Fig. 1
(a) Dynamic cycle of DNA demethylation and remethylation (DNMT DNA methyltransferase, SAM S-adenosyl methionine, 5mC methylcytosine, 5hmC hydroxymethylcytosine, 5fC 5-formylcytosine, 5caC carboxylcytosine, TET ten-eleven translocation enzyme, TDG thymine-DNA glycosylase, TDG/BER TDG-mediated base excision repair) (b) DNA methylation by DNMTs
Subsequent induction of methyl group modification by DNA methyltransferases 1, 3A and 3B (DNMT1, DNMT3A and DNMT3B) maintains DNA methylation patterns. DNMT3A and DNMT3B induce de novo DNA methylation whereas DNMT1 primarily methylates the opposite strand of 5mC, maintaining DNA methylation patterns (Fig. 1b). This model of DNA demethylation and remethylation is the most widely accepted, although several other mechanisms of active DNA demethylation and remethylation have been proposed (Chen et al. 2013; Wang et al. 2014b; Thillainadesan et al. 2012a).
3 DNA Methylation and Gene Transcription
DNA methylation at gene promoter sequences is generally associated with transcriptional silencing or decreased gene expression. DNA methylation physically disturbs the locus by preventing transcriptional proteins binding to the promoter, and by interacting with proteins known as methyl-CpG-binding domain proteins (MBDs). MBD proteins attract additional proteins to the locus, including histone deacetylases and other chromatin remodeling proteins. This forms compact, inactive chromatin, termed heterochromatin.
DNA methylation has been shown to play a role in the transcriptional activity of a number of genes involved in blood pressure regulation. The renin-angiotensin-aldosterone system (RAAS) plays a major role in efficient fluid retention, and as such is involved in maintaining blood pressure, and in the pathogenesis of essential hypertension. Components of RAAS including human AGT (Wang et al. 2014a), human ACE (Riviere et al. 2011), rat Agtr1b (Bogdarina et al. 2007) and human CYP11B2 (Demura et al. 2010) have been shown to be negatively regulated by DNA methylation. Similarly, when CpG island sequences of the human AGTR1 promoter were cloned into a reporter plasmid its transcriptional activity was dependent upon DNA methylation (unpublished observation). DNA methylation has been demonstrated to decrease the transcriptional activity of HSD11B2 whose loss of function mutation causes the syndrome of apparent mineralocorticoid excess and hypertension (Alikhani-Koopaei et al. 2004). In spontaneous hypertensive rats, increased expression with low promoter DNA methylation is observed in Slc12a2 that maintains ionic balance, thereby regulating blood pressure (Lee et al. 2010).
4 DNA Methylation Dynamics During Intrauterine Programming
After fertilization, the erasure of epigenetic factors (called genomic initialization) progresses until embryo implantation (preimplantation programming). The initialized cells attain the totipotent ability to change into any cell type. In humans, DNA methylation patterns are largely erased in the paternal genome, except in paternally imprinted regions and transposable elements. In contrast, the maternal genome methylation patterns are almost entirely maintained and demethylation occurs to a much lesser extent (Okae et al. 2014). Global DNA demethylation of the mouse maternal genome, however, takes place by passive dilution through DNA replication during initialization (passive DNA demethylation) (Lee et al. 2014).
DNA remethylation on both paternal and maternal genome occurs during human embryonic development, with global DNA methylation level reaching at almost 80 % in the early post-implantation embryos (Guo et al. 2014, 2015). Cell lineage-specific DNA methylation patterns are established through the course of differentiation. Primordial germ cells (PGCs), which can give rise to sperm and oocytes, show a drastic decrease in global DNA methylation to less than 10 % at approximately 10–11 weeks of gestation (Guo et al. 2015). In human somatic tissues a tissue-specific signature exists at 9 weeks gestation, however, further gain or loss of DNA methylation occurs in a substantial number of genomic regions during the first and second trimester, and the DNA methylation patterns in fetal somatic tissues become comparable to those of the corresponding adult tissues. De novo DNA methylation is associated with cessation of developmental processes, while DNA demethylation is observed, thereby activating tissue-specific functions (Slieker et al. 2015). DNA sequence-specific-transcription factors regulate both active DNA demethylation and de novo DNA methylation during embryonic (Feldmann et al. 2013; Lienert et al. 2011) and fetal (Slieker et al. 2015) development. In contrast to DNA methylation patterns during adulthood, which remain relatively stable, DNA methylation dynamics during intrauterine life are much more dramatic.
Although DNA methylation is extensively erased in human preimplantation embryos and PGCs, the erasure is incomplete (Tang et al. 2015). In addition to changes in DNA methylation related to development and differentiation, DNA can also become hypermethylated or hypomethylated by maternal factors at various genomic loci in human fetal cells during intrauterine life (Drake et al. 2015; Chhabra et al. 2014; Chang et al. 2011; Chen et al. 2014). If changes in DNA methylation patterns occurring by maternal factors are not erased or diluted during intrauterine life, they tend to postnatally persist in somatic and germ cells. Maintained methylation patterns in somatic cells affect phenotype associated with DNA methylation patterns. In other words, the factor influences postnatal constitution. The epigenetic changes in germ cells are maintained and carried forward to the offspring, even in the absence of the initial trigger in a phenomenon known as transgenerational inheritance. The regions that evade global DNA demethylation in the preimplantation embryos and PGCs would potentially represent hotspots of transgenerational epigenetic inheritance (Tang et al. 2015).
5 Postnatal DNA Methylation Dynamics
Various environmental and health factors can induce changes in DNA methylation in both somatic and germ cells throughout postnatal life. Cytosines of CpG dinucleotides are either methylated or unmethylated at the cellular level. DNA methylation patterns in regulatory regions, including promoters and cis-acting elements, exert a profound impact on transcriptional activity. The number of cells with an unmethylated promoter determines the expression level of the corresponding gene in a given tissue. It therefore follows that tissue DNA methylation patterns are of physiological importance to constitution. Dynamic changes in DNA methylation patterns influence two crucial aspects of constitution (acquired predisposition) and non-Mendelian inheritance (transgenerational epigenetic inheritance) (Fig. 2).


Fig. 2
Proposed model: “DNA methylation changes susceptibility to hypertension”
It is important to understand the signals that influence the dynamics of DNA methylation patterns. In vitro and in vivo studies have shown that active and gradual DNA demethylation (Miller and Sweatt 2007; Miller et al. 2010; Wang et al. 2014a, b; Thomassin et al. 2001; Demura et al. 2015) (Fig. 3a) or de novo methylation (Wang et al. 2014b; Ishikawa et al. 2015; Thillainadesan et al. 2012b; Wajapeyee et al. 2013; Serra et al. 2014) (Fig. 3b) following long-term stimulation or suppression occurs around a transcription factor-binding site (TFBS) and a transcription start site (TSS), respectively. Stimulatory signals switch the gene expression phenotype from an inactive to an active state within a tissue, whereas suppressive signals switch the gene expression phenotype from an active to an inactive state (Fig. 4). Therefore, although changes in DNA methylation patterns almost always causes changes in transcriptional activity, they are a consequence rather than a cause.





Fig. 3
Schematic representation of dynamic changes in DNA methylation patterns by either stimulation (a) or suppression(b) (TSS transcription start site, pTFBS positive transcription factor-binding site, nTFBS negative transcription factor-binding site)

Fig. 4
Gene expression phenotype conversion
There are examples of what happens to DNA methylation patterns when transcriptional signals (activation or repression) are removed. In vitro studies using cultured hepatocytes showed that glucocorticoid-induced DNA demethylation at a distal enhancer element of the rat Tat gene is maintained for at least 3 months following glucocorticoid withdrawal (sustained type of active DNA demethylation) (Thomassin et al. 2001). Conversely, DNA demethylation within the promoters of both AGT (Wang et al. 2014a) and CYP11B2 (Demura et al. 2010) upon stimulation is reversed when the stimuli (interleukin 6 for AGT, potassium for CYP11B2) are removed (reversed type of active DNA demethylation) (Demura et al. 2015).
Miller et al. have elegantly offered valuable insight into the dynamics of DNA methylation patterns and memory formation. Fear conditioning transiently induces low DNA methylation at the Reln locus (that promotes memory), and high DNA methylation at the Ppp1cc locus (that suppresses memory) in the hippocampus where short memory is formed. These hippocampal changes are reversed 1 day following fear-conditioning removal (Miller and Sweatt 2007). By contrast, fear conditioning induces high DNA methylation, and associated decreased gene expression, in the memory suppressor Ppp3ca in the dorsomedial prefrontal cortex where remote memory is stored. This cortical change lasts at least 1 month following fear-conditioning removal (Miller et al. 2010). These examples demonstrate that some factor can evoke gene-targeted and bidirectional changes when and where needed, upregulating some genes with active DNA demethylation, and downregulating others with de novo DNA methylation.
Dynamic changes in DNA methylation patterns are typically divided into 4 types* reversed DNA demethylation (Miller and Sweatt 2007; Wang et al. 2014a; Demura et al. 2015) (Fig. 5a), sustained DNA demethylation (Thomassin et al. 2001) (Fig. 5b), reversed DNA methylation (Miller and Sweatt 2007) (Fig. 5c) and sustained DNA methylation patterns (Miller et al. 2010) (Fig. 5d). Sustained patterns of DNA demethylation (Fig. 5b) and methylation (Fig. 5d) are more likely to exert an impact on phenotype through epigenetic phenomena including acquired predisposition, transgenerational epigenetic inheritance and intrauterine programming.




Fig. 5
Schematic representation of dynamic changes in DNA methylation patterns upon some signal and its cessation. (a) Reversed DNA demethylation. (b) Sustained DNA demethylation. (c) Reversed DNA methylation. (d) Sustained DNA methylation (TSS transcription start site, pTFBS positive transcription factor-binding site, nTFBS negative transcription factor-binding site)
6 Molecular Mechanisms Underlying DNA Methylation Dynamics
Two independent research groups have described that transcriptional activation by estrogen induces cyclical DNA demethylation and remethylation of the promoters and first exons of several estrogen-responsive genes (Metivier et al. 2008; Kangaspeska et al. 2008). During estrogen stimulation, the TDG/BER system demethylates DNA by replacing a methylated cytosine with an unmethylated cytosine. The DNA is then remethylated by DNMTs. The periodic time interval of DNA demethylation and remethylation is approximately 20–40 min. However, long-term stimulation or suppression result in gradual DNA demethylation (Fig. 3a) or de novo methylation (Fig. 3b) around a TFBS and a TSS over days or years. The degree of changes in DNA methylation depends on distance from a TFBS or a TSS (Fig. 3). Therefore, the tendency to maintain DNA methylation patterns appear to be due to the competition between TDG/BER system and DNMT enzymes. Additional factors are required to induce gradual changes in DNA methylation patterns in the face of this competition.
A balance between DNA methylation and demethylation activities in the nucleus has a role in the gradual changes in DNA methylation patterns. More importantly, protein complexes responsible for DNA methylation and demethylation activities need to be locally recruited and formed. Recent evidence shows that ncRNAs play a key role in regulating DNA methylation patterns in a locus-specific fashion. Antisense long ncRNA upregulates TCF21 gene expression with low DNA methylation of the promoter. The long ncRNA sequence is complementary to that of the TCF21 promoter, and interacts with both the TCF21 promoter and GADD45A that recruits TET and TDG for base excision and repair (Arab et al. 2014). Another example is that of the DNMT1-interacting long ncRNAs. These ncRNAs arise from corresponding gene-specific loci and act as a shield, halting DNMT1 and thus preventing DNA methylation at their site of transcription (Di Ruscio et al. 2013).
DNMT1 maintains DNA methylation patterns by methylating the opposite DNA strand of de novo hemi-methylated CpG dinucleotides (Fig. 1b). Once double-stranded DNA methylation has been achieved, double-stranded demethylation would be resistant to erasure (James et al. 2003). Blocking gene-specific DNA methylation by DNMT1-interacting long ncRNAs may be required to establish and maintain low DNA methylation after stimulation removal (sustained DNA demethylation, Fig. 5b). Conversely, genomic loci lacking expression of DNMT1-interacting long ncRNAs may maintain high DNA methylation after suppression removal (sustained DNA methylation, Fig. 5d). The presence or absence of DNMT1-interacting long ncRNAs expression may explain four types of gene-specific changes in DNA methylation patterns upon stimulation or suppression.
7 Intrauterine Programming and Hypertension
A maternal diet low in protein increases the susceptibility of offspring to hypertension. The Dutch famine cohort shows that children from mothers with insufficient protein intake, in relation to carbohydrate intake, during the third trimester of pregnancy had higher blood pressure in adulthood (Roseboom et al. 1999, 2001a). In addition, the famine is associated with glucose intolerance, obesity, and elevated serum cholesterol in later life (Roseboom et al. 2001b). Reduced representation bisulfite sequencing (RRBS) analysis, that covers only 5–10 % of all genomic CpG dinucleotides, was used to identify the Dutch famine-associated changes in DNA methylation in genes that could be responsible for the adverse metabolic profile (Heijmans et al. 2008; Tobi et al. 2014). More comprehensive analyses, such as whole genome bisulfite sequencing (WGBS) (that covers >70 % of human genomic CpG dinucleotides), is likely to identify changes in the methylation of genes involved in the development of hypertension.
Maternal low protein diets in rat (Kwong et al. 2000) and mouse (Watkins et al. 2008) programs offspring to develop hypertension. In rats, offspring of mothers fed a low protein diet develop hypertension with decreased DNA methylation and subsequent increased expression of the type 1b angiotensin II receptor gene (Agtr1b) in the adrenal gland (Bogdarina et al. 2007). These changes, involved in developing hypertension, are seen very early in life and last until at least 12 weeks of age, suggestive of a sustained type of DNA demethylation. In contrast, angiotensinogen in the liver shows increased expression at 1 week of age, and is normalized by 12 weeks. Despite lacking data on DNA methylation, it is predicted that Agt will show a returned type of DNA demethylation. Similar findings of increased Agtr1 expression have been reported in sheep following a maternal undernutrition diet (Whorwood et al. 2001).
When maternal glucocorticoid synthesis is pharmacologically blocked during the first two weeks of pregnancy, the maternal low protein diet-associated hypertension in the offspring is ameliorated. This is associated with a concomitant normalization of gene expression and DNA methylation of Agtr1b in the rat adrenal gland (Bogdarina et al. 2010), suggesting that the maternal hypothalamic-pituitary-adrenal axis plays a central role in the development of hypertension associated with prenatal exposure to a maternal low protein diet.
8 Acquired Predisposition and Hypertension
Tissue DNA methylation patterns are of physiological importance to constitution. One human study evaluated the association between DNA methylation and acquired predisposition for hypertension (Wang et al. 2014a). Visceral adipose tissue (VAT) surrounding cortisol-producing adenoma (Cushing’s syndrome, CS), aldosterone-producing adenoma (APA), and non-functioning adenoma (NFA) were examined. Both cortisol and aldosterone were found to have the ability to stimulate VAT AGT transcription, with accompanying AGT promoter DNA demethylation. Cortisol and aldosterone exert differential effects through binding and activation of a subfamily of NR3C glucocorticoid receptors (GRs) (NR3C1) and mineralocorticoid receptors (MRs) (NR3C2), respectively. The sites of DNA demethylation at the AGT locus appear to be associated with GR or MR binding sites (Wang et al. 2014a; Demura et al. 2015). This study demonstrates that disease conditions such as CS and APA cause acquired predisposition to susceptibility to hypertension through DNA demethylation of VAT AGT. Surgical removal of the tumor does not always restore blood pressure to normal levels in CS and APA, suggesting that the DNA methylation patterns induced by excess circulating cortisol and aldosterone are not fully reversed. A genome-wide analysis of DNA methylation is necessary to elucidate whether changes in DNA methylation patterns are reversed or sustained in a locus-specific fashion following surgical treatment for CS and APA. Such analysis is likely to have a large impact on our understanding of the role of DNA methylation in hypertension.
High DNA methylation around a TSS is associated with the absence of RNA polymerase II. Conversely, low DNA methylation around a TSS is associated with the presence of either active (high transcription levels) or stalled (low transcription levels) RNA polymerase II (Takeshima et al. 2009). The combination of high promoter DNA methylation and intermediate DNA methylation around the TSS of VAT AGT in NFA reflects suppression of VAT AGT transcription (Wang et al. 2014a; Demura et al. 2015). Excessive salt in contemporary diets reduces circulating RAAS levels (Oliver et al. 1975). Collectively, although VAT would be a major site of AGT production, high promoter DNA methylation of VAT AGT in NFA is considered to be adaptive response against an overabundance of salt in contemporary society.
Salt is a well-recognized risk factor for the development of hypertension. In rats, high-salt diets suppresses the level of circulating RAAS, yet VAT Agt expression is increased and accompanied by low Agt promoter methylation (Wang et al. 2014a; Demura et al. 2015). Using RRBS analysis, exposure to a high-salt diet for just 7 days induces significant changes in DNA methylation and hydroxymethylation patterns in the renal outer medulla (Liu et al. 2014).
As described, concrete, although limited, examples are available that demonstrate that disease conditions and dietary factors can induce an acquired predisposition to hypertension. Further insights into the relationship between DNA methylation and hypertension will be gleaned from additional studies in this area.
9 Transgenerational Epigenetic Inheritance and Hypertension
Whilst several animal studies have illustrated transgenerational inheritance, there is currently no direct and definitive evidence showing transgenerational epigenetic inheritance in hypertension. In addition to their target organs, environmental factors and disease conditions can cause changes in DNA methylation patterns in all tissues including germ cells. Changes in germ cells are less apparent than those that occur in target tissues (Dias and Ressler 2014). From an evolutionary perspective, this system would provide the ability to adapt to environmental circumstances and aid in preservation of the species.
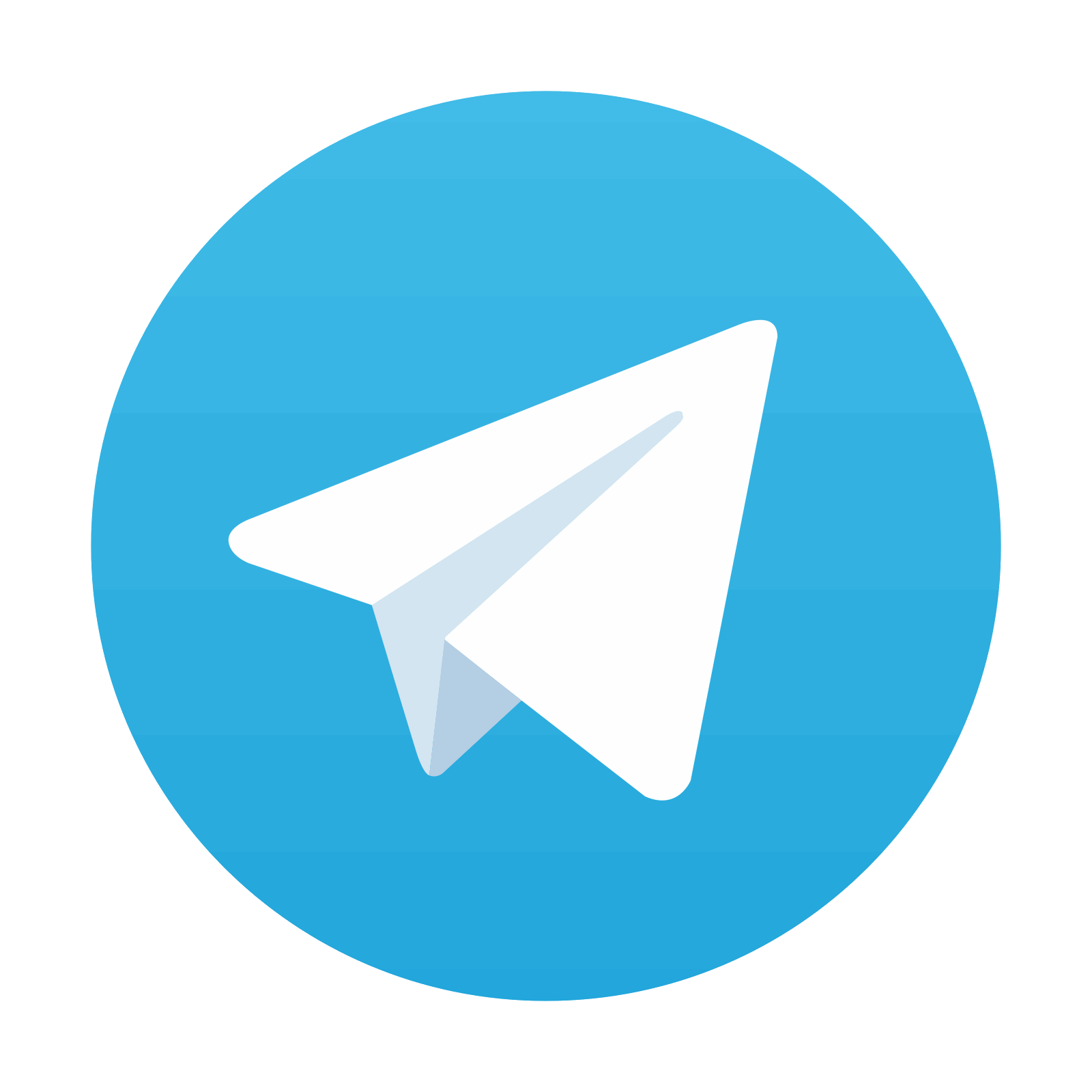
Stay updated, free articles. Join our Telegram channel

Full access? Get Clinical Tree
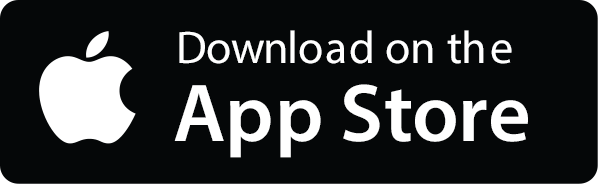
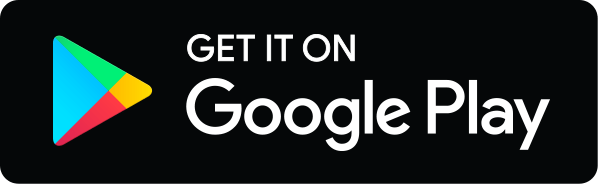