in 17% of cases. Arrhythmogenic causes of sudden death are harder to quantify because the hearts tend to be structurally normal or near-normal; a potentially arrhythmogenic cause was present in 45% of cases in the large Australia/New Zealand series. In addition, pathogenic or likely pathogenic variants in genes associated with congenital arrhythmia susceptibility and familial cardiomyopathy have been demonstrated in 3% to 35% of postmortem samples from cases of sudden unexplained death (see Table 89.1 ). Because these diseases may be inherited, screening for affected family members is critical. In work from several centers, approximately 15% of families are found to be affected with a heritable cardiac disease when first-degree relatives receive screening after sudden death in the family.
Screening of first-degree relatives after sudden unexplained death is particularly important because therapy can alter the natural history of these diseases among surviving family members. Long QT syndrome has become the prototypical channelopathy because it has the highest known incidence rate in the population and because β-blocker therapy for primary prevention is associated with a 42% to 78% reduction in risk of aborted cardiac arrest or sudden cardiac death. Among the remaining channelopathies and cardiomyopathies there have been successes with various combinations of medications, left cardiac sympathetic denervation, ablation therapy, lifestyle modification, and avoidance of triggering medications. The most common channelopathy and cardiomyopathy diseases are long and short QT syndrome, Brugada syndrome, catecholaminergic polymorphic ventricular tachycardia (CPVT), ARVD, HCM and dilated cardiomyopathy (DCM). For exceptionally high-risk patients, primary prevention implantable cardioverter defibrillators (ICDs) may be offered although most electrophysiologists follow one or more ICDs that were placed in anticipation of a high-risk outcome before subsequent research helped downgrade the patient’s risk in the patient’s particular disease. Therefore extensive risk-benefit consideration and patient counseling are required to help families understand the balance between the benefits of primary prevention ICDs and the potential risks of ICD placement, including infection, shocks, device malfunction, subsequent interventions, device failure, and psychosocial implications.
Other diagnoses found in surviving relatives call for therapy that does not directly impact acute arrhythmia risk, but may decrease long-term morbidity and mortality. For example, early and severe atherosclerotic coronary heart disease on autopsy should trigger screening for familial hyperlipidemia. Autopsy may also uncover conditions such as undiagnosed CHD, vascular malformations that may have ruptured or otherwise caused sudden death, toxicology findings consistent with overdose, or myocarditis. While there are suggestions that there may be a heritable component to several of these disorders, the primary work to date has focused on channelopathies and cardiomyopathies, which are amenable to genetic testing for monogenetic autosomal dominant variants.
In summary, a sudden death event in a previously healthy young person is a dramatic signal that first-degree relatives—siblings, parents, and children—require risk stratification.
Phenotype Screening Among First-Degree Relatives
Several cardiac societies have endorsed a systematic approach to phenotype screening among first-degree relatives of a sudden “unexplained” death victim. Sudden unexplained death occurs when autopsy and toxicology do not identify a cause of death and toxicology does not suggest a toxic exposure. The circumstances of the death should be investigated by interviews with relatives or in collaboration with the medical examiner or coroner’s office. An expert opinion on cardiac histology can be useful; slides can be mailed for a second opinion. Overinterpretation of cardiomyopathy or inflammatory causes in unexperienced hands is more common than underinterpretation. The danger of overinterpretation is that it narrows the spectrum of further investigation in first-degree relatives and puts the remainder of the family at risk for a missed diagnosis of a potentially heritable disorder.
While phenotypic evaluation of the decedent is being completed by the coroner, medical examiner, or any consultants, an evaluation of first-degree relatives should begin as soon as the family is willing. Doctors and families have the same priorities: first-degree relatives with symptoms, especially syncope, palpitations, or known arrhythmias, should be screened as quickly as feasible. The initial steps are noninvasive, beginning with history and physical examination. Testing should include an ECG in standard 12-lead position and an ECG in a modified position with V 1 and V 2 in the second intercostal space. This position oversamples the right ventricular outflow tract, where Brugada syndrome and ARVD have their central focus of histopathology. Echocardiography provides information on ventricular dimensions and function. Exercise stress electrocardiography provides information on exercise-sensitive arrhythmia, especially CPVT. Myocardium with marginal perfusion can also be identified by exercise stress testing in some cases (e.g . , severe premature coronary artery disease). A consensus statement endorses these noninvasive studies in first-degree relatives of a young sudden death victim as class I recommendations. More invasive or complex diagnostic studies have been used with varying efficacy. They retain class IIA and IIB recommendations in the consensus statement because the test characteristics show neither excellent specificity nor excellent sensitivity. As one example, a study from the Cardiac Arrest Survivors with Preserved Ejection Fraction (CASPER) registry performed provocative testing with sodium channel blockers among survivors of cardiac arrest or first-degree relatives with a family history of sudden death. In this slightly older cohort (47 years of age ±15 years), testing provoked a Brugada pattern in only 6.9% of subjects, less than half of which were uncovered in subjects with normal resting ECGs and only one of the induced cohort had a positive genotype. In our center, we take a staged approach to screening first-degree family members, beginning with history and physical, two ECGs, echocardiography, and exercise stress testing (class I indications) and only moving to more advanced testing if history and physical or initial testing suggests that further screening is required (see Fig. 89.1 for a diagrammatic representation of our screening protocol). Although the consensus statement currently recommends discharging asymptomatic, fully grown adults with negative workups, a low rate of persistent events continues to occur in diagnosis-negative relatives and our understanding of the genetic etiologies remains imperfect. Therefore in our center, we continue to see phenotype-negative families at long follow-up intervals to revisit clinical testing as needed and reconsider the state of genotype testing in the family.

Genotype Screening Among First-Degree Relatives
Significant efforts have already focused on evaluating postmortem DNA samples. Although there is near-universal consensus that saving a genetic sample from the decedent is the best practice, there are several hurdles to overcome. These hurdles include the variability in practice among coroners and medical examiners’ offices, the understandable desire of the family to have the body released back to their care for burial as soon as possible, the degradation of DNA in postmortem samples prior to collection, and the practical considerations of getting material saved, stored, and DNA extracted effectively.
Table 89.3 tabulates the yield of postmortem genetic screening after sudden unexplained death. There continues to be discussion within the field about the best genetic approach. Some studies performed genetic screening panels using a small, predetermined list of genes firmly implicated in sudden death. Others have used whole exome or whole genome sequencing, to sample more broadly across the genome, using computational tools to evaluate 100 or more genes. As costs of next-generation sequencing decrease, the latter approach is likely to be increasingly available. However, the risk of larger panels is the discovery of variants of unknown significance or variants in genes with unclear application to the case at hand.
First Author, Year, Reference Number | Number of Genes Screened | Number of Probands | Yield of Pathogenic and Likely Pathogenic Variants (%) |
---|---|---|---|
Methner, 2016 | 64 | 71 | 3.7 |
Winkel, 2012 | 3 | 44 | 11 |
Lahrouchi, 2017 | 77 | 302 | 13 |
Skinner, 2011 | 5 | 52 | 15 |
Chugh, 2004 | 5 | 12 | 16 |
Farrugia, 2015 | 23 | 16 | 19 |
Wang, 2014 | 6 | 274 | 20 |
Di Paolo, 2004 | 5 | 10 | 20 |
Behr, 2008 | 11 | 57 | 21 |
Tester, 2012 | 6 | 173 | 26 |
Bagnall, 2016 | 131 a | 490 | 27 |
Nunn, 2015 | 135 | 59 | 29 |
Anderson, 2016 | 100 | 32 | 32 |
Christiansen, 2016 | 100 | 61 | 34 |
Interpretation of genetic information can be exceptionally difficult in sudden unexplained death cases. A recent study in 302 sudden arrhythmic death cases that searched specifically for clinically actionable pathogenic or likely pathogenic variants found that molecular autopsy combined with clinical evaluation increased the diagnostic yield in surviving families from 26% to 39%. Families should be forewarned before molecular autopsy is undertaken that genetic information is additive and rarely diagnostic in isolation. In summary, genetic sequencing and interpretation is a parallel process that informs phenotypic workup, but does not replace it. As Fig. 89.1 shows, molecular autopsy can be performed simultaneously with the family’s phenotypic workup, using genetic information to guide the workup as necessary, but incorporating phenotypic information to make a final diagnosis in the family. From there, clinicians can determine the value of lifestyle modifications, pharmacotherapy, interventional therapy, and family cascade screening.
Ethical Considerations and Future Directions (See Also Chapter 83 )
Families require careful counseling prior to genetic screening after a sudden death episode. Despite everyone’s best intentions, there are potential downsides to testing surviving family members for a genetic variant that is found in the decedent. In the United States, federal law (the Genetic Information Nondiscrimination Act) prohibits discrimination by health insurance companies, group health plans, employers, labor unions, and employment agencies on the basis of genetic testing information. This law does not protect against use of genetic testing information by companies that sell life insurance, disability insurance, or long-term care insurance. In addition, while the decedent’s insurance will occasionally cover the cost of molecular autopsy, this is not universal, and providers should be transparent with families about the out-of-pocket costs of molecular autopsy prior to proceeding.
Screening for Atherosclerotic Cardiovascular Disease Risk Factors
The chronic process of atherosclerosis begins in early life, with initial changes observed even in the fetus. In two contemporary studies, Pathological Determinants of Atherosclerosis in Youth and the Bogalusa Heart Study, autopsies of youth who died from unintentional injury revealed that the severity and extent of atherosclerosis in childhood correlated strongly with the presence and intensity of traditional risk factors such as dyslipidemia, hypertension, obesity, tobacco smoke exposure, and diabetes mellitus. Multiple observational studies indicate that risk factors measured in childhood predict subclinical atherosclerosis in adulthood, often more strongly than do risk factors measured in adulthood. Finally, the Princeton Lipid Research Clinics Follow-up Study demonstrated that the metabolic syndrome risk factor cluster in childhood predicted actual clinical cardiovascular disease at ages 30 to 48 years. Taken together, these observations create a strong chain of evidence linking risk factor development in early childhood to atherosclerotic cardiovascular disease in adulthood.
Nevertheless, because of the long period between childhood and adult events, there are no randomized controlled trials of childhood screening for risk factors to reduce adult clinical atherosclerotic cardiovascular disease events. Such trials are unlikely to ever occur. For this reason, there has been some debate in the medical community about the utility and cost-effectiveness of screening, particularly regarding dyslipidemia. This will be reviewed below.
Of the traditional risk factors for atherosclerotic cardiovascular disease, only dyslipidemia screening will be discussed here. While very important, screening for the remainder of the risk factors generally occurs in the primary care clinic, with most centers referring patients with these risk factors to noncardiology specialists (nephrology for hypertension, endocrinology for diabetes, weight management clinic for obesity) when required for management. An excellent review of the evidence and expert recommendations for screening for these risk factors in childhood can be found in the 2011 National Heart, Lung, and Blood Institute (NHLBI) Integrated Guidelines for Cardiovascular Risk Reduction in Children.
Dyslipidemia
Dyslipidemias are disorders characterized by abnormal lipoprotein metabolism resulting in increased total cholesterol (TC), increased low-density lipoprotein cholesterol (LDL-C), increased triglyceride, and/or decreased high-density lipoprotein cholesterol (HDL-C). These lipid abnormalities can be caused by genetic mutations, environmental factors such as diet and activity level, or secondary to other processes such as kidney disease and organ transplantation.
The most important genetic dyslipidemia to consider is familial hypercholesterolemia (FH), which is caused by heterozygous or homozygous mutations in the gene encoding the LDL receptor or related proteins. FH is likely when the fasting LDL-C level is 160 mg/dL or greater in childhood or 190 mg/dL or greater in adulthood, there is a positive family history of hyperlipidemia or premature coronary disease, and secondary causes (such as hypothyroidism) have been excluded. Heterozygous FH has an estimated prevalence of 1 in 250 in the United States and accelerates atherosclerotic cardiovascular disease risk by up to 30 years, while homozygous FH has an estimated prevalence of 1 in 1 million to 1 in 160,000 and causes cardiovascular disease events in childhood. FH has the highest prevalence of all the genetic defects that cause significant premature mortality, and this formed the basis of early screening programs for childhood dyslipidemia, as described below.
When considering dyslipidemia in childhood, it is important to understand developmental changes in lipid levels that occur in healthy children. Lipids and lipoprotein levels start low at birth, rise slowly over the first 2 years of life, and then stabilize until puberty. During puberty, cholesterol levels decrease before rising again in late adolescence and early adulthood. In particular, the expected decline in cholesterol levels during puberty must be anticipated when interpreting and managing lipids in this age group.
Selective Screening Versus Universal Screening for Dyslipidemia
There has been significant debate on the role of universal versus selective screening for dyslipidemias in children and adolescents. In 1992, the National Cholesterol Education Program (NCEP) Expert Panel on Blood Cholesterol Levels in Children and Adolescents recommended targeting screening to children with family history of early coronary artery disease, family history of hypercholesterolemia, or unknown family history. Such a strategy seeks to identify children with FH. However, after publication of the 1992 NCEP guidelines, new data were reviewed in the follow-up American Academy of Pediatrics report on Lipid Screening and Cardiovascular Health showing that selective screening missed between 30% and 60% of children with dyslipidemias. Children with FH may not be detected with this strategy if their parents are unaware of the family history, and in fact, screening children has been advocated as a strategy to identify adult relatives with this condition since the diagnosis may be more straightforward in childhood. Moreover, with the continued increase in the prevalence of pediatric obesity, there has been a shift in the patterns of childhood dyslipidemia from primarily isolated elevated LDL-C due to FH to the increasingly common obesity-associated pattern: moderately to severely elevated triglycerides, decreased HDL-C, and normal to mildly elevated LDL-C. Both patterns are associated with accelerated atherosclerosis. Because childhood dyslipidemia is identifiable, tracks into adulthood, and is amenable to intervention, the 2011 NHLBI Guidelines for Cardiovascular Health and Risk Reduction in Children and Adolescents recommend universal screening before puberty (9 to 11 years of age) and again after puberty (18 to 21 years of age).
Recommended Screening for Dyslipidemia
As mentioned above, the NHLBI Guidelines provide age-specific recommendations for pediatric providers to screen for cardiovascular disease risk factors including dyslipidemias. The document also provides guidance on reinforcing healthy lifestyle habits and providing treatments to decrease these risk factors, starting in infancy. For dyslipidemia, the Guidelines recommend:
- ■
Universal screening at 9 to 11 years of age (before puberty) and again at 18 to 21 years of age (after puberty) with random non–HDL-C level or fasting lipid profile.
- ■
Screening from 2 to 8 years of age and 12 to 16 years of age with a fasting lipid profile only if there is a positive family history, other risk factors for cardiovascular disease, or a moderate- or high-risk condition.
- ■
No routine lipid screening from birth to 1 year of age.
The above guidelines are delineated in great detail in the 2011 NHLBI Cardiovascular Risk Guidelines, as mentioned. The reader is referred to this review for additional details.
Future Considerations for Dyslipidemia Screening
In 2016, the United States Preventive Services Task Force reviewed the literature and concluded that there is insufficient evidence to assess the benefit of universal versus targeted screening for dyslipidemias in children and adolescents. This conclusion was based on a lack of large, randomized control trials in the pediatric population evaluating targeted versus universal screening for dyslipidemia and effectiveness of early detection and treatment on intermediate and long-term outcomes. As mentioned above, the long timeline (decades), cost, and ethical considerations of such studies in children are prohibitive. Modeling approaches may offer some insight into these questions, but to date these data are lacking.
However, available data indicate that initiation of statin therapy in children and adolescents with high LDL-C due to familial hypercholesterolemia restores endothelial function and slows or even reverses progression of atherosclerosis as indicated by carotid intimal media thickness. Importantly, multiple studies have demonstrated the safety of statin therapy in children and adolescents. Furthermore, clinical trial data indicate that lifestyle modification can improve intermediate outcomes in children with multifactorial dyslipidemia. Given the relatively high prevalence of FH as the prototypical genetic dyslipidemia and the very high prevalence of obesity in children, effective dyslipidemia screening in the younger populations will continue to have an important role in decreasing adult atherosclerotic cardiovascular disease burden.
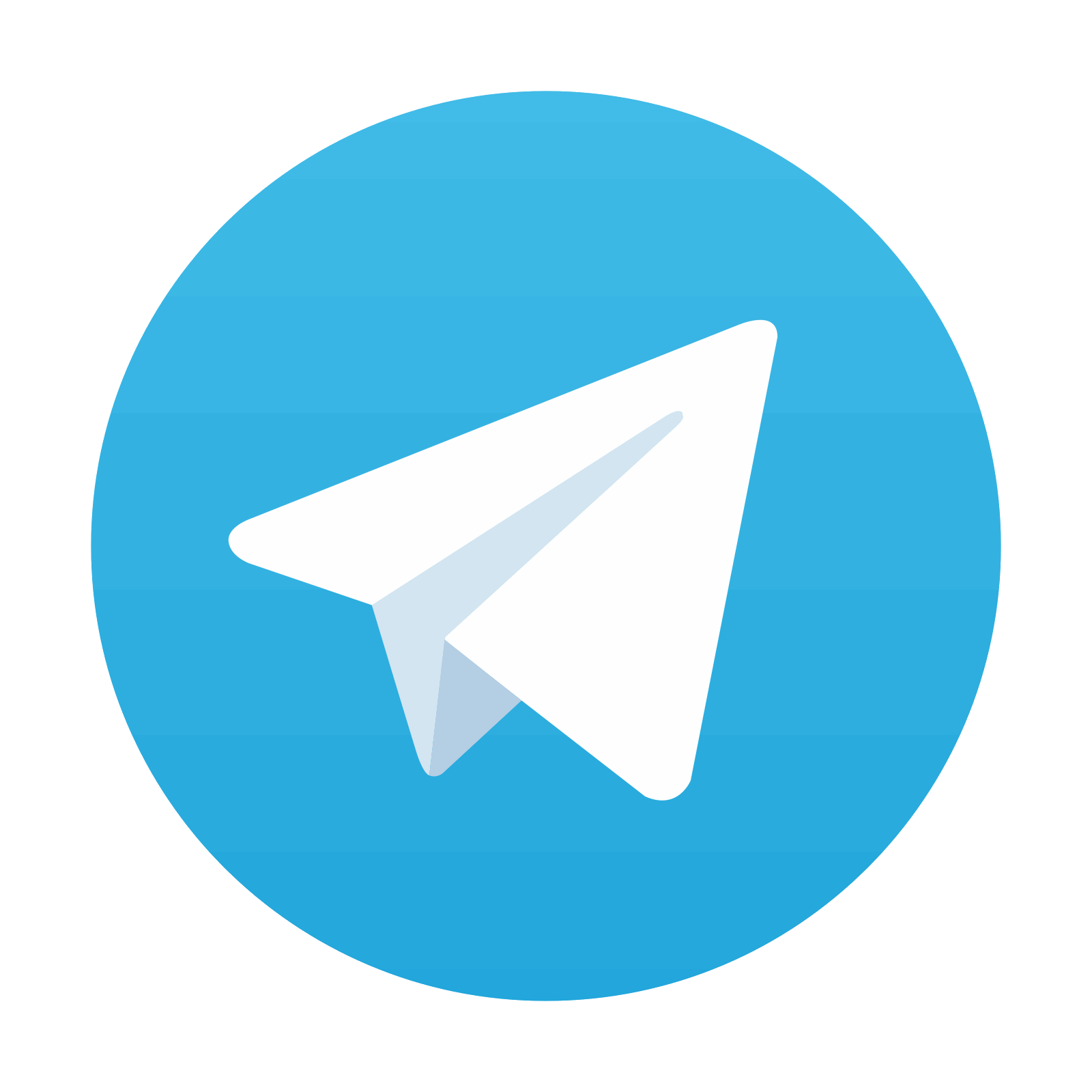
Stay updated, free articles. Join our Telegram channel

Full access? Get Clinical Tree
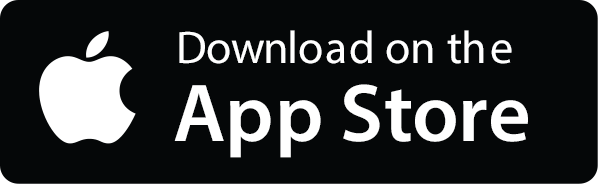
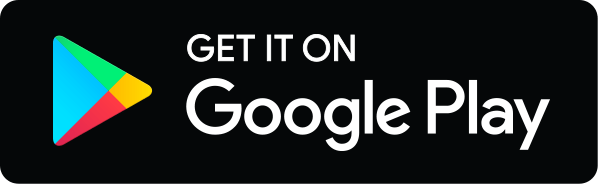