Background
Right ventricular (RV) fractional area of change (FAC) is a quantitative two-dimensional echocardiographic measurement of RV function. RV FAC expresses the percentage change in the RV chamber area between end-diastole (RV end-diastolic area [RVEDA]) to end-systole (RV end-systolic area [RVESA]). The objectives of this study were to determine the maturational (age- and weight-related) changes in RV FAC and RV areas and to establish reference values in healthy preterm and term neonates.
Methods
A prospective longitudinal study was conducted in 115 preterm infants (23–28 weeks’ gestational age at birth, 500–1,500 g). RV FAC was measured at 24 hours of age, 72 hours of age, and 32 and 36 weeks’ postmenstrual age (PMA). The maturational patterns of RVEDA, RVESA, and RV FAC were compared with those in 60 healthy full-term infants in a cross-sectional study (≥37 weeks, 3.5 ± 1 kg), who underwent echocardiography at birth ( n = 25) and 1 month of age ( n = 35). RVEDA and RVESA were traced in the RV-focused apical four-chamber view, and FAC was calculated using the formula 100 × [(RVEDA − RVESA)/RVEDA)]. Premature infants who developed chronic lung disease or had clinically and hemodynamically significant patent ductus arteriosus were excluded ( n = 55) from the reference values. Intra- and interobserver reproducibility analysis was performed.
Results
RV FAC ranged from 26% at birth to 35% by 36 weeks’ PMA in preterm infants ( n = 60) and increased almost 2 times faster in the first month of age compared with healthy term infants ( n = 60). Similarly, RVEDA and RVESA increased throughout maturation in both term and preterm infants. RV FAC and RV areas were correlated with weight ( r = 0.81, P < .001) but were independent of gestational age at birth ( r = 0.3, P = .45). RVEDA and RVESA were correlated with PMA in weeks ( r = 0.81, P < .001). RV FAC trended lower in preterm infants with bronchopulmonary dysplasia ( P = .04) but was not correlated with size of patent ductus arteriosus ( P = .56). There was no difference in RV FAC based on gender or need for mechanical ventilation.
Conclusions
This study establishes reference values of RV areas (RVEDA and RVESA) and RV FAC in healthy term and preterm infants and tracks their maturational changes during postnatal development. These measures increase from birth to 36 weeks’ PMA, and this is reflective of the postnatal cardiac growth as a contributor to the maturation of cardiac function These measures are also linearly associated with increasing weight throughout maturation. This study suggests that two-dimensional RV FAC can be used as a complementary modality to assess global RV systolic function in neonates and facilitates its incorporation into clinical pediatric and neonatal guidelines.
Right ventricular (RV) performance is an important determinant of clinical status and long-term outcome in preterm and term neonates with cardiopulmonary pathology. RV mechanics begin to undergo maturational changes in the early postnatal period that have long-term influence on cardiac function. Preterm birth is associated with global alterations in myocardial structure and function by adult age, including smaller RV size and greater RV mass than normal. Intuitively, alterations in RV function in the first months of age may serve as a sensitive marker of altered hemodynamics, as well as of the onset of clinical and subclinical cardiorespiratory dysfunction. Therefore, early assessment of RV performance, in healthy and critically ill preterm infants, is essential for the surveillance in the early neonatal period.
Recent pediatric and neonatal echocardiographic guidelines recommend performing quantitative measurements of RV systolic function using at least one of the following echocardiographic measures: (1) RV fractional area of change (FAC), (2) tricuspid annular plane systolic excursion (TAPSE), or (3) RV myocardial performance index (MPI). Normal values for TAPSE and RV MPI have previously been established in separate studies of healthy children, neonates, and preterm infants, but, there are no reference normal data in children or neonates for RV FAC.
The structural and functional organization of the right ventricle has a complex three-dimensional myofiber arrangement. The dominant longitudinal shortening of the right ventricle provides the major contribution to RV ejection fraction (EF) and stroke volume during systole, and there is a strong association between RV FAC measured by echocardiography and RV EF determined by magnetic resonance imaging (MRI). RV FAC that describes this longitudinal shortening on a global level may provide a sensitive measure of RV function in neonates. RV FAC as an echocardiographic tool to assess RV systolic function has been applied in adults children, and neonates. However, the reference values for the controls cohorts for the pediatric studies refer to those for adults. The use of RV FAC in children and neonates requires knowledge of the range of normal values specific to these patient populations as well as the variations due to maturational changes before routine clinical applications of RV FAC can be implemented in neonates.
Therefore, the aims of this study were to determine the maturational (age- and weight-related) changes of RV area and RV FAC in preterm infants, compare the evolution with term infants, and establish normal reference values in both preterm and term neonates.
Methods
Study Population
Preterm Infants
One hundred fifteen preterm infants (born between 23-0/7 and 28-6/7 weeks’ gestation) were prospectively enrolled from among infants participating in the Premature and Respiratory Outcomes Program (ClinicalTrials.gov identifier NCT01435187). All the infants were enrolled at Washington University/St Louis Children’s Hospital neonatal intensive care unit between August 2011 and August 2013. All 115 preterm infants underwent echocardiography at 32 and 36 weeks’ postmenstrual age (PMA). The timings of the echocardiographic studies at 32 and 36 weeks’ PMA were carefully selected to avoid the early postnatal period of clinical and cardiopulmonary instability and early mortality associated with extreme preterm birth. Choosing to study all infants at a common PMA optimizes the determination of the impact of gestational and chronological age on cardiac function at a specific developmental stage and allows the analysis of measures by postgestational weeks from birth. To evaluate the pattern of longitudinal maturation by gestational age at birth and birth weight, 30 of the 115 infants underwent echocardiography at two additional time points, 24 and 72 hours of age. In these 30 infants, we tracked the maturational patterns at 24 hours of age, 72 hours of age, 32 weeks’ PMA, and 36 weeks PMA. Available patient clinical and demographic characteristics were obtained at each time point and are summarized in Table 1 .
Timing of echocardiography | 24 h of age | 72 h of age | 32 wk PMA | 36 wk PMA |
---|---|---|---|---|
Infants recruited at each time point | 30 | 30 | 115 | 115 |
Infants included in reference values ∗ | 20 | 20 | 60 | 60 |
Gestational age at birth | 27 ± 1 | 27 ± 1 | 26 ± 2 | 26 ± 2 |
Weight at birth | 915 ± 118 | 915 ± 118 | 899 ± 218 | 899 ± 218 |
Weight at examination | ||||
Male/female | 9/11 | 9/11 | 27/33 | 27/33 |
Antenatal steroids | 17/20 | 17/20 | 53/60 | 53/60 |
Surfactant | 20/20 | 20/20 | 60/60 | 60/60 |
Respiratory | ||||
Weight (kg) at echocardiography | 858 ± 111 | 814 ± 140 | 1,400 ± 238 | 2,192 ± 297 |
RR (breaths/min) | 52 ± 7 | 48 ± 11 | 52 ± 9 | 50 ± 9 |
Invasive mechanical ventilation | 17/30 | 14/30 | 1/115 | 0/115 |
BPD | ||||
No/mild | 21/30 | 21/30 | 60/115 | 60/115 |
Moderate/severe ∗ | 9/30 | 9/30 | 55/115 | 55/115 |
Cardiovascular | ||||
PDA | 27/30 | 23/30 | 19/115 | 11/115 |
Small | 17 | 13 | 13 | 8 |
Moderate ∗ | 6 | 5 | 5 | 3 |
Large ∗ | 4 | 5 | 1 | 0 |
HR (beats/min) | 159 ± 14 | 164 ± 12 | 148 ± 16 | 146 ± 11 |
SBP (mm Hg) | 50 ± 10 | 59 ± 10 | 73 ± 10 | 80 ± 9 |
DBP (mm Hg) | 33 ± 7 | 37 ± 9 | 42 ± 9 | 46 ± 10 |
MAP (mm Hg) | 39 ± 7 | 44 ± 8 | 49 ± 14 | 55 ± 14 |
∗ Infants with moderate or large PDA at 24 or 72 hours of age, or any size PDA at 32 or 36 weeks’ PMA, were excluded from the reference values. Infants requiring respiratory support at 36 weeks’ PMA, classified as moderate or severe BPD, were also excluded from the reference values.
Inclusion criteria included preterm infants born between 23-0/7 and 28-6/7 weeks’ gestational age and alive at 1 year of age. Infants with any suspected congenital anomalies of the airways, congenital heart disease (except hemodynamically insignificant ventricular or atrial septal defects), intrauterine growth restriction, or small size for gestational age were excluded from the study. Infants with qualitative RV or left ventricular systolic dysfunction and/or echocardiographic signs of pulmonary hypertension (i.e., unusual degree of RV hypertrophy, flat septum, or elevated tricuspid regurgitation velocity) were also excluded from the reference values.
Patients with clinically and hemodynamically significant patent ductus arteriosus (PDA), defined as moderate to large PDA, were excluded from the reference values. We used the relationship of the PDA to left pulmonary artery (LPA) to define size and clinical significance (large = PDA/LPA ratio ≥ 1, moderate = PDA/LPA ratio < 1 but ≥ 0.5, and small = PDA/LPA ratio < 0.5). Spontaneous closure of the PDA in extremely low gestational age neonates may not occur in the first week of life, and this delayed closure may be a physiologic consequence of increased pulmonary vascular resistance from pulmonary immaturity. Infants with moderate to large PDA based on its relationship to the LPA have a 15 times greater likelihood of requiring treatment for clinically and hemodynamically significant PDA than those with small PDA. We therefore excluded infants with moderate to large PDA in the first 4 days of age and any size PDA at 32 and 36 weeks’ PMA from the reference values.
Only infants with “respiratory healthiness” were included for analysis. In the first few months of age, the definition of “respiratory healthiness” may become difficult as a large majority of premature infants present with acute respiratory failure after birth and often require some sort of respiratory support up to 36 weeks’ PMA. Respiratory disease syndrome and the need for mechanical ventilation are common in preterm birth in the early postnatal period. Moderate and severe bronchopulmonary dysplasia (BPD), defined as the need for persistent supplemental oxygen support at 36 weeks’ PMA, is recognized as the most significant respiratory consequence of premature birth in the late postnatal period. Therefore, infants with any need for oxygen supplementation at 36 weeks’ PMA, classified as moderate or severe BPD, were excluded from the reference values. We assessed for the contributions of mechanical ventilation and respiratory disease syndrome, as well as BPD, as defined by the 2001 National Institutes of Health (NIH) BPD workshop ( Appendix 1 ), in a subanalysis from birth through 36 weeks’ PMA.
Healthy Term Infants
RV areas and RV FAC were acquired in 60 healthy full-term infants enrolled as a control cohort from the St Louis Children’s Hospital newborn nurseries. These infants were enrolled from a control group from the Premature and Respiratory Outcomes Program and from a control cohort enrolled through the Maternal Lipid Metabolism and Neonatal Heart Function in Diabetes study (ClinicalTrials.gov identifier NCT01346527). Twenty-five infants underwent echocardiography at birth (5 ± 3 days) and 35 separate infants at 1 month of age (30 ± 7 days). Infants were included from each study if they were ≥37 weeks’ gestational age at birth. Patients were excluded if they required oxygen supplementation or respiratory support in the first month of age, had abnormal findings on chest radiography, or were diagnosed with congenital heart defects other than PDA or patent foramen ovale. We compared the maturational patterns from birth to 1 month of age with the observed patterns in preterm infants.
We obtained informed written consent from all parents in each study, and the institutional review board of Washington University approved the studies.
Echocardiographic Analysis of FAC
Standard echocardiograms were acquired in the resting state without sedation by one designated experienced primary pediatric cardiac sonographer (T.J.S.) Two-dimensional, real-time, grayscale images were acquired by a commercially available ultrasound imaging system (Vivid 7 or 9; GE Medical Systems, Milwaukee, WI). The images were obtained using a transducer centered frequency phased-array probe (ranging between 7.5 and 12 MHz) and optimized to visualize the myocardial walls. Two-dimensional images were acquired from the RV-focused apical four-chamber view using a previously published protocol for RV image acquisition and data analysis. The image data were digitally stored for three cardiac cycles in cine loop format for offline analysis by vendor-customized semiautomated software analysis program (EchoPAC version 110.0.x; GE Medical Systems).
RV end-systolic area (RVESA) and RV end-diastolic area (RVEDA) were measured in the RV-focused apical four-chamber view. Beats with similar RR intervals were used to minimize errors in calculation. RVESA and RVEDA were measured at the frames just before tricuspid valve opening and just after the valve closure, respectively. A “sail sign” was traced from the (1) septal side of the tricuspid annular plane (septal-tricuspid annular hinge point) to the (2) apical-septal point and then to the (3) RV free wall side of the tricuspid annular plane (lateral-tricuspid annular hinge point). The trabeculations were included in the RV area measurements to avoid underestimating the areas. RV endocardial areas were delineated for three consecutive cardiac cycles, and the mean of the three values was used to compute FAC. RV FAC was automatically calculated from the EchoPAC software analysis as follows: 100 × [RVEDA (cm 2 ) − RVESA (cm 2 )]/RVEDA (cm 2 ) ( Figure 1 ).

Reproducibility
To consider intra- and interobserver variability, RVEDA, RVESA, and RV FAC were measured in 50% of the preterm ( n = 60) and term ( n = 30) infants by two investigators (P.T.L. and B.D.), both of whom were blinded to patient demographic and clinical data. Each observer used the same measurement protocol and was blinded to the other’s results. The images used for the reproducibility analysis in the preterm population were randomly chosen from all four time points and included both healthy preterm infants and those infants who developed BPD or had clinically and hemodynamically significant PDA. The reproducibility analysis was based on a combination of Bland-Altman plot analysis (percentage bias, 95% limits of agreements) and coefficient of variation. The strength of agreement between the intra- and interobservations was evaluated by simple linear regression analysis (Pearson’s correlation). P values < .05 were considered to indicate statistical significance.
Statistical Methods
All data are expressed as mean ± SD or as percentages. Continuous variables were tested for normality using the Kolmogorov-Smirnov test and a histogram illustration of the data. Analysis of variance and Student t tests were used to compare the changes in RV areas and RV FAC from birth to 36 weeks’ PMA in the preterm infants and to compare the patterns with those in healthy full-term infants between birth and 1 month of age. The Mann-Whitney test was used when normal distribution was not achieved. The correlation between the measures and gestational age and birth weight were analyzed with the Pearson correlation coefficient. A stepwise multiple regression was used to estimate RV FAC, RVEDA, and RVESA from postnatal age (in weeks), weight, and gender. The statistical analysis was performed using SPSS version 14.0 (SPSS, Inc, Chicago, IL).
Results
Patient Characteristics
The clinical and demographic characteristics of the preterm patients are summarized and compared in Table 1 . Weight, systolic blood pressure, diastolic blood pressure, and mean arterial blood pressure increased from birth to 36 weeks’ PMA, and heart rate decreased, as expected ( Table 1 ).
Maturational Patterns of RV Areas and RV FAC
In preterm infants, RV FAC ranged from 26% at birth to 35% by 32 weeks’ PMA (4–8 weeks of age). In the healthy full-term infants, RV FAC ( P < .001) increased linearly from 33% at birth to 39% at 1 month of age ( Table 2 ). RV FAC in preterm infants increased almost 2 times faster in the first month of age compared with healthy term infants ( Table 2 ).
Birth | 1 mo | Slope of change | P | |
---|---|---|---|---|
Full-term infants | ( n = 25) | ( n = 35) | ||
RVEDA (cm 2 ) | 3.7 ± 0.7 | 4.8 ± 0.6 | 0.38 | <.001 |
RVESA (cm 2 ) | 2.4 ± 0.4 | 2.9 ± 0.4 | 0.26 | <.001 |
RV FAC (%) | 33 ± 5 | 39 ± 4 | 1 | <.001 |
Preterm infants | ( n = 20) | ( n = 20) | ||
RVEDA (cm 2 ) | 1.8 ± 0.2 | 2.3 ± 0.2 | 0.12 | <.001 |
RVESA (cm 2 ) | 1.3 ± 0.2 | 1.5 ± 0.3 | 0.05 | <.001 |
RV FAC (%) | 26 ± 4 | 35 ± 5 | 1.8 | <.001 |
Maturational Patterns of RV Areas and RV FAC in Healthy Preterm Infants
Overall, RV areas and RV FAC increased from birth to 36 weeks in preterm infants ( P < .001; Figure 2 ). A time-specific maturational pattern revealed that RVEDA and RVESA were stable ( P = .37) between 24 and 72 hours of age. Both increased linearly between 72 hours and 32 weeks’ PMA and also between 32 and 36 weeks’ PMA ( Figure 2 A). However, there was differential rate of growth for the two RV areas between 72 hours and 32 weeks’ PMA ( Figure 2 A). RVEDA increased 2.6 times more rapidly than RVESA, resulting in an overall increase in RV FAC. As a result, RV FAC increased from 26 ± 4% at birth to 35 ± 4% ( P < .001) at 32 weeks’ PMA ( Figure 2 B). Both RVESA and RVEDA increased at a similar rate between 32 and 36 weeks’ PMA, resulting in a constant RV FAC between 32 and 36 weeks’ PMA ( P = .92) ( Figure 2 ).

RVEDA, RVESA, and RV FAC were analyzed for the effect of birth weight and gestational age–related effects using percentile charts (mean ± 2 SDs). RV areas and RV FAC were linearly associated with birth weight ( r = 0.78, P = .001) but not with gestational age at birth ( r = 0.43, P = .52). RVEDA, RVESA, and RV FAC were further analyzed at 32 and 36 weeks’ PMA in all 60 healthy preterm patients to produce postnatal age- and weight-related percentile charts (mean ± 2 SDs) ( Figure 3 and Figure 4 ). RVEDA and RVESA were associated with both increasing weight ( r = 0.84, P < .001; Table 3 ) and postnatal age ( r = 0.72, P < .001; Table 4 ). RV FAC was associated only with increasing weight ( r = 0.68, P = .01) but not with postnatal age beyond 1 month ( r = 0.15, P = .61).


Weight (g) | Infants ( n ) | RVEDA (cm 2 ) | RVESA (cm 2 ) | RV FAC (%) |
---|---|---|---|---|
600 | 3 | 1.5 ± 0.2 | 1.2 ± 0.1 | 23 ± 2 |
700 | 3 | 1.9 ± 0.3 | 1.3 ± 0.2 | 29 ± 8 |
800 | 6 | 1.8 ± 0.2 | 1.3 ± 0.1 | 27 ± 5 |
900 | 6 | 1.9 ± 0.2 | 1.3 ± 0.2 | 28 ± 7 |
1,000 | 5 | 2.3 ± 0.4 | 1.4 ± 0.2 | 36 ± 6 |
1,100 | 11 | 2.3 ± 0.5 | 1.5 ± 0.3 | 34 ± 5 |
1,200 | 10 | 2.3 ± 0.4 | 1.6 ± 0.2 | 34 ± 6 |
1,300 | 13 | 2.4 ± 0.5 | 1.7 ± 0.4 | 34 ± 6 |
1,400 | 14 | 2.6 ± 0.4 | 1.8 ± 0.3 | 33 ± 4 |
1,500 | 13 | 2.7 ± 0.4 | 1.8 ± 0.4 | 36 ± 4 |
1,600 | 4 | 2.8 ± 0.3 | 2.0 ± 0.2 | 37 ± 5 |
1,700 | 10 | 2.9 ± 0.4 | 1.9 ± 0.3 | 36 ± 5 |
1,800 | 5 | 3.2 ± 0.7 | 2.3 ± 0.5 | 33 ± 4 |
1,900 | 6 | 3.2 ± 0.7 | 2.2 ± 0.6 | 34 ± 4 |
2,000 | 9 | 3.4 ± 0.5 | 2.4 ± 0.3 | 35 ± 6 |
2,100 | 5 | 3.5 ± 0.6 | 2.3 ± 0.4 | 37 ± 6 |
2,200 | 8 | 3.6 ± 0.4 | 2.3 ± 0.3 | 37 ± 7 |
2,300 | 8 | 3.8 ± 0.7 | 2.5 ± 0.5 | 35 ± 4 |
2,400 | 6 | 3.8 ± 0.6 | 2.7 ± 0.5 | 36 ± 5 |
2,500 | 7 | 3.9 ± 0.3 | 2.6 ± 0.4 | 34 ± 7 |
2,600 | 4 | 4.3 ± 0.7 | 3.1 ± 0.6 | 34 ± 5 |
2,700 | 4 | 4.4 ± 0.4 | 2.8 ± 0.2 | 36 ± 3 |
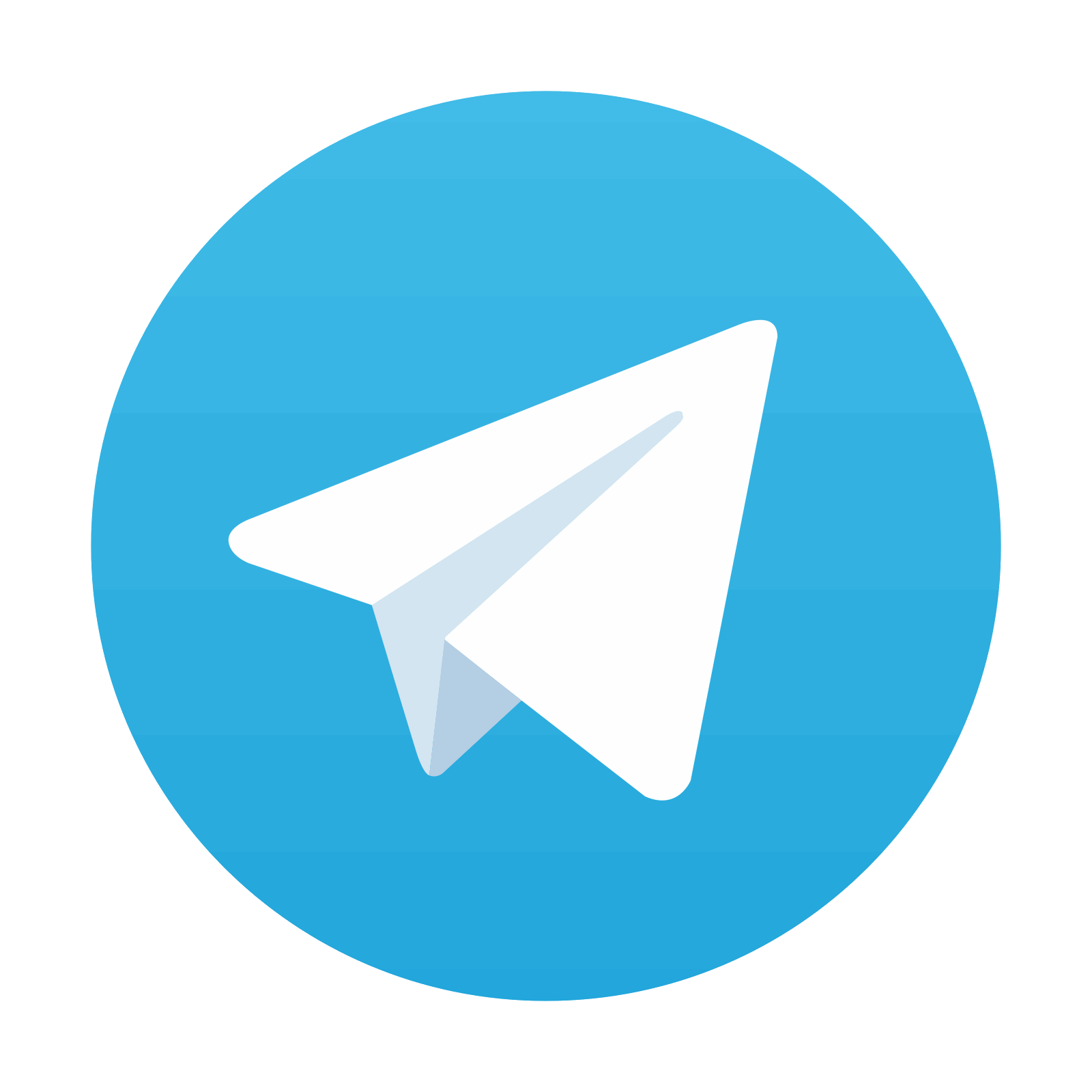
Stay updated, free articles. Join our Telegram channel

Full access? Get Clinical Tree
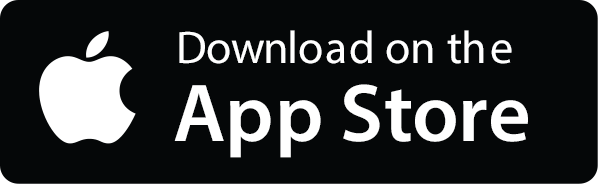
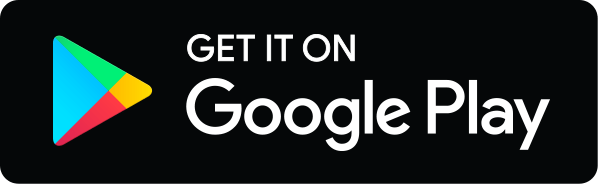
