Background
Structural right ventricular (RV) abnormalities are present in a substantial proportion of patients with hypertrophic cardiomyopathy (HCM), but the trigger for RV hypertrophy remains unclear. The aim of this study was to assess the relationship between RV and left ventricular (LV) remodeling and the impact of biventricular involvement on clinical status in this setting.
Methods
Ninety-nine patients with HCM and 30 normal subjects with a similar age and gender distribution were prospectively enrolled. Comprehensive echocardiography was performed in all, including the assessment of LV and RV function by tissue Doppler and speckle-tracking echocardiography. Measurement of RV free wall thickness (RVWT) was performed at end-diastole, in a zoomed subcostal view, focusing on the RV midwall.
Results
Patients with HCM had increased RVWT (6.4 ± 1.9 vs 3.6 ± 0.8 mm, P < .001) and lower values of RV global longitudinal strain (−19.4 ± 4.4% vs −23.8 ± 2.7%, P < .001) compared with control subjects. RVWT was independently related to LV mass and LV global longitudinal strain. Increased RVWT was correlated with New York Heart Association class ( r = 0.20, P = .04) and calculated sudden cardiac death risk score ( r = 0.52, P < .001) and was independently related to the presence of ventricular arrhythmias (odds ratio, 2.02; 95% CI, 1.28–3.19; P = .002).
Conclusions
In patients with HCM, the presence of RV hypertrophy was associated with increased LV mass and reduced LV longitudinal strain, correlated with increased calculated sudden cardiac death risk score, and independently related to the presence of ventricular arrhythmias. These data suggest more severe disease in patients with biventricular HCM.
Hypertrophic cardiomyopathy (HCM) is the most common genetic heart disease, characterized by great morphologic, functional, and clinical heterogeneity. Myocardial hypertrophy, the classical phenotypic hallmark of this condition, can be found at any location, including the right ventricle. Structural right ventricular (RV) abnormalities, present in a substantial proportion of patients with HCM, can be a consequence of the sarcomeric protein genetic mutations, afterload changes, or ventricular interdependence, but the main trigger remains unclear. Few data are presently available about the relationship between left- and right-heart remodeling in this setting.
In a close interdependence with morphologic changes, RV dysfunction can be an important marker of RV involvement in the myopathic process that characterizes HCM. However, because of the right ventricle’s complex shape and high load dependency, the accurate and reproducible assessment of RV function is still difficult, and the available data are limited. Several studies performed in patients with HCM have demonstrated RV diastolic and systolic dysfunction, as assessed by conventional parameters such as tricuspid annular plane systolic excursion (TAPSE), RV fractional area change (FAC), and RV myocardial performance index. On the other hand, the assessment of myocardial deformation by two-dimensional (2D) speckle-tracking echocardiography (STE) could provide a more accurate quantification of regional RV function and potentially additional information on clinical impact in this setting.
Changes in the morphology, structure, and function of the myocardium are responsible for the main clinical features of this disease: heart failure and arrhythmias. Although a large body of evidence supports the impact of left ventricular (LV) remodeling on the clinical status, there are few data regarding the relationship between RV remodeling and the clinical expression of the disease.
We hypothesized that in patients with HCM, (1) RV remodeling is a frequent finding, closely interrelated with LV remodeling, and (2) heart failure symptoms and arrhythmias are related to RV remodeling.
Methods
Study Population
We prospectively screened for enrollment 166 consecutive patients referred to our echocardiography laboratory between April 2009 and February 2015 who met the diagnostic criteria for HCM: M-mode and 2D echocardiographic evidence of wall thickness ≥ 15 mm in one or more LV myocardial segments in the absence of exercise training history and of cardiac or systemic conditions capable of inducing that magnitude of hypertrophy. Patients with concomitant hypertension were included in the study population only if they had clear features favoring the diagnosis of HCM: family history of HCM, RVH, maximal LV wall thickness (LVWT) ≥ 15 mm, severe LV diastolic dysfunction, or marked repolarization abnormalities on electrocardiography.
Patients with poor acoustic window ( n = 10), those who were technically unsuitable for Speckle-tracking echocardiographic analysis ( n = 10), and those with nonsinus rhythm ( n = 20 patients with atrial fibrillation and n = 4 patients with atrial flutter), valvular prostheses ( n = 4), pacemakers ( n = 8), LV segmental wall motion abnormalities ( n = 3), endocarditis ( n = 2), and suspected amyloidosis ( n = 6) were excluded.
Patients suspected on the basis of common clinical and laboratory data (electrocardiography, standard biology) of having causes of LV hypertrophy other than sarcomeric gene mutation and those with significant valvular heart disease other than mitral regurgitation were not eligible.
The final study population consisted of 99 patients.
Thirty healthy volunteers with a similar age and gender distribution served as a control group. They had no evidence of heart disease by physical examination, 12-lead electrocardiography, and echocardiography, and they were taking no medications. All subjects gave informed consent to participate in the study.
Clinical Data
The following clinical data were collected: age, gender, history of smoking, hypertension (defined as a history of hypertension requiring medical therapy), diabetes mellitus, and hypercholesterolemia. A family history of HCM or sudden death and the presence of chest pain, dyspnea, or syncope were also assessed. Clinical status was defined according to the New York Heart Association (NYHA) functional classification. In 91 of the 99 patients, 24-hour ambulatory electrocardiographic (ECG) monitoring using a Schiller-type Holter monitor was performed within 1 month of the echocardiographic study. The following arrhythmias were assessed: ventricular tachycardia (defined as three or more consecutive ventricular premature beats at a rate of ≥120 beats/min) and paroxysmal atrial fibrillation (defined as at least one period of >30 sec in duration of an absolute arrhythmia without detectable P waves and without a pattern consistent with an alternative diagnosis). The 5-year risk for sudden cardiac death (SCD) was estimated using the HCM Risk-SCD model. Routine laboratory testing, including renal function testing, was performed in 90 hospitalized patients. Information regarding current medications was also obtained.
Echocardiography
A commercially available ultrasound machine (Vivid 7 or Vivid E9, GE Vingmed Ultrasound AS, Horten, Norway) equipped with an M4S probe was used for all echocardiographic examinations. Standard echocardiographic views were obtained using second-harmonic imaging with frequency, depth, and sector width adjusted for frame-rate optimization (60–100 frames/sec). Image settings and frame rates were kept similar for LV four-chamber, two-chamber, and long-axis apical views, which were recorded immediately one after another. LV diameters, the thicknesses of the interventricular septum and LV posterior wall, and left atrial (LA) anteroposterior diameter were measured according to guideline recommendations. The maximal diastolic LVWT was measured, using 2D short-axis views in all LV segments, from base to apex. LV volumes and ejection fraction were calculated using the Simpson biplane method. LV mass was calculated using the equation of Devereux. All volumes and LV mass were normalized to body surface area. Basal RV diameter and minor axis of the right atrium were measured in the apical four-chamber view. The RV wall was visualized from different echocardiographic views ( Figure 1 ) and was carefully assessed in motion in order to identify the maximal wall thickness, excluding epicardial fat or ventricular trabeculae. Measurement of RV free wall thickness (RVWT) was performed at end-diastole, below the tricuspid annulus at a distance approximating the length of the anterior tricuspid leaflet when it is fully open, in a zoomed subcostal view with focus on the RV midwall. In patients with inadequate subcostal windows, measurement was performed in left parasternal windows. RVWT > 5 mm was used to define the presence of RVH. TAPSE was measured as an index of longitudinal systolic RV function between end-diastole and peak systole by M-mode echocardiography with the cursor aligned along the direction of the tricuspid lateral annular motion in the apical four-chamber view. RV FAC was calculated as recommended, providing an estimate of global RV systolic function. Peak systolic (S) and peak early diastolic (e′) mitral annular velocities were obtained by pulsed-wave tissue Doppler imaging from the apical four-chamber view using the septal and the lateral sites. The average between septal and lateral mitral e′ was used to calculate the ratio of peak early diastolic transmitral flow velocity E to e′, to estimate LV filling pressures. Peak systolic velocity of the tricuspid annulus (RV S velocity) was obtained in the view that achieved parallel alignment of the Doppler beam with the RV free wall. LV outflow tract gradient was measured by continuous-wave Doppler from the apical five-chamber view. LV outflow tract obstruction was defined as a peak gradient > 30 mm Hg at rest or during the Valsalva maneuver. Color Doppler echocardiography was used for the semiquantitative assessment of mitral regurgitation severity, as recommended. Systolic pulmonary artery pressure (sPAP) was estimated using the peak velocity of the tricuspid regurgitation jet, as recommended.

Both 2D and Doppler images were digitally stored as three consecutive cycles recorded during end-expiratory apnea. All echocardiographic data were analyzed offline using a commercially available software package (EchoPAC PC version BT12; GE Medical Systems, Milwaukee, WI) by a single observer (M.R.) experienced in 2D strain quantitation by STE.
Measurement of LV and RV Strain
Analysis of longitudinal LV strain by STE was performed in the four-chamber, two-chamber, and long-axis apical views, as previously described. LV global longitudinal peak systolic strain (GLS) was calculated using a 17-segment model. Analysis of longitudinal RV strain by STE was performed in the RV-focused four-chamber view. Briefly, after manually tracing the RV endocardium, an automatically generated region of interest divided into six segments (three for the RV free wall and three for the right part of the interventricular septum) was provided, which could be adjusted by contour position refinements and width tuning to fit the RV wall. RV segments with inadequate image quality were rejected by the software. RV GLS values, calculated using a six-segment model, were validated by the software in 80 patients (80% feasibility). Longitudinal strain of the RV free wall (RVWε) and of the interventricular septum, respectively, were also reported separately as the average of deformation in the three RV free wall segments and in the three septal segments.
Statistical Analysis
The normal probability distribution was tested using the Kolmogorov-Smirnov test. Continuous data are presented as mean ± SD and nonparametric data as medians and ranges. Variables were compared using Student’s t test, the Mann-Whitney U test, analysis of variance, the Kruskal-Wallis test, or the χ 2 test as appropriate. The relationships between different parameters were assessed by correlation analysis: Pearson’s method for continuous, normally distributed variables and Spearman’s ρ method for ordinal or continuous but skewed variables. To identify significant independent correlates of RV functional parameters or RVH, variables with P values < .20 in univariate analyses were included in a multivariate model.
To identify the ability of RVWT to distinguish patients with from those without nonsustained ventricular tachycardia (NSVT), receiver operating characteristic curve analysis was used, and the respective area under the curve was calculated. Predictors of arrhythmias in patients with HCM were assessed using binary logistic analysis. All statistical analyses were performed using SPSS version 14.0 for Windows (SPSS, Inc, Chicago, IL). Two-sided P values < .05 were considered to indicate statistical significance.
Measurement variability was assessed for RVWT and for RV strain parameters in a randomly selected group of 15 patients with HCM. For interobserver variability, measurements were carried out by a second operator (A.C.) on previously acquired images. For intraobserver variability, two sets of measurements were carried out by the same operator (M.R.) 1 month apart. Variability was calculated as the absolute differences between two measurements divided by the mean of the two measurements.
Results
Study Participants
The demographic and echocardiographic characteristics of the study population are presented in Tables 1 and 2 . There were no significant differences between patients and control subjects with respect to age, gender, body surface area, or heart rate ( P > .05 for all). All 90 hospitalized patients had creatinine levels in the normal range, except for two patients, one of whom had significant apical hypertrophy and the other obstructive HCM, both with ECG abnormalities highly suggestive of HCM. The remaining nine outpatients were asymptomatic, without any clinical features or ECG changes suggestive of amyloidosis. LV outflow tract obstruction was present in 54 patients (46 with asymmetric LV hypertrophy and eight with symmetric hypertrophy). The remaining 45 patients without LV outflow tract obstruction had asymmetric LV hypertrophy (24 patients), apical LV hypertrophy (16 patients), and symmetric hypertrophy (five patients). No patient had RV obstruction (intracavitary or at the level of the RV outflow tract). Ninety-two patients had mitral regurgitation (grade 1 in 36, grade 2 in 33, and grade 3 in 23). As expected, patients with HCM had higher indexed LV masses, indexed LA volumes, and E/e′ ratios and lower S-wave velocities at both lateral and septal sites compared with control subjects ( P < .001 for all). LV global longitudinal function (GLS) was severely reduced in patients with HCM, despite their slightly higher LV ejection fractions. Twenty-three patients had angina, and 13 patients had syncope ( Table 2 ). Twenty-seven patients were free of heart failure symptoms (NYHA class I), and 72 patients were symptomatic (NYHA class II in 43, class III in 27, and class IV in two patients). During ambulatory ECG monitoring, 23 patients had paroxysmal atrial fibrillation, and 15 patients had NSVT.
Variable | Controls ( n = 30) | Patients with HCM ( n = 99) | P |
---|---|---|---|
Age (y) | 48 ± 9 | 53 ± 17 | .11 |
Men | 12 (40%) | 45 (45%) | .59 |
Body mass index (kg/m 2 ) | 25 ± 3 | 28 ± 5 | .01 |
LV parameters | |||
LV mass index (g/m 2 ) | 86 ± 14 | 174 ± 60 | <.001 |
LV EDVi (mL/m 2 ) | 50 ± 10 | 42 ± 14 | .006 |
LV ESVi (mL/m 2 ) | 20 ± 5 | 15 ± 6 | <.001 |
LV ejection fraction (%) | 61 ± 4 | 65 ± 7 | .001 |
Septal S velocity (cm/sec) | 7.9 ± 1.1 | 5.7 ± 1.7 | <.001 |
Lateral S velocity (cm/sec) | 9.5 ± 1.7 | 6.0 ± 1.8 | <.001 |
Mean e′ velocity (cm/sec) | 12.3 ± 1.9 | 5.7 ± 2.1 | <.001 |
E/e′ ratio | 6.2 ± 1.4 | 16.1 ± 6.9 | <.001 |
LV GLS (%) | −20.5 ± 1.8 | −13.9 ± 3.2 | <.001 |
Right-heart parameters | |||
RAD (mm) | 33 ± 3 | 35 ± 5 | .05 |
RVD (mm) | 30 ± 3 | 31 ± 5 | .62 |
RVWT (mm) | 3.6 ± 0.8 | 6.4 ± 1.9 | <.001 |
TAPSE (mm) | 23.8 ± 3.1 | 23.8 ± 3.4 | .96 |
RV FAC (%) | 47.5 ± 7.1 | 47.6 ± 7.8 | .94 |
RV S velocity (cm/sec) | 14.2 ± 2.4 | 13.4 ± 3.1 | .18 |
RV GLS (%) | −23.8 ± 2.7 | −19.4 ± 4.4 | <.001 |
IVSε (%) | −18.9 ± 2.2 | −12.3 ± 4.1 | <.001 |
RVWε (%) | −28.3 ± 3.7 | −25.0 ± 5.5 | .004 |
LAVi (mL/m 2 ) | 29 ± 6 | 60 ± 26 | <.001 |
sPAP (mm Hg) | 23.2 ± 2.9 | 35.3 ± 11.7 | <.001 |
Variable | Patients without RVH ( n = 46) | Patients with RVH (n = 53) | P |
---|---|---|---|
Age (y) | 52 ± 17 | 53 ± 16 | .86 |
Men | 20 (43%) | 25 (47%) | .71 |
Body mass index (kg/m 2 ) | 28 ± 5 | 27 ± 5 | .53 |
SBP (mm Hg) | 135 ± 22 | 125 ± 23 | .02 |
Symptoms | |||
Angina | 12 (26%) | 11 (21%) | .53 |
Syncope | 5 (11%) | 8 (15%) | .53 |
NYHA class (I/II/III/IV) | 15/21/10/0 | 12/22/17/2 | .29 |
Atrial fibrillation | 7 (15%) | 16 (30%) | .11 |
NSVT | 3 (7%) | 12 (23%) | .02 |
Cardiovascular risk factors | |||
Hypertension | 25 (54%) | 23 (43%) | .27 |
Dyslipidemia | 31 (82%) | 32 (73%) | .34 |
Diabetes mellitus | 10 (22%) | 4 (7%) | .04 |
Smoker | 7 (15%) | 11 (21%) | .47 |
Creatinine (mg/dL) | 0.81 ± 0.35 | 0.92 ± 0.24 | .09 |
Medications | |||
β-blockers | 43 (93%) | 47 (89%) | .40 |
Calcium channel blockers | 4 (9%) | 6 (11%) | .66 |
ACE inhibitors | 18 (39%) | 16 (30%) | .65 |
Amiodarone | 6 (13%) | 13 (25%) | .14 |
ICD | 2 (4%) | 4 (7%) | .50 |
RV Remodeling in Patients with HCM
RVWT was significantly higher and RV strain parameters (GLS and RVWε) were significantly lower in patients with HCM compared with normal subjects ( Table 1 ). There were no significant differences between patients and control subjects with respect to TAPSE, RV FAC, and RV S velocity ( P > .05 for all). Fifty-three patients had RVH (RVWT > 5 mm). Patients with RVH had significantly lower values for LV GLS, RV GLS, RVWε, and RV S velocity ( Figure 2 ) and higher values for E/e′ ratio and LV mass than patients without RVH ( Table 3 ). There were no significant differences between patients with and without RVH regarding age, gender, body mass index, heart rate, TAPSE, RV FAC, sPAP, the presence and severity of LV outflow tract obstruction, or mitral regurgitation severity ( P > .05 for all) ( Table 3 ). The prevalence of dyslipidemia (data available in 82 patients), hypertension, and smoking status were similar in patients with and without RVH ( Table 2 ). A larger number of patients without RVH had diabetes mellitus ( P = .04). There were no significant differences between patients with and without RVH regarding treatment with β-blockers ( P = .40), calcium channel blockers ( P = .66), or angiotensin-converting enzyme inhibitors ( P = .35). Nineteen patients were on antiarrhythmic medication (amiodarone) for supraventricular or ventricular arrhythmias. There was no significant difference between patients with and without RVH regarding the use of amiodarone ( P = .14).

Variable | Controls ( n = 30) | Patients without RVH ( n = 46) | Patients with RVH ( n = 53) | P |
---|---|---|---|---|
LV parameters | ||||
LV mass index (g/m 2 ) | 86 ± 14 | 152 ± 55 ∗ | 194 ± 57 ∗† | <.001 |
LV EDVi (mL/m 2 ) | 50.0 ± 10 | 41 ± 11 ∗ | 43 ± 16 | .01 |
LV ESVi (mL/m 2 ) | 20 ± 5 | 14 ± 6 ∗ | 15 ± 6 ∗ | <.001 |
LVWT (mm) | 9.3 ± 0.9 | 19.4 ± 4.5 ∗ | 21.5 ± 4.4 ∗† | <.001 |
LV ejection fraction (%) | 61 ± 4 | 66 ± 7 ∗ | 64 ± 6 ∗ | .001 |
Septal S velocity (cm/sec) | 7.9 ± 1.1 | 6.4 ± 1.7 ∗ | 5.1 ± 1.4 ∗† | <.001 |
Lateral S velocity (cm/sec) | 9.5 ± 1.7 | 6.9 ± 1.8 ∗ | 5.4 ± 1.5 ∗† | <.001 |
Mean e′ velocity (cm/sec) | 12.3 ± 1.9 | 6.7 ± 2.3 ∗ | 4.9 ± 2.1 ∗† | <.001 |
E/e′ ratio | 6.2 ± 1.4 | 13.5 ± 5.3 ∗ | 18.1 ± 7.4 ∗† | <.001 |
LV GLS (%) | −20.5 ± 1.8 | −15.4 ± 3.0 ∗ | −12.7 ± 2.9 ∗† | <.001 |
Right-heart parameters | ||||
RAD (mm) | 33 ± 3 | 35 ± 5 | 35 ± 6 | .12 |
RVD (mm) | 30 ± 3 | 31 ± 6 | 30 ± 4 | .71 |
RVWT (mm) | 3.6 ± 0.8 | 4.8 ± 0.34 ∗ | 7.8 ± 1.6 ∗† | <.001 |
TAPSE (mm) | 23.8 ± 3.1 | 24.3 ± 3.3 | 23.3 ± 3.5 | .32 |
RV FAC (%) | 47.5 ± 7.1 | 47.5 ± 6.7 | 47.7 ± 8.8 | .98 |
RV S velocity (cm/sec) | 14.2 ± 2.4 | 14.4 ± 3.2 | 12.4 ± 2.7 ∗† | .005 |
RV GLS (%) | −23.8 ± 2.7 | −22.4 ± 3.5 | −17.0 ± 3.6 ∗† | <.001 |
IVSε (%) | −18.9 ± 2.2 | −14.7 ± 3.2 ∗ | −10.3 ± 3.6 ∗† | <.001 |
RVWε (%) | −28.3 ± 3.7 | −28.4 ± 4.7 | −22.2 ± 4.7 ∗† | <.001 |
LAVi (mL/m 2 ) | 29 ± 6 | 56 ± 31 ∗ | 63 ± 21 ∗ | <.001 |
sPAP (mm Hg) | 23.2 ± 2.9 | 32.6 ± 7.3 ∗ | 37.6 ± 14.1 ∗ | <.001 |
LV outflow tract obstruction | — | 23 (50%) | 31 (58%) | .38 |
LV outflow tract gradient (mm Hg) | 89 ± 28 | 92 ± 31 | .75 | |
Mitral regurgitation degree (1/2/3) | — | 19/14/8 | 17/19/15 | .27 |
Intraobserver variability was 5.0 ± 4.0% for measuring RVWT, 6.5 ± 5.1% for measuring RV GLS, and 7.3 ± 5.4% for RVWε. Interobserver variability for the same parameters was 5.5 ± 4.3%, 6.3 ± 5.7%, and 8.1 ± 6.6%, respectively.
Relationship between RV and LV Remodeling in Patients with HCM
RVWT and Doppler tissue imaging or speckle-tracking echocardiographic RV functional parameters (RV S velocity, RV GLS, and RVWε) had moderate correlations with septal S velocity and LV GLS ( Figure 3 ) and a weak correlation with LV ejection fraction ( Table 4 ). RVWT and RV strain parameters were also related to LV mass, lateral S velocity, mean e′ velocity, and E/e′ ratio ( Table 4 ). All RV functional parameters were correlated with RVWT ( Table 4 ). Neither sPAP nor LV outflow gradient or mitral regurgitation severity was related to RV remodeling parameters ( P > .05 for all).

Variable | RVWT | RV S velocity | RV GLS | RVWε | ||||||||
---|---|---|---|---|---|---|---|---|---|---|---|---|
Univariate analysis | Multivariable analysis | Univariate analysis | Multivariable analysis | Univariate analysis | Multivariable analysis | Univariate analysis | Multivariable analysis | |||||
P | r | P | P | r | P | P | r | P | P | r | P | |
Age | .78 | — | .85 | — | .22 | — | .03 | 0.23 | .03 | |||
BMI | .67 | — | .99 | — | .92 | — | .41 | — | ||||
LV EDVi | .98 | — | .61 | — | .60 | — | .85 | — | ||||
LV ESVi | .20 | — | .58 | — | .71 | — | .45 | — | ||||
LVMi | <.001 | 0.45 | .02 | .89 | — | <.001 | 0.37 | .85 | .003 | 0.32 | .60 | |
Septal S | <.001 | −0.39 | — | <.001 | 0.55 | .05 | <.001 | −0.41 | — | .003 | −0.32 | — |
Lateral S | <.001 | −0.40 | — | .24 | — | <.001 | −0.40 | — | .002 | −0.35 | — | |
Mean e′ | <.001 | −0.38 | — | .42 | — | <.001 | −0.45 | — | <.001 | −0.44 | — | |
E/e′ ratio | .006 | 0.29 | .73 | .07 | .86 | .004 | 0.33 | .37 | .01 | 0.29 | .93 | |
LV EF | .03 | −0.21 | — | .02 | 0.26 | — | .02 | −0.25 | — | .01 | −0.27 | |
LV GLS | <.001 | 0.54 | <.001 | .003 | −0.33 | — | <.001 | 0.67 | <.001 | <.001 | 0.60 | .003 |
LAVi | .003 | 0.29 | .23 | .60 | — | .22 | — | .41 | — | |||
sPAP | .12 | .90 | .11 | .75 | .52 | — | .58 | — | ||||
LVOT gradient | .17 | .29 | .83 | — | .84 | — | .80 | — | ||||
MR degree | .10 | .46 | .11 | .33 | .07 | .50 | .14 | .84 | ||||
RVWT | — | — | .006 | −0.30 | .20 | <.001 | 0.61 | .006 | <.001 | 0.53 | .04 |
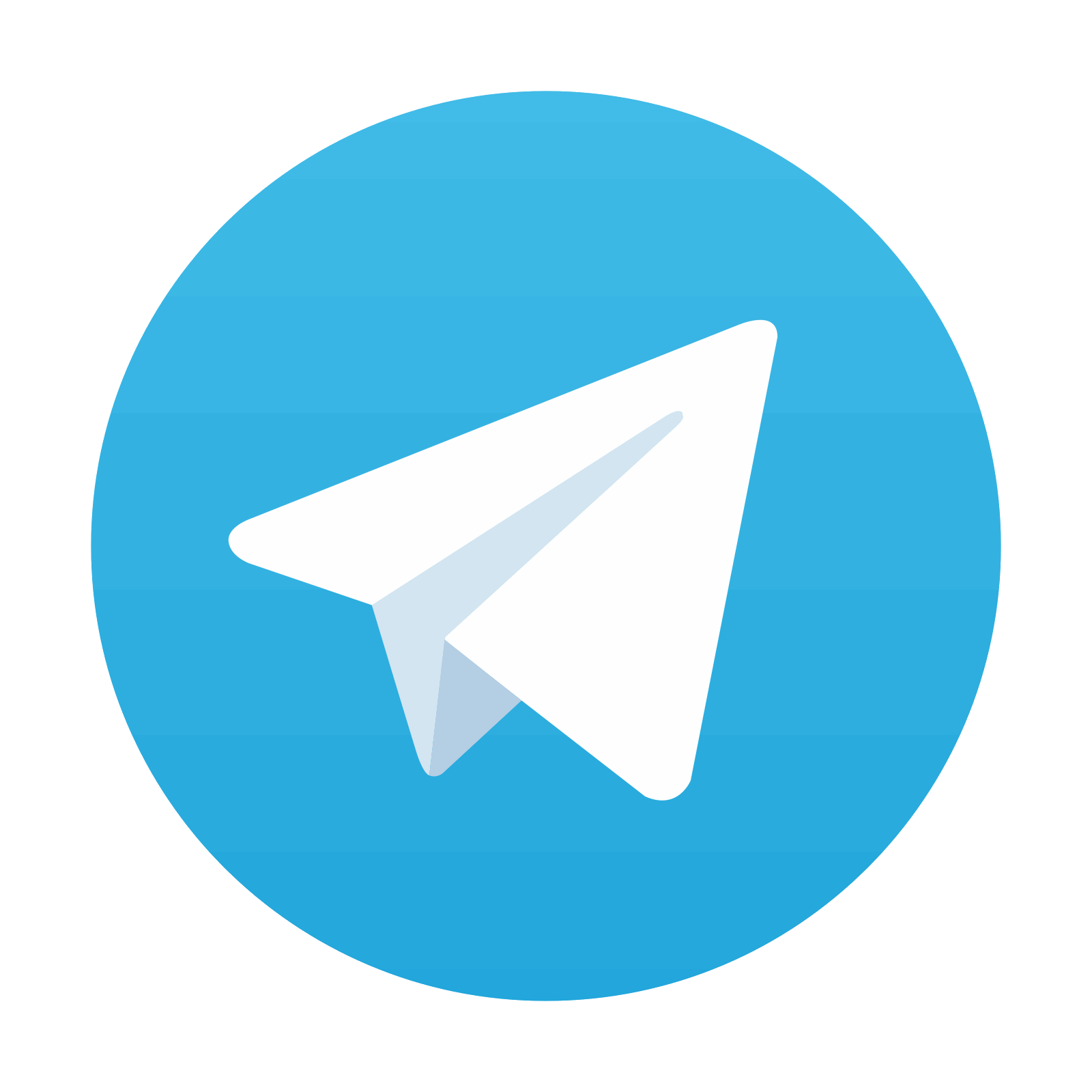
Stay updated, free articles. Join our Telegram channel

Full access? Get Clinical Tree
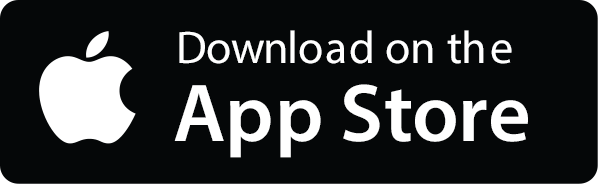
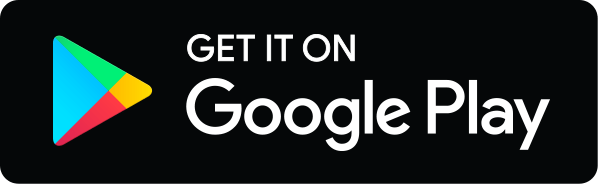
