The first report of minimally invasive esophagectomy (MIE) appeared in the early 1990s. Whether thoracoscopic, laparoscopic, transhiatal, or combined, MIE evolved as a result of several goals: to reduce thoracotomy-related chest wall discomfort and postoperative debility; to achieve a more frequent R0 rate and better lymphadenectomy; and to achieve superior local control compared with the MIE transhiatal esophagectomy.1,2 Robotic-assisted esophageal resection emerged onto the surgical literary scene in 2003 and 2004 with the transhiatal esophagectomy and 3-field esophagolymphadenectomy, respectively. The advantages of robotic technology that are brought to this procedure include multi-articulated instruments with 7 degrees of rotational freedom, referred to as the EndoWrist®, simulating normal wrist movements thus differentiating the robotic system from standard videoscopic techniques; and the three-dimensional (3D) imaging provided by the double optic system allowing depth perception that improves surgical precision. Subsequent case series over the last decade have accomplished many of these goals while conferring clinical advantages of minimally invasive surgery (MIS), thus paving a road for the different methods of the robotic-assisted esophagectomy.
Robotic esophagectomy has been performed for high-grade dysplasia, invasive carcinoma, and severe surgically failed esophageal dysfunction. Esophageal cancer is the most common indication, although not readily accepted by the esophageal surgical community. The nihilists believe the sole benefits of MIE are a new conduit for swallowing and some staging information, and that radiation and chemotherapy are critical for long-term survival. Others believe that resection not only provides palliation, but also reduces the likelihood of local–regional recurrence and, if performed correctly, will improve survival. Collaborative efforts have found that depth of esophageal wall penetration and location of tumor are important for determining the extent of lymphadenectomy. Key factors for surgical and clinical success must follow a few general rules: (1) achieve an R0 resection; (2) provide adequate staging by performing an extensive lymphadenectomy assisted by knowledge of the tumor location, cell type, as well as information from high-resolution computed tomogram (CT), FDG-positron emission tomography, and endoscopic ultrasound; and (3) minimize local–regional recurrence, postoperative pain, and procedural-related morbidity and mortality.
An open technique using wide resection can be compromised because of the lack of visibility inherent in a thoracotomy exposure and articulations necessary to perform a thorough lymphadenectomy. In addition, the significant torque on the chest wall required to obtain the best visibility increases the likelihood for postoperative discomfort. Aforementioned robotic advantages, we feel, solve limitations of prior approaches thus extending clinical benefits to the patient.
The preoperative evaluation is no different for robotic surgery than for the conventional open surgical procedure. The history and physical examination are integral components in the initial phases of surgical decision making. Further workup may include CXR, CT chest/abdomen, FDG-positron emission tomography, barium swallow, upper endoscopy, bronchoscopy, endoscopic ultrasound, pulmonary function testing, and cardiopulmonary exercise testing. We do not exclude patients based on BMI, ASA status, or age. Higher values on each of these measures actually may benefit from a minimally invasive approach rather than a thoracotomy. On the other hand, those patients with marginal pulmonary or cardiac reserve may have difficulty with single-lung ventilation and carbon dioxide insufflation, which are typically employed in robotic techniques. We place all of our patients in a preoperative physiotherapy program for conditioning.
There is little guidance in the literature regarding the planning and preparation necessary for an efficient thoracic robotic procedure. Robotic work, in the chest, even more than in the abdomen, requires additional planning, skills, and knowledge of the anatomy and an understanding of the limitations of the technology. Creation and maintenance of a skilled operating room robotically oriented team is absolutely imperative to facilitate communication and to minimize operating room time. The bedside surgical assistant provides key exposure, mobilization, and suctioning. An operating nurse and support nursing staff, as well as an experienced anesthesiologist, who can perform single-lung ventilation and hemodynamically support the patient, are critical to maintaining the flow of the procedure.
The rigidity of the chest wall and the movement of the heart, lungs, and mediastinum present technical challenges and specific techniques are used to optimize exposure. In general, for the chest portion, if that is the first portion of the procedure and if we are not planning an in-the-chest anastomosis, we place the patient in the prone and a slightly reverse Trendelenburg position. The weight of the mediastinal and lung structures fall toward gravity, exposing the normally less visible areas thus providing more room for dissection and countertraction.
We also introduce CO2 insufflation to distend the mediastinum creating more space for dissection. It also compresses the ipsilateral lung and diaphragm, helps to evacuate any smoke from the cautery or harmonic dissection device, and helps to keep the lens clean. We typically use a pressure of 5 to 15 mm Hg at a maximal flow rate, although we often start with low pressures and slowly advance the pressure after the patient has hemodynamically adjusted. For the chest, we most often use the 0-degree scope as it provides a better peripheral view, often a limitation in the chest, and we rarely need the angled vision afforded by the 30-degree scope.
Lower esophageal, periesophageal, and diaphragmatic procedures may be best performed transabdominally in a supine position. Robotic arm ports placed at varying distances beneath each costal margin and a periumbilically placed videoscope optimize the robotic transhiatal thoracic access. Through this approach, most procedures in the lower mediastinum can be performed; however, limitations of the current robotic arms and instruments prevent accessibility beyond the level of the mid-mediastinum. Therefore, for example, a transhiatal robotic esophagectomy with extensive lymphadenectomy would be very difficult to perform in most patients. These aspects will need to be addressed in the future to improve utility.
Like patient positioning, proper port placement is critical. The intended operative area should be drawn on the chest using the CT scan and surface landmarks as a guide. In the case of esophagectomy, the scope of visibility for the chest exposure is quite large, encompassing the entire cranial–caudal length of the thorax.
For the prone portion of the procedure, we place five ports in the chest, each about 10 to 15 cm apart, three of them in a line along the area of the esophagus toward the posterior axillary line with the central port view at the center of the esophagus (or the most critical part of the dissection), (Fig. 166-1). As a rule, the robotic chassis or bedside cart is brought in exactly opposite the viewing scope, keeping the pathology to be dissected or removed between the camera port and the base of the bedside robotic cart.
Additional ports are placed, as necessary, for a fourth robotic arm or accessory instruments to be used by the surgical assistant for retraction or dissection assistance. Occasionally, the robotic arms and instruments do not sufficiently reach the target and specific maneuvers may be necessary: inserting the trocars further into the chest; moving the trocar into a different intercostal space through the same skin incision; adding positive end-expiratory pressure to the opposing lung thereby pushing the mediastinum closer to the reach of the robotic instruments; and/or removing some of the CO2 pressure used during the procedure. For better exposure, the partially open fan retractor (US Surgical, Norwalk, CT) or the paddle retractor (US Surgical) are safe, and may provide sufficient retraction of the lung, the heart, and great vessels.
The first case reports of robotic esophagectomy were published in 20033 and 2004.4 Since that time there have been four different reports in the literature: (1) the chest only approach; (2) the robotically assisted transhiatal esophagectomy, initially coined the robot-assisted transhiatal esophagectomy (RATE) procedure; (3) the robotically assisted esophagolymphadenectomy, a modification of the three-hole or McKeown approach, termed the robot-assisted extended lymphadenoesophagectomy (RALE) procedure; and (4) the robotically assisted Ivor Lewis approach with an intrathoracic esophagogastrostomy, termed the robot-assisted Ivor Lewis esophagectomy (RILE) procedure. As with the MIE and the open thoracotomy approaches, there are many different steps and many different techniques used to perform each of those steps. Thus, the merits of the overall approach and the successes or failures should not be attributed to the robot or the general approach. Robotic surgery is still in its infancy and even more so with its use in esophagectomy and alimentary tract reconstruction.
The conventional open approach and the thoracoscopic MIS approach have shortcomings. The conventional open esophagectomy has a reported morbidity of 40% to 80%.5 The published institutions have an in-hospital mortality of approximately 5%.5 In the largest published VATS esophagectomy series, the major and minor morbidity was 32% and 55%, respectively, with 1.4% mortality.6 A recent comparative review article from the Netherlands demonstrated nicely the benefits of MIE versus open techniques. Calculated means showed a significant decrease in estimated blood loss (EBL), hospital and ICU stays, number of harvested lymph nodes, lower morbidity (especially pulmonary complications), and mortality (1.9% vs. 4.5%).7 A recent review compared nine robotic case series to the study by Verhage. This found even further improvement in the robotic group with respect to operating times (-6%), EBL (-22%), ICU stay (−42%), major complications (−45%), and lymph node retrieval (+27%).8 They also found comparative rates for robotic versus laparoscopic approaches in the length-of-hospital stay and mortality rates (15.6 vs. 15.9 days, 2.4% vs. 1.3%, respectively).
Critically important, the robotic approach has been shown to be oncologically sound and similar to that found in the open non-MIS esophagectomy cases. In a recent review article appraising the literature on robotic esophagectomy, R0 resections were noted in 76% to 100% of patients, the median lymph node retrieval was 14 to 38, and disease-specific recurrence rate was 14%.8 There is insufficient information to provide long-term survival in robotic esophagectomy patients; however, a few studies have reported >50% survival at >15 months.9–12 Currently, there is insufficient data to make any conclusions, but as a tool, robotics can potentially offer as rigorously sound a cancer operation as the more standard open procedures.
Tables 166-1 and 166-2 highlight the relevant case reports and series in tabular format.6–19 It summarizes each according to the technique type and various metrics related to their preoperative, intraoperative data, and surgical outcomes. As was shown in multiple series, the Dutch group, for example, noted that their complication rate was initially high, approximately 60%, which subsequently improved after they gained experience. As with the open technique, the most common complication was pulmonary-related albeit abated. The location and histology type of the primary lesion was most commonly in the lower third of the esophagus and adenocarcinoma, respectively. Neoadjuvant therapy was given at variable rates, according to institutional protocol. Conversion rates were low (mean 4%, range 0%–15%) and were mainly related to difficulties with adhesions or bleeding. The median operating room time varied relating to surgeon experience and technique: chest only averaged 6½ hours, RATE averaged 4¾ hours, RALE averaged 11 hours, and RILE averaged 9 hours, but this represents a very early experience and most surgeons are reporting shorter times with greater experience. The higher later two times attributed to the inherently more complex total robotic esophagectomy approach. The median intraoperative blood loss also varied relating to technique: chest only averaged 380 mL, RATE averaged 75 mL, RALE averaged 588 mL, and RILE averaged 400 mL. Obviously, these are very rough estimates as data variability within the case series limit true averages. The R0 rate for the series was >76% and often as high as 100% in many series. The average number of lymph nodes retrieved and/or examined was 21 among the studies. The anastomosis was mostly performed in the neck (only a few RILE patients) and the leak rate was reportedly as high as 33% and as low as 0% with an average of 17%. Regarding the anastomosis, it was quite surgeon or institution specific; most performed either a side-to-side linear stapling technique20 or a circular stapling technique. Still, advocates of a hand-sewn technique report lower leak rates than the average.19 For the group overall, the average length-of-hospital stay and perioperative 30-day mortality was 11.75 days and 1.3%, respectively.
AUTHOR | YEAR | TECHNIQUE | n | ADENO (%) | DISTAL OR GEI (%) | NEOADJ (%) | STAGE | ABD LN DISSECTION | THORACIC LN DISSECTION | PYLORUS INTERVENTION | LOCATION OF ANASTOMOSIS | SPECIMEN EXTRACTION | ANASTOMOSIS METHOD | |
Horgan USA | 2003 | RATE | 1 | 100 | 100 | 0 | 1 | D1 | Sampling | None | Cervical | Neck | 2-layer | |
Kernstine USA | 2004 | RALE | 1 | 100 | 100 | 100 | 2b | D2 | MLND | None | Cervical | Neck | Linear stapler | |
Bodner Austria | 2005 | Chest only | 4 | 25 | 75 | NR | 1b–3a | NR | NR | NR | Cervical | Neck | NR | |
Van Hillegersberg The Netherlands | 2006 | Chest only | 21 | 48 | 62 | 14 | 1–4 a | D1 Left gastric | MLND | NR | Cervical | Abdomen | 1-layer | |
Anderson USA | 2007 | Chest only (n = 22) RATE (n = 1) Abd Only (n = 2) | 25 | NR | 100 | 68 | NR | D2 | MLND | None | Neck Neck Chest | Neck Neck Chest | Linear stapler | |
Kernstine USA | 2007 | Chest only (n = 6) RALE (n = 8) | 14 | 57 | 71 | 64 | 2b–4 | D2 | MLND | None | Chest | Neck | Linear stapler | |
Kernstinea USA | 2008 | RILE | 39 | NR | NR | NR | NR | D1 | MLND | Botulinum? | Cervical selective chest | Neck | Linear stapler | |
Galvani USA | 2008 | RATE | 18 | 100 | NR | NR | 0–2 a | D1 | Sampling | None | Cervical | Neck | Linear stapler, 2-layer | |
Boone The Netherlands | 2009 | Chest only | 47 | 61 | 74 | 6 | 1–4b | D1 Left gastric | MLND | NR | Cervical | Abdomen | 1-layer | |
Sutherland USA | 2010 | RATE | 36 | 88 | NR | 38 | NR | NR | Sampling | NR | Cervical | Neck | Circular stapler | |
Kim South Korea | 2010 | Chest only RALE (n = 4) | 21 | 5 | 52 | 14 | 1–4 | D1 | MLND | None | Cervical | Neck | Stapled: 19 linear, 2 circular | |
Clark (meta-analysis) England | 2010 | Chest only RATE | 130 | NR | 70 | NR | 1–4 | NR | NR | NR | Both | Neck | Both | |
Weksler USA | 2012 | Chest only | 11 | 90 | NR | 37 | 0–3 | D1 | MLND | Pyloroplasty | Cervical | Abdomen | Stapled | |
Cenfolio USA | 2012 | Chest only | 22 | 82 | NR | 91 | 1–3 c | D1 | Left gastric | MLND | Botulinum | Chest | Chest | 2-layer (73%) Stapled (27%) |
Dunn USA | 2012 | RATE | 40 | 90 | 92.5 | 42 | 1–4 | D1 | Left gastric | MLND | Pyloroplasty | Cervical | Neck | Circular stapler |
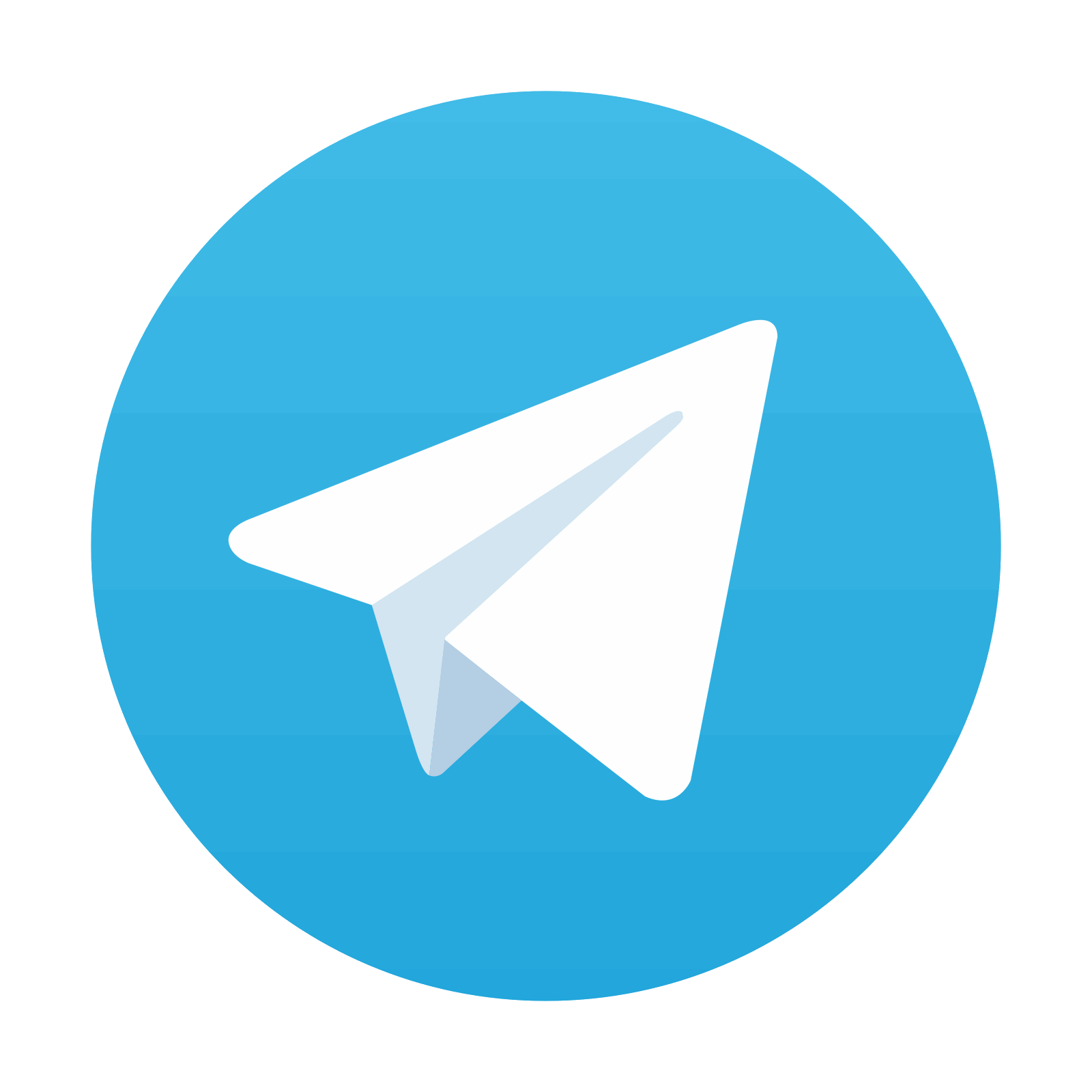
Stay updated, free articles. Join our Telegram channel

Full access? Get Clinical Tree
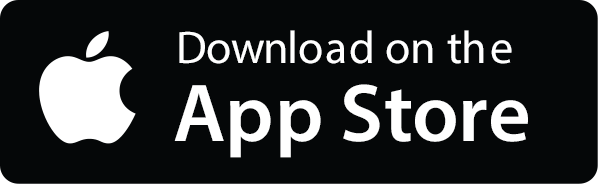
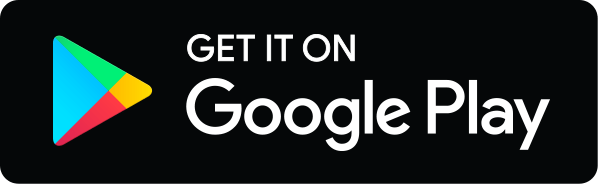
