A 66-year-old man with cardiogenic shock, severe left ventricle (LV) systolic dysfunction, multiorgan failure and rhabdomyolysis caused by influenza B virus infection was admitted to the Intensive Care Unit. He needed circulatory support with veno-arterial extracorporeal life support, intra-aortic balloon pump, inotropes, and vasopressors. In addition, mechanical invasive ventilation and continuous renal replacement therapy were needed to maintain respiratory function, acid-base equilibrium, and fluid electrolyte balance as well as to treat rhabdomyolysis. Antibiotic and antiviral therapies were tailored by culture and serology. However, after seven days, LV systolic function was not improved, the LV was dilating, and filling pressure was increasing. Thus, the patient underwent left ventricular assist device (LVAD) implantation as bridging therapy to recovery or to transplantation. Initially, the LVAD reduced LV filling pressure and volume, and in the following weeks LV systolic function slowly improved. After two months, LV systolic function recovered completely, the LVAD was removed, and the patient was transferred to a peripheral hospital for rehabilitation.
Ventricular assist devices (VADs) were introduced in clinical practice in the 1980s as mechanical support for the left ventricle (left ventricular assist device [LVAD]), right ventricle (right ventricular assist devices [RVAD]), or both (biventricular assist device [BiVAD]) in patients with end-stage heart failure (HF) headed to transplant or in patients with temporary cardiac shock who were expected to recover ventricular function. Use of these devices increased in the years that followed, and in 2018, VADs, particularly LVADs, are considered a pivotal treatment in patients with HF; these devices have several indications, including bridge to transplant, bridge to candidacy, destination therapy, and bridge to recovery. Moreover, LVAD is considered the only valid alternative treatment when there are not enough organs available for transplant to satisfy the growing need. Indeed, the global HF prevalence, currently estimated between 23 and 26 million people, is expected to increase as a result of the population aging and the improved survival rates associated with other forms of heart disease.1 The huge need for treatment of this population of HF patients easily explains why from 2006 to 2014 more than 15,000 patients in the United States received a VAD implant, with total procedures approaching 2500 per year and with 1- and 2-year survival rates of 80% and 70%, respectively.2
LVADs are composed of an inflow cannula, usually placed in the left ventricular (LV) apex; an outflow cannula, usually anastomosed in the ascending aorta; a propulsion pump that moves the blood provided by the inflow cannula to the aorta through the outflow cannula; and an external controller, which receives and processes information from the pump. According to the pumping mechanisms, LVADs can be classified as either pulsatile or continuous flow. Pulsatile LVADs, the first generation of VADs available, were characterized by a pumping mechanism that simulated the pulsatile action of the heart with a rate that could be fixed or self-adjusting according to preload. These VADs, including the Novacor LVAS (Novacor, Salt Lake City, UT), the HeartMate vented electric LVAD (Abbott, Abbott Park, Illinois), and the Abiomed BVS 5000 (Abiomed, Danvers, MA), could be extracorporeal (the pump located outside the body) or intracorporeal (the pump inside the body, usually in the left chest or upper abdomen). All pulsatile LVADs have unidirectional valves in the inflow and outflow cannulae that increase the risk of thrombus formation, are usually large, and are highly dependent on adequate preload. These limitations caused the development of a new generation of LVADs, characterized by pumping mechanisms that guaranteed a continuous flow. These devices are smaller, always intracorporeal, do not require unidirectional valves, and are able to provide higher flows at lower pressures than pulsatile LVADs. Continuous-flow LVADs can be further classified as axial-flow LVADs (MicroMed DeBakey, MicroMed Technology, Houston, TX; HeartMate II, Abbott, Abbott Park, Illinois; Jarvik 2000, Jarvik Heart, New York, NY) and centrifugal-flow LVADs (HeartMate III, Abbott, Abbott Park, Illinois; HeartWare, HeartWare International Inc., Framingham, MA), depending on the mechanical structure of the pump. In both axial- and centrifugal-flow LVADs, preload can be reduced by increasing the speed of the rotor or increased by decreasing the speed of the rotor.
Echocardiography commonly is considered the most important diagnostic tool in the selection and management of patients requiring LVAD therapy, as indicated by the International Society for Heart and Lung Transplantation’s current guidelines for mechanical circulatory support (2013).3 Indeed, echocardiography is a commonly available, easy, cheap, and fast diagnostic technique, and it is able to provide all of the information the clinician needs to manage patients with end-stage HF. The fundamental goals of the echocardiographic evaluation of these patients are the identification of eligibility for LVAD implantation, the stratification of the risk profile of the individual patient, the perioperative evaluation of complications that could onset during the implantation, and a serial follow-up to detect eventual device malfunction. Preoperative and follow-up evaluations usually are performed by 2-dimensional (2D) transthoracic echocardiography (TTE), whereas the perioperative assessment is performed by 2D transesophageal echocardiography (TEE). Recently, new technologies, including 3-dimensional (3D) echocardiography and strain measurements, were tested in this setting and shown to provide additional information for the management of these patients.
One of the most important roles of the echocardiographic assessment of patients with end-stage HF is the evaluation of possible eligibility for LVAD treatment. A positive opinion depends on the balance between the advantages conferred by the implant and the risk stratification of the individual patient.
The study of the LV plays a pivotal role in the evaluation of these elements. Several clinical and echocardiographic variables have been tested, and reduced LV ejection fraction (EF), altered LV geometry, and restrictive LV filling pattern, calculated through power Doppler assessment of the mitral inflow pattern, have been shown to have the most powerful ability to identify high-risk patients.4-6 LV volumes usually are measured using 2D TTE, and EF is calculated by a biplane method of disks summation (modified Simpson’s rule), tracing the blood-tissue interface in the apical 4- and 2-chamber views.7 However, it recently was demonstrated that 3D echocardiography can provide a more accurate estimation of LV volumes and EF because it does not rely on geometric assumptions and is not affected by foreshortening of the LV apex.8 For this reason, when available, 3D TTE should be considered the technique of choice for the calculation of LV volumes and EF.7 The use of score systems that combine clinical and echocardiographic variables has been shown to improve risk stratification. A recently proposed score combined 5 TTE variables (end-systolic volume index, left atrial volume index, mitral E-wave deceleration time, tricuspid annular peak systolic excursion, and pulmonary artery systolic pressure) and demonstrated a great ability to predict mortality, the rate of which increased significantly with an increase of the score from 0 to 5, with a 4-fold risk of death in the high-risk subgroup of patients (score 5) compared with the low-risk subgroup.4
Recently, new myocardial deformation measurements, such as speckle-tracking echocardiography (STE) strain and strain rate, have provided additional information for further evaluation of this population of patients. STE strain is considered one of the most feasible and sensitive tools in the early diagnosis of cardiac dysfunction; it allows a frame-by-frame tracking of natural acoustic markers within the myocardium, and is angle independent, not influenced by translational movement due to respiration and tethering from adjacent myocardium, and not sensitive to signal noise. Global longitudinal strain (GLS) appeared to be the most sensitive and effective STE parameter for the evaluation of LV function; in patients with end-stage HF, a reduced GLS was more significantly associated with poor outcomes (all-cause mortality, cardiovascular death, heart transplantation, and arrhythmic events)9 than EF and wall motion score index (WMSI), particularly in patients with a very low EF.10,11 Moreover, similar results have been obtained using 3D STE. This new technology based on 3D datasets can overcome 2D limitations, such as the significant speckle decorrelation that takes place when out-of-plane motion occurs, and has been shown to be a sensitive and accurate predictor of cardiovascular-related death or hospitalization due to HF.12,13
The evaluation of right ventricle (RV) function perhaps plays an even more important role than the study of LV function in patients with end-stage HF. Indeed, in this subgroup of subjects, reduced RV function is not only a powerful prognostic tool for the prediction of poor outcomes,14 it also is a fundamental marker of an increased risk of developing post-LVAD implantation RV failure (RVF),15-17 one of the most life-threatening post-implant complications.
The echocardiographic assessment of RV size and function is not always easy due to the shape and location of the RV; however, using the apical 4-chamber, parasternal short-axis, and subcostal views, sometimes slightly modifying the angulation or shift of the transducer to better include the entire chamber, it is possible to obtain a fairly detailed evaluation of the RV.18 Right ventricular ejection fraction (RVEF), when calculated by 2D echocardiography, is not considered a reliable estimation of RV systolic function because the complex RV shape and geometry, with inflow and outflow portions in different planes, make the measurement ineffective. On the other hand, as previously underlined in regard to the LV, 3D echocardiography can overcome these limitations in the quantification of RV volumes and EF, providing a comprehensive, reproducible assessment of the RV chamber that is no longer based on geometric assumptions; thus, it is possible to obtain an accurate and feasible estimation of RV function.19
RV systolic function often can be evaluated by 2D echocardiography through several parameters, including tricuspid annular plane systolic excursion (TAPSE), tricuspid annular longitudinal velocity by tissue Doppler imaging (TDI S’), right-sided index of myocardial performance (RIMP), and fractional area change (FAC). Of these measurements, FAC has been shown to be the most reliable for the assessment of RV systolic function, with a good correlation with RVEF estimated by cardiac magnetic resonance.20 It is calculated in the apical 4-chamber view by obtaining end-diastolic and end-systolic RV areas by planimetry of the endocardial border, and, when reduced, it proved to be an effective predictor of poor outcomes in patients with end-stage HF.21,22 However, FAC quantification is dependent on image quality, as wrong tracing of the RV area can lead to underestimation or overestimation of RV function. When a reliable estimation of FAC is not possible, TAPSE, a mono-dimensional measure of RV longitudinal function obtained by quantifying the displacement of the lateral portion of the tricuspid annulus in the apical 4-chamber view, has been shown to be a valid alternative to predict outcomes in these patients.23
However, the new myocardial deformation measurements, recently tested with great results in the study of RV systolic function, are able to provide additional information about RV systolic function, improving the risk stratification algorithms in use. Two-dimensional STE GLS, the most representative and reproducible strain for the assessment of RV function, recently has emerged as the most accurate and sensitive tool for the evaluation of RV function and as the most powerful independent predictor of severe adverse events in patients with HF.24,25 RV GLS is obtained only from the apical 4-chamber view, and it could reflect the average value of the RV free wall and septal segments (RV GLS) or of the RV free wall (RV FWLS) alone. In patients with end-stage HF, a more impaired RV strain was associated with increasing New York Heart Association class, greater LV volumes, and worse LV systolic function, and it was able to predict long-term adverse events with an incremental prognostic value to LVEF.26 These findings reinforce the concept that RV systolic function is a key element in the prediction of poor outcomes in this population.
However, as previously mentioned, RV function also is a fundamental factor in the evaluation of a patient’s risk profile for the development of one of the most dangerous post-LVAD implantation complications, RVF. While RVF is more common in patients with pulsatile-flow devices, it still occurs in 13% to 40% of continuous-flow device patients.27 Several definitions have been provided to indicate RVF; however, the Interagency Registry for Mechanically Assisted Circulatory Support (INTERMACS)28 defines RVF by the need for an RVAD, inhaled nitric oxide, or inotropic therapy for more than 1 week at any time after LVAD implantation in the presence of symptoms and signs of persistent RV dysfunction, such as central venous pressure >18 mm Hg with a cardiac index <2.3 L/min/m2 in the absence of elevated left atrial or pulmonary capillary wedge pressure (>18 mm Hg), cardiac tamponade, ventricular arrhythmias, or pneumothorax.
Currently, the pathophysiology of RVF is not completely explained. The main factors include (1) interventricular dependence; (2) the sudden increase of RV preload due to an acute increase in venous return after the restoration of cardiac output, which can determine the dilatation of the RV with an exacerbation of tricuspid regurgitation (TR); and (3) some perioperative events—including suboptimal ventilation, alveolar hypoxia, hyperinflation, acidosis, RV ischemia, bleeding, and transfusions—that could worsen RV function. Evaluation of the patient’s risk profile includes assessment of clinical, hemodynamic, and echocardiographic variables. Several different clinical elements have been considered by authors to predict RVF—including laboratory parameters, blood pressure, inotrope dependency, obesity, and so on—and many scores were proposed.29-33 However, all the schemes perform only modestly when applied to external populations.34 Therefore, they are not commonly used in clinical practice.
Hemodynamic measurements, including pulmonary vascular resistance (PVR) and pulmonary artery systolic pressure (PASP), can be quantified invasively by catheterization or indirectly by echocardiography. Pulmonary hypertension (HTN) and high PVR seem to be associated with poor outcomes after LVAD implantation,35 but there is no agreement among authors about the real value of these parameters in the risk stratification for RVF.31,33
On the other hand, echocardiography is the most important tool for the evaluation of a patient’s risk profile because it is able to provide a large number of measurements noninvasively. Conventional parameters already discussed showed a good ability to predict RVF onset. Dilated RV; reduced systolic function as demonstrated by decreased FAC,35 TAPSE,36 and TDI S’; and moderate-to-severe TR32,37 are the main predictors of RVF and poor outcomes after LVAD implantation. Very interesting results have been obtained using 2D STE parameters. Reduced 2D speckle-tracking strain and strain rate predict a high risk of RVF onset with greater sensitivity compared with conventional parameters,27,38-41 whereas the improvement of these parameters during follow-up suggests a low-risk profile (Table 37-1).42,43 These new measurements seem to be the most reliable and accurate elements for the risk stratification of RVF and, unless current data are contradicted by larger future studies, they should be considered essential tools in the echocardiographic preoperative assessment of these patients.
Reference | Number of Patients | Main Findings |
---|---|---|
Grant AD et al (JACC 2012)27 | 117 |
|
Dandel M et al (Circulation 2013)38 | 205 |
|
Kalogeropoulos AP et al (Eur Heart J Cardiovasc Imaging 2015)39 | 120 |
|
Cameli M et al (J Heart Lung Transplant 2013)40 | 10 |
|
Kato TS et al (JACC Heart Fail 2013)41 | 68 |
|
Cameli M et al (Transplant Proc 2015)42 | 19 |
|
Herod JW et al (J Card Fail 2014)43 | 17 | RV systolic strain, systolic strain rate, and RV diastolic strain rate improved after LVAD implantation. |
Patients found to be eligible for LVAD implantation should be evaluated by 2D TTE, with attention focused on the detection of specific elements that can influence the surgical procedure and clinical outcomes. Right-to-left shunting, such as patent foramen ovale and interatrial or interventricular septal defects, should be carefully searched. Indeed, the LV unloading performed by an LVAD causes a drastic reduction in left chamber pressure, whereas right chamber pressure is not significantly modified; this sudden imbalance of pressure can exacerbate a right-to-left shunt or induce reversal of the shunt direction from left to right to right to left, causing hypoxemia and raising the risk of paradoxical embolism.44 Two-dimensional Doppler TEE should be considered the technique of choice for the detection of intracardiac shunts because it has been shown to be a very accurate and sensitive, although invasive, test. The use of agitated saline contrast agents with the Valsalva maneuver should be avoided in this context because the high LV filling pressure before implantation can make the maneuver useless and result in an underestimated diagnosis.
Another important factor that should be taken into account is the high risk of thrombosis in these patients. Indeed, the very low LVEF that characterizes these subjects is the main risk factor for thrombus formation. The LV, especially the apex, should be carefully examined to find thrombi, which commonly cause inflow cannula obstruction or embolism. Conventional apical views usually allow a good exploration of the left chambers; however, when the image quality is not satisfactory or the presence of clots cannot be excluded safely, the use of intravenous contrast agents or TEE could improve the accuracy of detection.
Preoperative evaluation of cardiac valve function plays an important role because the evidence of severe valve dysfunction could modify the surgical procedure. Indeed, some alterations can worsen after LVAD activation or can make the LVAD implant ineffective. The current International Society for Heart and Lung Transplantation guidelines for mechanical circulatory support (2013)3 recommend surgical repair or valve replacement during device implantation when severe valve dysfunction is detected; however, concurrent valve replacements increase the mortality rate after LVAD implantation45 and they are an important risk factor for RVF. For these reasons, valve function should be evaluated carefully, using quantitative parameters and, when necessary, improving the accuracy of the measurements using 2D TEE or 3D echocardiography, the gold standard for the evaluation of valve anatomy and function.46-48
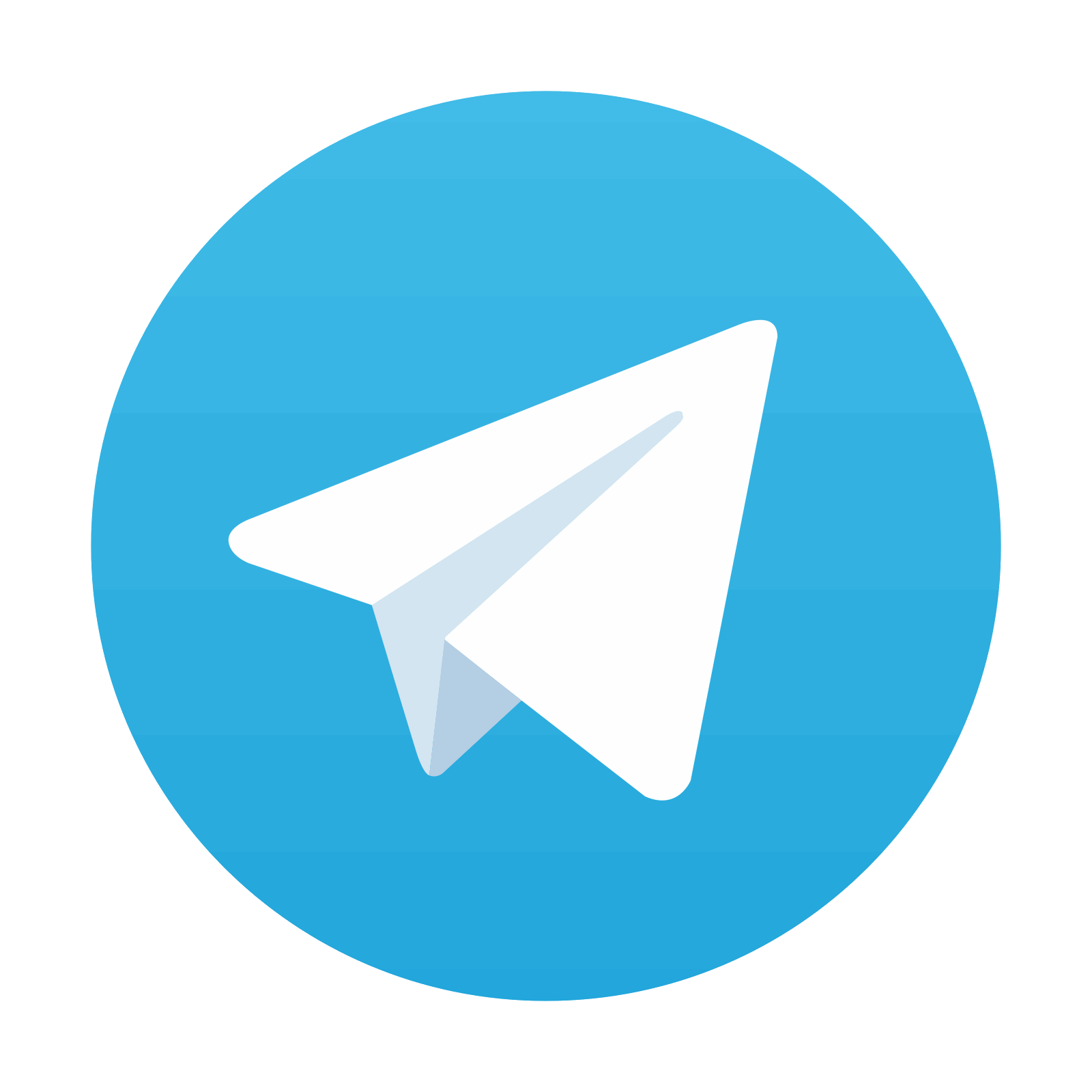
Stay updated, free articles. Join our Telegram channel

Full access? Get Clinical Tree
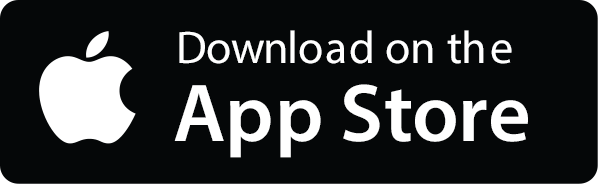
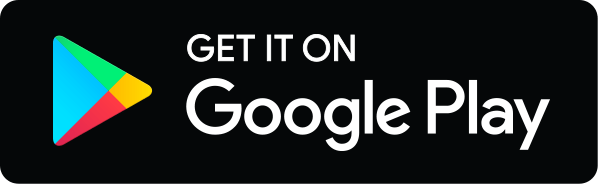