Background
Although quantitative right ventricular (RV) strain analysis may be useful in congenital and acquired heart disease populations with RV failure, a comprehensive, standardized approach is lacking. An 18-segment RV strain analysis obtained from three standardized RV apical echocardiographic images was used to determine the feasibility, normal values, and reproducibility of the method in normal adults.
Methods
Forty healthy, prospectively enrolled volunteers with no cardiac histories and normal QRS durations underwent echocardiography optimized for strain analysis including three RV apical views. Two-dimensional speckle-tracking longitudinal strain analysis was performed using EchoPAC software. Eleven retrospectively identified subjects with RV disease were included as a pilot population. All had been imaged using the same protocol including the three RV apical views.
Results
All control subjects had normal anatomic morphology and function by echocardiography. Feasibility of the RV strain analysis was good (adequate tracking in 696 of 720 segments [97%]). RV global peak systolic strain was −23 ± 2%. Peak strain was highest in the RV free wall and lowest in the septum. Dyssynchrony indices demonstrated no dyssynchrony using left ventricular criteria. Reproducibility of most strain measures was acceptable. This methodology identified important disease not seen in the four-chamber apical view alone in the pilot population of 11 patients with RV disease. Strain patterns and values were different from those in the control population, indicating that differences do exist from normal.
Conclusions
Eighteen-segment RV strain analysis is feasible, with strain measures falling into discrete ranges in this normal population. Those with RV disease illustrate the potential utility of this approach. These data indicate that this model can be used for more detailed studies evaluating abnormal RV populations, in which its full potential can be assessed.
Two-dimensional (2D) speckle-tracking echocardiography is often used for the assessment of quantitative indices of global and regional left ventricular (LV) function by means of three standardized views, but no such multiple-view model is yet available for the right ventricle. The lack of a comprehensive right ventricular (RV) model may be due to multiple factors common in the right ventricle. These include limited target access induced by chest wall configuration in combination with the anterior retrosternal position of the right ventricle, a lack of standardized multiple RV views, and a lack of readily available RV strain analysis software.
LV strain analysis has enjoyed clinical success, in part because global and regional LV longitudinal strain is assessed in 18 segments from three standardized LV apical views. RV strain analysis, on the other hand, has been performed in only a few studies using six segments from one view (apical four chamber). To our knowledge, there are no studies using an 18-segment (18S) three-view RV strain analysis model. Early work during the development of this approach to RV strain analysis was performed in a systemic RV population (with D-transposition of the great arteries after atrial switch) at our institution and suggested the potential need for comprehensive image acquisition because of important findings seen outside of the four-chamber RV apical view (unpublished data).
Clinical RV functional assessment may be aided by a more detailed RV strain analysis that would be useful for the clinical management of patients with forms of congenital heart disease, pulmonary hypertension, RV infarction, and even LV assist devices. Furthermore, a comprehensive 18S RV model may also be useful for the determination of regional strain abnormalities resulting from activation delays (dyssynchrony) of the right ventricle, as is found in the left ventricle. This includes those with congenital heart disease and heart failure in a systemic or single right ventricle who might benefit from cardiac resynchronization therapy (CRT).
In this study, we evaluated feasibility, reproducibility, and RV regional variability of, and propose normal ranges for, an 18S acquisition and analysis model of RV longitudinal strain for the evaluation of global and regional RV mechanics in a healthy young adult population using currently available software. Such validation in a normal population is an important precursor to the application of this comprehensive RV model to patients with heart disease. Three patients (two congenital and one with pulmonary hypertension) from a pilot group of 11 patients with abnormal right ventricles are provided to demonstrate the potential for such a model and possibilities for further investigation.
Methods
Study Subjects
Complete transthoracic echocardiograms were obtained in 40 young, healthy, prospectively enrolled volunteer subjects, with the addition of three apical RV views optimized for strain analysis. All subjects underwent normal cardiac physical examinations. Inclusion criteria were a age ≥ 18 years at the time of the study, no history of cardiac abnormalities, normal echocardiographic findings including anatomy, LV ejection fraction ≥ 50%, fractional area change (FAC) ≥ 35%, and tricuspid annular plane systolic excursion (TAPSE) ≥ 16 mm. Exclusion criteria were any abnormal echocardiographic findings or prolonged QRS duration. Studies were obtained at Duke from March to July 2012. This study was approved by the Duke institutional review board, and all control subjects gave informed consent.
This study in the normal population was performed after noticing some regional abnormalities in patients with RV disease during routine clinical studies that prompted the development of the scanning methodology. The normal subjects were the main focus of this study.
Pilot Study Patients
An additional small pilot test population of 11 adult patients with abnormal RV function consequent to congenital or acquired disease was conducted to determine if any gross differences could be found in preliminary comparison with the normal population. These subjects were chosen from populations with RV pathology to illustrate the potential utility of the 18S model and CRT in diseased states but not to characterize abnormal populations in a comprehensive or quantitative manner. The pilot subjects were retrospectively identified for this study but had complete echocardiograms obtained with the three RV apical views.
All patients with abnormal findings had chronic elevations of RV pressure. The right ventricle was systemic in five subjects with D-transposition of the great artery (subaortic right ventricle consequent to atrial switch operations in infancy), and six had normal anatomy (subpulmonic right ventricle with acquired chronic pulmonary hypertension due to primary lung disease). All patients had New York Heart Association (NYHA) class II to IV symptoms requiring multiple heart failure medications. RV studies in the abnormal pilot group were obtained in the same fashion as in the normal population between June 2010 and July 2012.
Functional Echocardiography with Three Apical RV Views
All echocardiographic studies were acquired using a GE Vivid E9 imaging system using a 3.5-MHz ultrasound probe (GE Vingmed Ultrasound, Horten, Norway). Echocardiographic RV systolic function was assessed using the traditional markers of FAC and TAPSE measured according to the standard methodology on the four-chamber apical view in RV end-systole and end-diastole. LV systolic function was assessed using the LV ejection fraction with the biplane Simpson’s method.
Grayscale echocardiography was performed with images optimized for longitudinal 2D speckle-tracking strain analysis (50–90 frames/sec) from three apical RV views with the subject in the standard left lateral recumbent position. The three apical RV views were equivalent to the imaging planes of the two-chamber, three-chamber, and four-chamber LV apical views but with the transducer angled rightward ( Figure 1 ). In the early phase of this investigation, equivalent LV and RV imaging planes were ensured by starting from the LV view and simply angling rightward without changing the transducer position to obtain the RV view. However, view optimization of the “inflow” view often required repositioning of the transducer toward the left anterior axillary line, especially to image the anterior RV wall segments. Once RV landmarks were established, proper RV views were confirmed through a combination of starting with LV views and angling rightward and RV landmarks. Before performing study examinations, sonographers attended an echocardiographic strain mechanics course to learn the new RV views and to determine when an image was of adequate quality for strain analysis. The training included a more experienced observer with hand held over the examining sonographer’s hand during an instructional examination to ensure proper transducer orientation at 60° rotational intervals. Sonographers performed online strain analyses at the time of study to verify the adequacy of the images.

The volumetric probe was used as an aid in development and training for 60° rotation examinations. After initial learning, this probe was no longer necessary, particularly because of its inability to supply adequate acquisition frame rates.
The views were initially termed “equivalent” in reference to preserved LV transducer plane orientations and as a reminder of where the image data should be entered into the analysis software for planar spatial reconstruction in the resulting target diagram that was designed for the left ventricle. The resulting four-chamber equivalent view has four chambers (right atrium and ventricle, left atrium and ventricle); the two-chamber equivalent view, alternatively called the “outflow” view, has three (right atrium, right ventricle, and RV outflow tract); and the three-chamber equivalent view, alternatively termed the RV “inflow” view, has two chambers (right atrium and ventricle) ( Figure 1 ).
RV 2D Speckle-Tracking Longitudinal Strain Analysis
Longitudinal strain was analyzed in all 40 subjects by two experienced investigators. Offline strain analysis was performed using EchoPAC PC version BT11 (GE Vingmed Ultrasound) using conventional LV software with the RV views substituted for the standard LV views. The reference point was placed at the beginning of the QRS complex. Pulmonary valve closure relative to the QRS complex was defined on a spectral Doppler tracing of pulmonary outflow.
EchoPAC speckle-tracking software was then applied to each properly obtained RV view to produce six segmental peak strain curves and time-to-peak intervals. This software has been previously validated for the determination of global peak strain in the left ventricle from the two-chamber, three-chamber, and four-chamber apical LV views. The planes of those views were maintained in the equivalent apical RV views with 60° counterclockwise rotations between each view ( Figure 1 ). The RV endocardial border was manually traced in end-systole using the medial and lateral tricuspid valve annulus as the beginning and end points in the four-chamber and inflow views. In the outflow view, the myocardium was traced from the tricuspid valve annulus on the posterior wall to the most basal region of the anterior septal wall visible in this view before its transition to the proximal RV outflow tract ( Figure 2 ). The region of interest was adjusted to include myocardial thickness but exclude the pericardium. The integrity of RV speckle-tracking was automatically detected by the software and visually confirmed. In case of poor tracking, the tracing was readjusted or the region of interest thickness changed. Segments with persistent inadequate tracking were excluded from analysis.

Global peak strain was calculated by averaging the peak strain values of all RV segments for that subject. Regional averages (septal, apical, and free wall) of global peak strain or time to peak strain were calculated by averaging the segmental values of the six segments in each of those regions. Two measures of RV mechanical dyssynchrony using time-to-peak methods were analyzed: (1) the standard deviation of the time-to-peak intervals (SDttp) in all segments and (2) the maximum opposing wall delay (MOW), which was measured as the maximal delay in time to peak between the opposing walls in the mid or basal segments. Regional pattern analysis was also applied.
Averaged or calculated strain values were obtained in the 18S model. When the 18S model was compared with the 6S model (traditional four-chamber apical view only), a subscript 18 or 6 denotes which model was used.
Statistical Analysis
Continuous variables (reported as mean ± SD) were compared using paired and unpaired t tests after visualizing the data to confirm normality. Categorical variables (reported as percentages) were tested for differences using Fisher’s exact tests. P values < .05 were considered statistically significant. Averaged strain indices were compared between the 18S and 6S models using absolute mean difference and coefficient of variation (CV), which were calculated in the standard fashion. Statistical analyses were performed using Stata version 12 (StataCorp LP, College Station, TX).
Interobserver and intraobserver variability of averaged and segmental strain measures was reported as absolute mean difference and CV. For interobserver variability, strain analysis was performed on a randomly selected subpopulation of 25 of these subjects using the same cardiac cycle by a second experienced reader blinded to the results of the primary reader. For intraobserver variability, the primary reader also repeated measurements in a blinded fashion no sooner than 1 month after the first assessment on the 25 subjects.
Results
Normal Population
In the normal population, there were 40 young healthy adult patients with normal cardiac function and anatomy. Baseline population characteristics and traditional echocardiographic functional characteristics are listed in Table 1 . Adequate tracking for strain analysis was obtained in 696 of all 720 segments (97%). The anterior free wall segments (RV three-chamber equivalent view) had the lowest strain tracking rate (86%). Averaged strain measures assessing global and regional averaged peak strain are reported for the 18S model in Table 2 . Regional peak strain comparing the free wall, apical, and septal regions was highest in the free wall and lowest in the septum. Individual segmental peak strain is reported in Table 3 .
Variable | Value |
---|---|
Age (y) | 29 (18–52) |
Men | 16 (40%) |
QRS duration ≥ 120 msec | 0 |
TAPSE (mm) | 22 ± 3 (15, 30) |
FAC (%) | 43 ± 5 (35, 57) |
LVEF (%) | 59 ± 4 (50, 65) |
Variable | Echocardiographic strain ( n = 40) | P |
---|---|---|
Function | ||
Global peak strain (%) | −23 ± 2% (−18, −27) | |
Septal peak strain (%) | −20 ± 2% (−16, −24) | <.001 ∗ |
Apical peak strain (%) | −22 ± 4% (−15, −30) | <.001 † |
Free wall peak strain (%) | −27 ± 4% (−20, −36) | <.001 ‡ |
Timing of contraction | ||
Septal time to peak (msec) | 408 ± 25 (357, 487) | <.0011 ∗ |
Apical time to peak (msec) | 421 ± 24 (382, 474) | .0032 † |
Free wall time to peak (msec) | 430 ± 25 (385, 491) | <.0013 ‡ |
Dyssynchrony indices | ||
Basal MOW (msec) | 38 ± 14 (0, 93) | |
Mid MOW (msec) | 29 ± 15 (0, 112) | |
SDttp (msec) | 25 ± 7 (10, 42) | |
Classic pattern | 0 |
∗ The reported P values were obtained from paired t tests comparing septal and apical.
Segment | Peak strain (%) | Intraobserver variability (%, %) | Interobserver variability (%, %) | Time to peak (msec) | Intraobserver variability (msec, %) | Interobserver variability (msec, %) |
---|---|---|---|---|---|---|
Basal (wall no.) | ||||||
Septum (1) | −19 ± 3 (−13, −25) | 0, 8 | 1, 12 | 398 ± 35 (351, 519) | 2, 5 | −5, 7 |
Anterior septum (2) | −17 ± 3 (−13, −36) | 0, 15 | 0, 18 | 415 ± 31 (351, 488) | −8, 6 | −2, 5 |
Anterior FW (3) | −31 ± 8 (−17, −44) | 1, 12 | 0, 15 | 433 ± 39 (305, 514) | −6, 6 | −12, 8 |
Lateral FW (4) | −27 ± 5 (−13, −36) | 0, 15 | 1, 22 | 435 ± 33 (386, 502) | 3, 3 | 1, 4 |
Posterior FW (5) | −24 ± 5 (−16, −42) | 1, 16 | 0, 28 | 435 ± 32 (372, 520) | 2, 6 | 5, 8 |
Posterior septum (6) | −23 ± 3 (−17, −30) | 1, 9 | 1, 15 | 407 ± 35 (342, 496) | 10, 4 | 8, 5 |
Mid (wall no.) | ||||||
Septum (1) | −19 ± 3 (−12, −24) | 0, 7 | 1, 13 | 404 ± 27 (351, 505) | −1, 3 | −11, 5 |
Anterior septum (2) | −19 ± 3 (−13, −27) | 1, 15 | 0, 20 | 426 ± 35 (368, 520) | 2, 4 | 8, 6 |
Anterior FW (3) | −29 ± 5 (−13, −36) | 1, 15 | 2, 18 | 433 ± 34 (379, 514) | 1, 3 | −5, 5 |
Lateral FW (4) | −28 ± 4 (−13, −38) | 1, 10 | 1, 11 | 420 ± 27 (380, 489) | 4, 4 | 0, 8 |
Posterior FW (5) | −26 ± 5 (−16, −37) | 0, 12 | 1, 13 | 423 ± 31 (372, 520) | 5, 4 | 1, 8 |
Posterior septum (6) | −22 ± 3 (−17, −31) | 1, 10 | 0, 14 | 400 ± 26 (342, 465) | 3, 3 | 1, 4 |
Apical (wall no.) | ||||||
Septum (1) | −20 ± 5 (−8, −33) | 0, 22 | 1, 25 | 420 ± 31 (351, 478) | 0, 4 | −9, 7 |
Anterior septum (2) | −22 ± 5 (−14, −33) | 1, 19 | 0, 35 | 427 ± 38 (369, 520) | 4, 4 | 0, 6 |
Anterior FW (3) | −20 ± 7 (−4, −33) | −1, 21 | −1, 40 | 428 ± 35 (379, 505) | −19, 5 | −19, 8 |
Lateral FW (4) | −26 ± 6 (−11, −37) | 1, 16 | 0, 17 | 414 ± 30 (351, 477) | 2, 3 | −4, 8 |
Posterior FW (5) | −26 ± 5 (−15, −37) | 1, 15 | 1, 21 | 417 ± 34 (369, 504) | −5, 4 | −9, 6 |
Posterior septum (6) | −19 ± 6 (−17, −31) | 0, 28 | 0, 29 | 423 ± 29 (379, 491) | 13, 7 | 13, 7 |
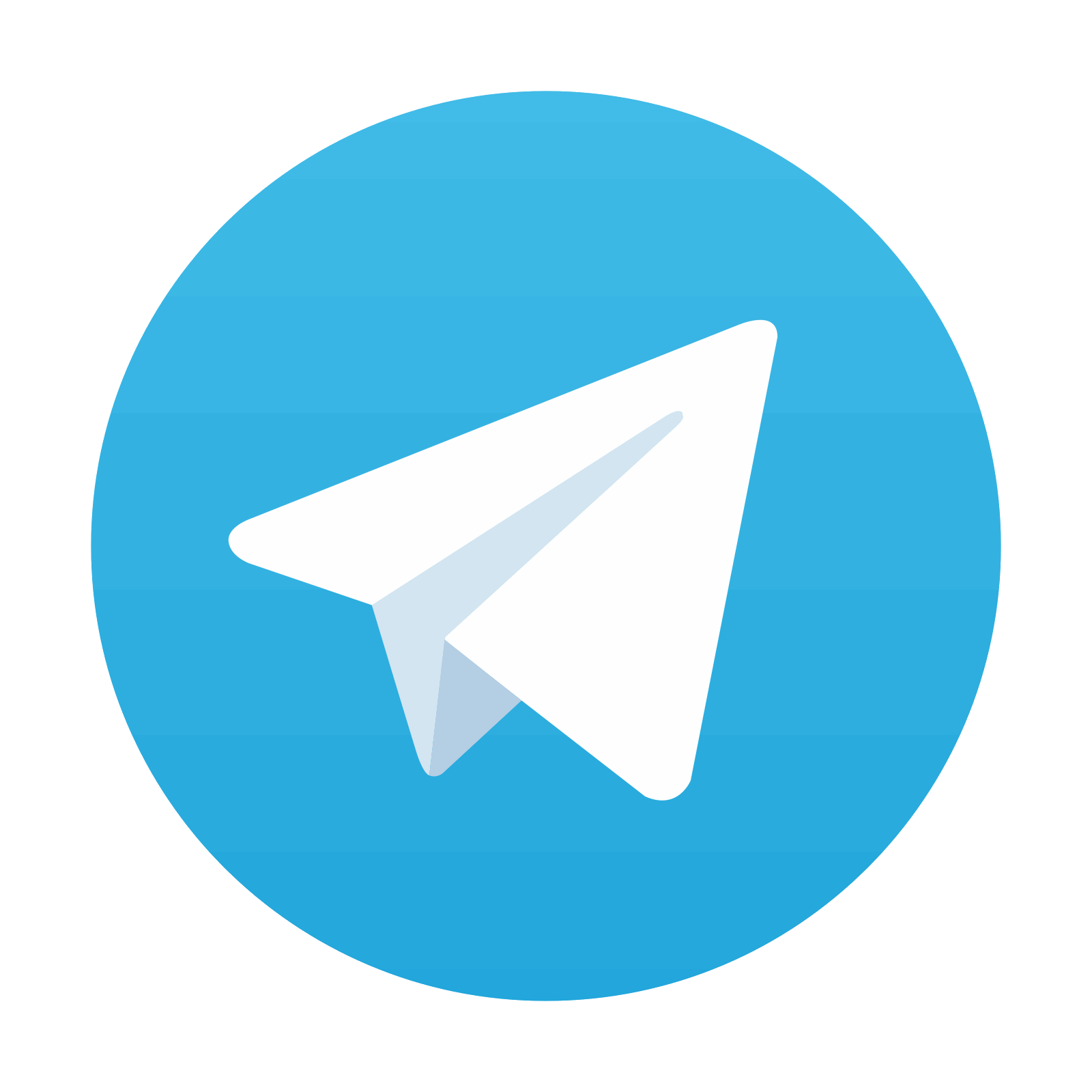
Stay updated, free articles. Join our Telegram channel

Full access? Get Clinical Tree
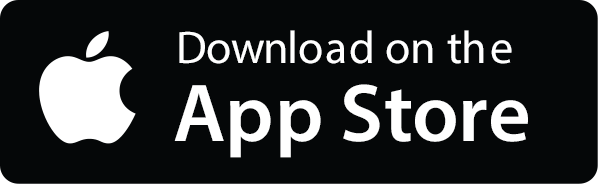
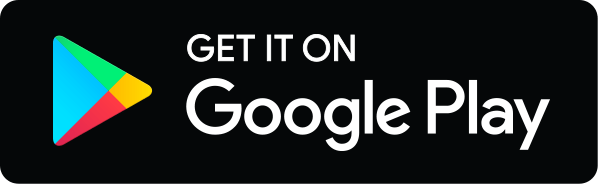
