Background
Right ventricular (RV) global longitudinal strain (RV strain) is a sensitive measure of RV mechanics. Its relationship with standard clinical markers and long-term events in chronic systolic heart failure is not well established. The aim of this study was to examine the ability of RV strain to provide incremental prognostic value to left ventricular (LV) ejection fraction (LVEF) in patients with chronic systolic heart failure.
Methods
In 171 patients with chronic systolic heart failure (LVEF ≤ 35%), a retrospective substudy of RV strain was performed using Velocity Vector Imaging to analyze previously recorded, comprehensive echocardiographic images. Death, cardiac transplantation, and heart failure hospitalization were tracked for 5 years.
Results
In this study cohort (mean age, 57 ± 14 years; mean LVEF, 25 ± 6%), mean RV strain was −11.6 ± 5.4%. More impaired RV strain was associated with increasing New York Heart Association class (rank-sums P < .0001) and greater LV volume (LV end-systolic volume index: r = 0.35, P < .0001). Worse RV strain was associated with reduced LVEF ( r = −0.45, P < .0001), worse LV diastolic dysfunction (E/e′ septal: r = 0.19, P = .017; left atrial volume index: r = 0.18, P = .031), and standard indices of RV systolic and diastolic dysfunction (RV s′: r = −0.43, P < .0001; RV e′/a′: r = 0.16, P = .0040; right atrial volume index: r = 0.20, P = .015). RV strain predicted long-term adverse events (hazard ratio, 1.30; 95% confidence interval, 1.02–1.70; P = .037). Furthermore, RV strain ≥ −14.8% predicted adverse events after adjustment for age, LVEF, RV s′, E/e′ septal, and right atrial volume index.
Conclusion
In patients with chronic systolic heart failure, worse RV strain provides prognostic value incremental to LV function.
The right ventricular (RV) ejection fraction is a powerful predictor of mortality and morbidity in patients with heart failure (HF) secondary to ischemic or idiopathic dilated cardiomyopathy. The identification of variables to predict high-risk cardiac events may result in more aggressive medical or surgical therapy and lead to improved patient survival. However, precise clinical assessment of abnormalities in RV structure and performance is challenging, primarily because RV morphology is complicated and indices of RV function are often poorly visualized by standard echocardiographic techniques.
Speckle-tracking imaging is a recently developed echocardiographic technique that analyzes the degree of myocardial deformation throughout the cardiac cycle. Although high variability has been a concern for two-dimensional strain analysis especially for the right ventricle, the evaluation of RV and left ventricular (LV) function using this technique has been accepted. Furthermore, two-dimensional strain is more robust than tissue Doppler–derived strain, does not have angle dependency, and is easier to calculate. Growing evidence suggests that deformation-based parameters of LV function provide incremental prognostic information over standard parameters in populations at risk for or with HF. However, the long-term prognostic value of deformation-based parameters of RV function and its relationship with standard clinical markers in patients with HF is not well established. We sought to examine the ability of RV global longitudinal strain (RV strain) to provide the incremental prognostic value to LV ejection fraction (LVEF) in patients with chronic systolic HF.
Methods
Study Design and Patient Population
This was a retrospective study of RV mechanics performed in 2012 by analyzing images obtained from 207 patients who were enrolled prospectively in the Assessment of Doppler Echocardiography Prognosis and Therapy (ADEPT) study between May 1, 2001, and June 30, 2003. The ADEPT study has been described in detail. In brief, we prospectively enrolled ambulatory patients who were referred to the outpatient clinic of the Department of Cardiovascular Medicine at Cleveland Clinic for evaluation of symptomatic (New York Heart Association functional classes II–IV) chronic systolic HF. Eligible patients were 18 to 75 years of age with LVEFs ≤ 35%. This study was approved by the Cleveland Clinic Institutional Review Board, and all subjects provided informed consent. Patients were excluded if they had mitral stenosis or had undergone mitral valve surgery, had severe mitral regurgitation (>3+), had severe aortic stenosis (peak velocity > 4 m/sec) or regurgitation, had significant hepatic or renal dysfunction, or were in atrial fibrillation during echocardiographic examination. Patients underwent echocardiographic evaluation of systolic and diastolic performance as well as plasma sample collection. Adverse events (all-cause mortality, cardiac transplantation, and HF hospitalization) were prospectively tracked for 5 years by scheduled telephone follow-up and validated by chart review as previously described.
Transthoracic Echocardiography
Comprehensive transthoracic echocardiography was performed by a highly experienced research sonographer using commercially available HDI 5000 (Phillips Medical Systems, Bothell, WA) and Acuson Sequoia (Siemens Medical Solutions USA, Inc, Malvern, PA) machines. Two-dimensional and color Doppler imaging was performed in standard parasternal and apical views. LV systolic and diastolic indexes were acquired as previously outlined in the ADEPT trial. In terms of mitral annular velocities, we chose to use only a septal measurement of Doppler tissue imaging for the left ventricle. All images were stored digitally and measured later using offline software (syngo Dynamics version 9.0; Siemens Medical Solutions USA, Inc) by an independent investigator who was blinded to the clinical data. All measures of right-sided heart structure and performance were averaged over three cardiac cycles, and two experienced individuals who were blinded to the clinical data made all measurements. RV Doppler indexes were acquired over 10 consecutive beats using sweep speeds of 50 and 100 cm/sec. With pulsed-wave Doppler echocardiography, we acquired transtricuspid flow using a 1-mm to 2-mm sample volume placed at the tricuspid valve leaflet tips in the apical four-chamber view and hepatic vein flow using a 3-mm to 4-mm sample volume placed in the hepatic vein. Tricuspid annular Doppler tissue imaging was performed with standard presets optimized to eliminate background noise and enhance tissue signals using a 5-mm sample volume placed in the four-chamber view. Measurements from pulsed-wave Doppler included transtricuspid peak early and late velocities, early deceleration time, RV Tei index, hepatic vein systolic and diastolic flows, and peak tricuspid annular systolic (s′) and diastolic early (e′) and late (a′) velocities. Tricuspid annular plane systolic excursion and RV fractional area change were measured concordant with the current guidelines. Right atrial (RA) area was measured in the apical four-chamber view at end-systole. RA volume index was calculated using Simpson’s method, in the four-chamber view, and indexed to body surface area.
RV Global Longitudinal Strain Measurements
Measurements were performed offline using dedicated software (Velocity Vector Imaging [VVI]; Siemens Medical Solutions USA, Inc) in 2012. One cardiac cycle was selected for an apical four-chamber view, the endocardial border was traced manually in the end-diastolic frame, and the software subsequently traced the borders in the other frames automatically. The vectors of the velocities of the endocardial points were then displayed and overlaid onto the B-mode images. In segments with poor tracking (assessed subjectively), endocardial borders were readjusted until better tracking was achieved. If this was unattainable, that segment was excluded. The right ventricle was divided into six standard segments (free and septal walls at the basal, middle, and apical levels), and graphical displays of deformation parameters for each segment were then generated automatically. An RV global longitudinal strain curve was provided by averaging each of six regional strain curves. RV strain was defined as the peak negative value on the RV global longitudinal strain curve during the entire cardiac cycle ( Figure 1 ). RV free wall strain was also defined as the peak negative value on the RV free wall strain curve. We obtained RV strain only in the case of adequate tracking quality in at least five of the six segments. All measurements were made by individual research personnel blinded from data analyses.

Interobserver and Intraobserver Variability
Interobserver and intraobserver variability for RV strain was studied in a group of 17 randomly selected subjects by one observer repeated twice and by two investigators who were unaware of each other’s measurements and of the study time point. The coefficient of variation and the bias (mean difference) and limits of agreement (1.96 SDs of the difference) between the first and second measurements were determined.
Statistical Analysis
Continuous variables are summarized as mean ± SD if normally distributed and as median and interquartile range if not normally distributed. Normality was assessed using the Shapiro-Wilk W test. Spearman’s rank correlation method was used as a nonparametric measure of association between RV strain and clinical and echocardiographic indices. Wilcoxon or Kruskal-Wallis rank-sums tests were used to compare differences in RV strain across clinical categories, while proportions were compared using contingency table analysis. A Cox proportional-hazards regression model was used to assess the clinical risk associated with increasing continuous standardized increments of RV strain. The optimal receiver operating characteristic curve cutoff value for the prediction of adverse clinical events was chosen as the value maximizing sensitivity plus specificity. The proportional-hazards assumption was verified with log (time) versus log [−log (survival)] plots. Kaplan-Meier survival plots were calculated from baseline to time of adverse event and compared using the log-rank test. Statistical analyses were performed with using JMP version 9.0.0 (SAS Institute Inc, Cary, NC). All P values reported are from two-sided tests, and P values < .05 were considered statistically significant.
Results
Study Population
Of the 207 patients in ADEPT, 171 (88%) had RV strain that could be measured in four-chamber views. The average frame rate of the clips for RV strain analysis was 30.0 ± 1.3 frames/sec. The coefficient of variation of intraobserver variability for RV strain was 10 ± 6%. The coefficient of variation of interobserver variability was 10 ± 7%. The bias and limits of agreement of intraobserver and interobserver variability were 0.1 ± 4.1% and 0.3 ± 4.3%, respectively. The baseline clinical and echocardiographic parameters are summarized in Table 1 . For the study population as a whole, mean RV strain was −11.6 ± 5.4%. RV strain worsened with increasing New York Heart Association class ( P < .0001) and higher N-terminal pro–B-type natriuretic peptide ( r = 0.34, P < .0001). Compared with those who did not develop cardiac events, patients who did had greater left atrial and RA volume indices, greater LV mass indices, lower LVEFs, lower RV s′, higher E/e′ septal, higher RV e′/a′, and lower RV strain ( Table 1 ).
Variable | Overall population ( n = 171) | Cardiac events | P | |
---|---|---|---|---|
Yes ( n = 69) | No ( n = 102) | |||
Demographics | ||||
Age (y) | 57 ± 14 | 57 ± 15 | 58 ± 13 | .723 |
Men | 124 (73%) | 53 (77%) | 71 (70%) | .297 |
HF history | ||||
NYHA class III or IV | 64 (38%) | 37 (54%) | 27 (27%) | <.001 |
Ischemic etiology | 69 (41%) | 35 (51%) | 34 (34%) | .026 |
Systolic blood pressure (mm Hg) | 114 ± 20 | 110 ± 22 | 117 ± 18 | .007 |
Comorbidities | ||||
Hypertension | 91 (54%) | 34 (50%) | 57 (57%) | .372 |
Diabetes mellitus | 42 (25%) | 24 (35%) | 18 (18%) | .014 |
Medications | ||||
ACE inhibitors and/or ARBs | 154 (92%) | 62 (91%) | 92 (92%) | .850 |
β-blockers | 93 (55%) | 36 (52%) | 57 (57%) | .536 |
Spironolactone | 38 (23%) | 18 (27%) | 20 (21%) | .344 |
Loop diuretics | 127 (75%) | 59 (86%) | 68 (68%) | .008 |
Digoxin | 96 (60%) | 41 (62%) | 55 (58%) | .590 |
Echocardiographic data | ||||
RV strain (%) | −11.6 ± 5.4 | −10.5 ± 5.1 | −12.4 ± 5.5 | .013 |
RV systolic strain rate (%) | −0.72 ± 0.34 | −0.63 ± 0.31 | −0.79 ± 0.35 | .003 |
RV early diastolic strain rate (%) | 0.62 ± 0.34 | 0.60 ± 0.35 | 0.64 ± 0.33 | .325 |
RV late diastolic strain rate (%) | 0.52 ± 0.35 | 0.45 ± 0.32 | 0.56 ± 0.36 | .030 |
LVEF (%) | 25 ± 6 | 24 ± 6 | 26 ± 6 | .006 |
LV end-diastolic dimension (cm) | 6.3 ± 0.9 | 6.4 ± 0.9 | 6.2 ± 0.8 | .023 |
LVEDV index (mL/m 2 ) | 115 ± 38 | 116 ± 37 | 113 ± 38 | .631 |
LVESV index (mL/m 2 ) | 87 ± 33 | 91 ± 33 | 84 ± 34 | .178 |
LV mass index (g/m 2 ) | 157 ± 45 | 165 ± 42 | 152 ± 46 | .027 |
LA volume index (mL/m 2 ) | 42 ± 17 | 45 ± 18 | 40 ± 16 | .026 |
RA volume index (mL/m 2 ) | 28 ± 15 | 35 ± 17 | 24 ± 11 | <.0001 |
Mitral E/A ratio | 1.7 ± 1.4 | 2.2 ± 1.5 | 1.3 ± 1.2 | <.0001 |
Mitral DT (msec) | 176 ± 58 | 150 ± 43 | 195 ± 61 | <.0001 |
Tricuspid E/A ratio | 1.2 ± 0.5 | 1.2 ± 0.6 | 1.3 ± 0.5 | .273 |
Tricuspid DT (msec) | 203 ± 58 | 205 ± 47 | 203 ± 66 | .612 |
TAPSE | 1.79 ± 0.40 | 1.67 ± 0.40 | 1.87 ± 0.39 | .002 |
RV FAC | 35 ± 9 | 32 ± 8 | 37 ± 9 | <.0001 |
RV Tei index | 0.84 ± 0.31 | 0.90 ± 0.27 | 0.79 ± 0.33 | .019 |
RV systolic pressure (mm Hg) | 34 ± 13 | 37 ± 12 | 31 ± 13 | <.001 |
Mitral annular DTI | ||||
s′ septal (cm/sec) | 4.4 ± 1.2 | 4.1 ± 1.2 | 4.7 ± 1.2 | .010 |
e′ septal (cm/sec) | 4.5 ± 1.6 | 4.1 ± 1.4 | 4.7 ± 1.7 | .035 |
a′ septal (cm/sec) | 6.7 ± 2.6 | 5.5 ± 2.3 | 7.5 ± 2.5 | <.0001 |
E/e′ septal ratio | 20 ± 12 | 25 ± 15 | 16 ± 8 | <.0001 |
s′ lateral (cm/sec) | 5.8 ± 2.1 | 5.3 ± 1.8 | 6.2 ± 2.2 | .001 |
e′ lateral (cm/sec) | 7.3 ± 4.3 | 6.9 ± 3.2 | 7.6 ± 4.9 | .656 |
a′ lateral (cm/sec) | 7.9 ± 3.4 | 6.7 ± 2.8 | 8.7 ± 3.5 | <.001 |
E/e′ lateral ratio | 13.4 ± 10.0 | 15.7 ± 12.3 | 11.7 ± 7.6 | .002 |
Lateral tricuspid annular DTI | ||||
s′ tricuspid (cm/sec) | 10.0 ± 3.4 | 9.0 ± 3.3 | 1.6 ± 3.3 | .002 |
e′ tricuspid (cm/sec) | 8.1 ± 3.0 | 7.7 ± 2.9 | 8.4 ± 3.1 | .216 |
a′ tricuspid (cm/sec) | 11.8 ± 5.0 | 10.4 ± 5.5 | 12.8 ± 4.4 | .003 |
Laboratory data | ||||
NT-proBNP (pg/mL) | 1,158 (469–3,429) | 2,529 (642–5,242) | 882 (391–2,028) | <.001 |
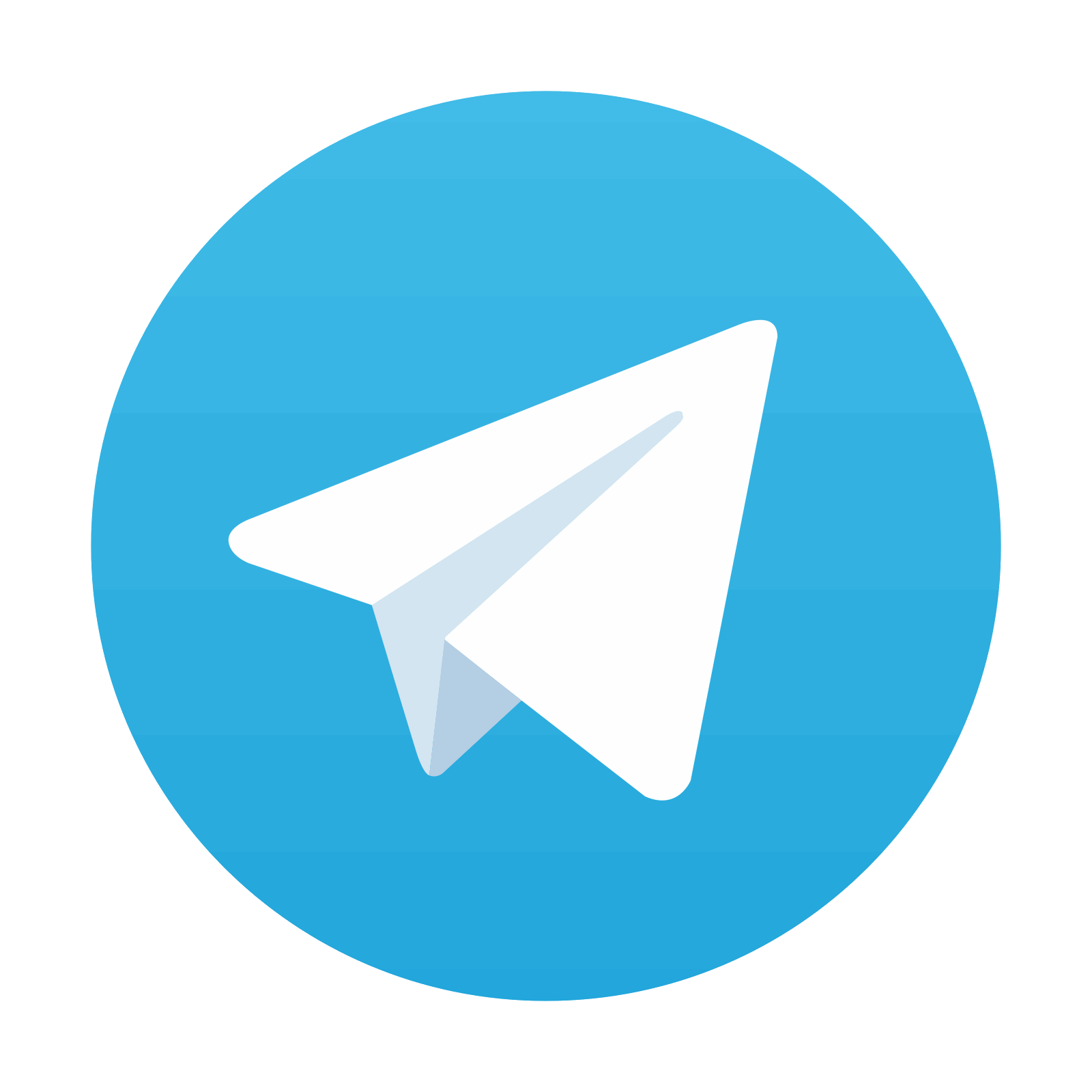
Stay updated, free articles. Join our Telegram channel

Full access? Get Clinical Tree
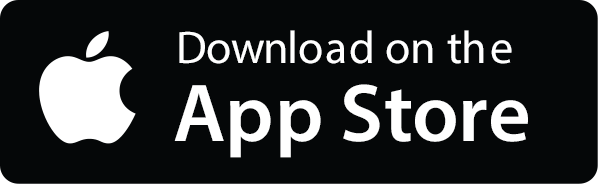
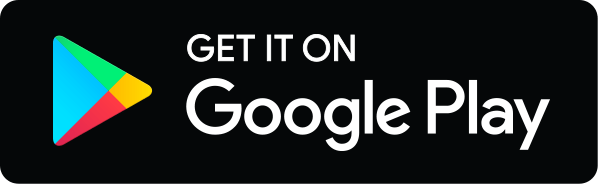
