Background
The aim of this study was to assess changes in right ventricular (RV) parameters determined by three-dimensional (3D) echocardiography and speckle-tracking echocardiography in patients with acute pulmonary embolism and RV dysfunction without systemic hypotension (submassive pulmonary embolism).
Methods
Sixty-six patients were prospectively studied at the onset of the acute episode and after median follow-up periods of 30 days and 6 months. Sixty-six controls were selected. RV fractional area change, tricuspid annular plane systolic excursion, and myocardial performance index were determined. RV systolic pressure was assessed using continuous-wave Doppler echocardiography. Three-dimensional RV ejection fraction (RVEF) was calculated. Two-dimensional peak systolic RV longitudinal strain (RVLS) was measured in the basal free wall, mid free wall (MFW), and apical free wall and the septum.
Results
Tricuspid annular plane systolic excursion and fractional area change were smaller and myocardial performance index was larger compared with controls ( P < .05). Global RVLS ( P < .05), MFW RVLS ( P < .001), and 3D RVEF ( P < .001) were lower in patients with pulmonary embolism than in controls. There was earlier reversal of MFW RVLS values on 30-day follow-up and longer reversal of 3D RVEF and RV systolic pressure values at 6-month follow-up. Receiver operating characteristic curve analysis showed that changes in 3D RVEF and MFW RVLS were the most sensitive predictors of adverse events. By multivariate analysis, RV systolic pressure ( P = .007), MFW RVLS ( P = .002), and 3D RVEF ( P = .001) were independently associated with adverse outcomes.
Conclusions
Acute submassive pulmonary embolism has a significant impact on RV function as assessed by 3D echocardiography and speckle-tracking echocardiography. Decreases in MFW RVLS and 3D RVEF may persist during short-term and long-term follow-up and correlate with unfavorable outcomes.
The in-hospital mortality of patients with massive pulmonary embolism (PE) varies from 25% to 50%, whereas that associated with so-called submassive PE (defined as the presence of right ventricular (RV) dysfunction without systemic hypotension) ranges from 3% to 15%, and that associated with nonmassive PE is <5%. Although there is consensus that thrombolytic therapy, catheter embolectomy, or surgery is indicated in patients with right heart failure and hemodynamic instability, the appropriate treatment of patients with submassive PE remains controversial. In this subset of patients, RV dysfunction should be identified and clinical and laboratory aspects evaluated to judge the level of severity.
Quantitative echocardiographic assessment of RV function is gaining increasing interest in cardiac diseases that affect the right ventricle, such as congenital heart disease and acute or chronic pulmonary hypertension. Two-dimensional speckle-tracking echocardiography (STE) is a new tool to evaluate myocardial wall movements and deformation, and it has been suggested that the use of indexes derived from STE can be proposed as an adjunctive tool in the comprehensive evaluation of RV function. Real-time three-dimensional (3D) echocardiography allows the measurement of RV end-diastolic volume (RVEDV) and RV ejection fraction (RVEF) irrespective of its shape and more reliably compared with two-dimensional echocardiography. The application of STE in RV dysfunction in patients with acute PE has been described, but the behavior of 3D RVEF in this setting has not been reported. We sought to assess the clinical utility of 3D echocardiography and speckle-tracking imaging in patients with acute submassive PE at time of diagnosis and after short-term and long-term follow-up.
Methods
Population
We prospectively studied 66 patients who had the diagnosis of acute submassive (hemodynamically stable) PE using personal histories, physical examinations, echocardiography, lower limb venous ultrasonography, and laboratory tests. PE diagnosis was confirmed by the presence of thrombi in the pulmonary arteries on thoracic computed tomography, in line with current European Society of Cardiology guidelines. Submassive or intermediate-risk PE was defined as RV dysfunction without systemic hypotension. Patients with at least one of the following echocardiographic findings were diagnosed with acute RV dysfunction: (1) RV hypokinesia assessed by qualitative interpretation of systolic RV free wall motion, (2) RV dilatation (end-diastolic diameter > 30 mm in the apical four-chamber view), and (3) paradoxical septal systolic motion. Exclusion criteria were moderate or greater valvular disease, chronic obstructive pulmonary disease or congestive heart failure, a prior documented episode of PE, comorbidity with life expectancy < 6 months, and insufficient ultrasound image quality (defined as more than three myocardial segments that were not optimally visualized using conventional two-dimensional echocardiography). Transthoracic echocardiography was performed at the onset of the acute episode and after median follow-up periods of 30 days and 6 months. Information about symptoms and functional class (according to the New York Heart Association) was collected. Written informed consent was obtained. Adverse clinical outcome was defined as the occurrence of one or more of the following: death, cardiopulmonary resuscitation, and acute PE recurrence. These adverse events were selected on the basis of the Management Strategies and Prognosis of Pulmonary Embolism 3 trial. Sixty-six healthy age-matched and sex-matched adults with no histories of cardiopulmonary disease, no known coronary risk factors, and normal electrocardiographic and echocardiographic results were selected as a control group.
Standard Echocardiography
All patients underwent transthoracic echocardiography using a commercially available cardiovascular ultrasound system (Vivid E9; GE Vingmed Ultrasound AS, Horten, Norway). Established criteria were used for measurement of the right chambers. The RV–to–left ventricular (LV) end-diastolic diameter ratio was calculated in the apical four-chamber view. RV end-diastolic area and end-systolic area were assessed using manual planimetry and RV fractional area change (FAC) was derived. Tricuspid annular plane systolic excursion (TAPSE) was measured using apical views adjusted to optimize RV structures and to achieve proper orientation for M-mode measures. RV myocardial performance index (MPI) was determined as the sum of the isovolumic contraction time and isovolumic relaxation time divided by the ejection time. Pulmonary acceleration time was calculated as the interval between the onset of ejection and peak pulmonary flow velocity from the parasternal short-axis view. RV systolic pressure (RVSP) was obtained by continuous-wave Doppler echocardiography. A systematic search was performed using two-dimensional and color flow Doppler to identify the most complete tricuspid regurgitation (TR) jet followed by continuous-wave Doppler acquisition of spectral envelopes of the greatest maximal velocity and density. Right atrial pressure was estimated by visualizing the inferior vena cava (IVC) diameter from long-axis subcostal views and its phasic response to respiration. Right atrial pressure was estimated as 5 mm Hg if the IVC was <2.0 cm in diameter at the junction of the right atrium, 15 mm Hg if the IVC was dilated and collapsed with respiration (>50%), and 20 mm Hg if the IVC was dilated and did not collapse with respiration. Pulmonary arterial hypertension (PAH) was classified according to baseline RVSP as mild (40–49 mm Hg), moderate (50–59 mm Hg), or severe (≥60 mm Hg). Normalization of pressures was defined as RVSP < 40 mm Hg. Estimation of pulmonary vascular resistance (PVR) was determined on the basis of the ratio of peak TR velocity to RV outflow tract time-velocity integral that was shown to be a good correlate of PVR despite reflecting pulmonary systolic pressure and not the transpulmonary pressure gradient. The peak TR velocity (meters/second) was determined using continuous-wave Doppler using the highest velocity obtained from multiple views. The RV outflow tract time-velocity integral (centimeters) was obtained by placing a 1-mm to 2-mm pulsed-wave Doppler sample volume in the proximal RV outflow tract just within the pulmonary valve when imaged from the parasternal short-axis view. PVR was calculated using the following equation: PVR (Wood units) = 10 × TR velocity/RV outflow tract time-velocity integral.
Tricuspid annular systolic velocity was measured at the lateral corner of the tricuspid annulus using apical views adjusted to optimize RV structures and to achieve proper orientation for Doppler measures.
McConnell’s sign was defined as reduced RV free wall contraction with preserved apical wall motion on two-dimensional echocardiography, and those signs were read blinded.
3D Echocardiography
Three-dimensional echocardiography was done using a dedicated broadband, wide-angle, matrix-array transducer to enable full coverage of the entire right ventricle by the pyramidal volume, with particular attention to the upper anterior wall and RV outflow tract. Before each acquisition, the images were optimized for endocardial border visualization by increasing the overall gain and modifying the time gain and compression. Echocardiographic images were stored digitally for offline analysis using TomTec software (TomTec Imaging Systems, Unterschleissheim, Germany). Within the 3D data set, three orthogonal main cut planes were selected to define the end-diastolic and end-systolic frames within the sequence as well as several landmarks. On the basis of the initial view adjustment and the landmarks, the program automatically provides four-chamber, sagittal, and coronal RV views. RVEDV and RV end-systolic volume (RVESV) were measured from each 3D echocardiographic data set. Tracing was done through the bases of the papillary muscles. RVEF was calculated as follows: RVEF = [(RVEDV − RVESV)/RVEDV] × 100 ( Figure 1 ). The process of volume determination was done two times for each patient.

STE
The general principles that underlie the speckle-tracking echocardiographic modalities have been previously described. The acquisitions were obtained successively for all patients by the same physician, with analyses being performed retrospectively and offline. Apical four-chamber views were used, and special care was taken to ensure an adequate field of view to image the entire right ventricle by narrowing the two-dimensional sector width or positioning the transducer down an intercostal space and laterally. Two-dimensional peak systolic RV longitudinal strain (RVLS) was measured adopting a six-segment RV model (basal free wall, mid free wall [MFW], apical free wall, apical septum, mid septum, and basal septum). Peak systolic strain was recorded for the three RV myocardial free wall and septal segments and the entire RV wall ( Figure 1 ). RV dyssynchrony was quantified using the standard deviation of the heart rate–corrected intervals from QRS onset to peak systolic strain for the six segments. The echocardiographic results were analyzed by an echocardiologist blinded to the clinical data, using dedicated software (EchoPAC BT11, 4D Auto LVQ; GE Vingmed Ultrasound AS, Horten, Norway).
Statistic Analysis
Data are expressed as mean ± SD. Student’s t test was used for comparisons of normally distributed data. Univariate and multivariate Cox proportional-hazards regression models were used to calculate hazard ratios and 95% confidence intervals for different variables. Multivariate analyses were performed using a stepwise forward regression model in which each variable with a P value ≤ .10 on univariate analysis was entered into the model. Variance inflation factors were used to identify collinearity among explanatory variables. The cutoff values of the investigated parameters enabling the prediction of adverse events with the highest sensitivity and specificity were identified by means of receiver operating characteristic curves of different indexes. Statistical significance was set at P < .05. To analyze intraobserver variability, measurements of parameters were made at multiple sites in 15 different patients on two different occasions. For interobserver variability, a second investigator randomly made measurements at the above different sites (same recorded loops) without knowledge of other echocardiographic parameters. Intraobserver and interobserver variability was determined as the difference between the two sets of observations divided by the mean of the observations and expressed as a percentage.
Results
Sixty-six of 76 initially evaluated patients with PE were included in the study. Three-dimensional echocardiographic images of the right ventricle were successfully analyzed in 72 patients (95%). Longitudinal two-dimensional RV strain measurement from all segments was possible in 73 patients (96%). TR to estimate right-sided pressure was found in 71 patients (93%). The intraobserver and interobserver reproducibility of 3D and strain parameters was shown to be acceptable. Intraobserver variability was 4.3 ± 2.3% for 3D RVEDV, 5.3 ± 2.7% for 3D RVESV, 11.8 ± 4.1% for global RVLS, and 7.6 ± 3.1% for MFW RVLS. Corresponding values of interobserver variability were 4.9 ± 3.6%, 7.8 ± 3.9%, 12.4 ± 4.9%, and 8.7 ± 3.2%.
Baseline Characteristics
The baseline characteristics of all patients with PE and of those with and without adverse events are given in Table 1 . There were no significant differences between groups with regard to baseline characteristics except for elevated troponin. Troponin I levels were elevated in 42% of patients. Sixty-four percent ( n = 42) of the patients had limitations of functional capacity (New York Heart Association functional class ≥ II). Fourteen patients (21%) had tachycardia (heart rate ≥ 100 beats/min). Eighteen patients had adverse events. Thirty-day and 6-month all-cause deaths occurred in four and nine patients, respectively. Cardiopulmonary resuscitation was performed in two patients. Three patients had acute PE recurrence.
Variable | Total ( n = 66) | With adverse events ( n = 18) | Without adverse events ( n = 48) | P |
---|---|---|---|---|
Age (y) | 53 ± 11 | 54 ± 18 | 52 ± 14 | .078 |
Men/women | 32/34 | 8/10 | 22/26 | .426 |
BSA (m 2 ) | 1.82 ± 0.13 | 1.87 ± 0.16 | 1.85 ± 0.14 | .377 |
SBP (mm Hg) | 118 ± 10 | 113 ± 9 | 115 ± 8 | .114 |
DBP (mm Hg) | 77 ± 6 | 77 ± 8 | 78 ± 5 | .251 |
HR (beats/min) | 89 ± 5 | 90 ± 5 | 86 ± 7 | .432 |
Respiratory rate (breaths/min) | 23 ± 5 | 25 ± 8 | 22 ± 7 | .597 |
Tachycardia (>110 beats/min) | 14 (21%) | 4 (22%) | 10 (20%) | .323 |
NYHA functional class ≥ II | 42 (64%) | 12 (67%) | 30 (63%) | .426 |
Troponin I (ng/mL) | 0.06 ± 0.02 | 0.09 ± 0.03 | 0.035 ± 0.016 | .029 |
d -dimer (μg/mL) | 7.21 ± 6.13 | 8.07 ± 4.92 | 7.89 ± 5.11 | .216 |
Arterial oxyhemoglobin saturation < 90% | 18 (27%) | 5 (28%) | 13 (27%) | .528 |
DVT | 32 (48%) | 8 (44%) | 24 (50%) | .179 |
Comorbidities | 14 (21%) | 4 (22%) | 10 (20%) | .327 |
Thrombolysis | 7 (11%) | 3 (16%) | 4 (8%) | .166 |
Embolectomy | 2 (3%) | 1 (5%) | 1 (2%) | .315 |
Echocardiographic Parameters
Overall, 3D RVEFs were significantly reduced compared with controls ( Table 2 ). TAPSE and FAC decreased and MPI increased significantly. Global RVLS was significantly lower than in the control group. Speckle-tracking strain was especially reduced in the RV MFW and basal free wall segments ( Table 2 ). There was a direct correlation between MFW RVLS and 3D RVEF and a negative correlation between MFW RVLS and RV dyssynchrony ( Figure 2 ).
Variable | Patients with PE ( n = 66) | Controls ( n = 66) | P |
---|---|---|---|
RVEDA (cm 2 ) | 30 ± 3 | 17 ± 5 | .037 |
RVESA (cm 2 ) | 20 ± 4 | 9 ± 4 | .046 |
RVFAC (%) | 32 ± 11 | 49 ± 13 | .031 |
RV/LV ratio | 1.23 ± 0.27 | 0.69 ± 0.16 | .036 |
TAPSE (mm) | 15 ± 4 | 23 ± 4 | .024 |
RV MPI | 0.56 ± 0.11 | 0.26 ± 0.06 | .032 |
TV S a (cm/sec) | 7.2 ± 3.1 | 12.7 ± 2.5 | .039 |
MS | 35 (53%) | 0 | — |
RVSP (mm Hg) | 53 ± 14 | 23 ± 3 | .011 |
PVR (Wood units) | 3.9 ± 1.8 | 1.3 ± 0.5 | .049 |
PAT (msec) | 64.2 ± 11.7 | 126.2 ± 24.4 | .032 |
3D RVEDV (mL) | 197 ± 51 | 80 ± 16 | .004 |
3D RVESV (mL) | 119 ± 29 | 35 ± 7 | .002 |
3D RVEF (%) | 37 ± 8 | 56 ± 8 | <.001 |
RV dyssynchrony (STE) (msec) | 97 ± 34 | 24 ± 15 | .041 |
Global RVLS (STE) (%) | −17.2 ± 4.5 | −24.3 ± 3.8 | .026 |
AFW RVLS (STE) (%) | −15.6 ± 5.8 | −21.5 ± 4.3 | .043 |
MFW RVLS (STE) (%) | −8.2 ± 6.6 | −25.7 ± 4.2 | <.001 |
BFW RVLS (STE) (%) | −19.1 ± 7.5 | −26.5 ± 4.4 | .019 |
McConnell’s sign (RV wall hypokinesia with apical sparing) was observed in 35 patients (53%) with acute PE and was associated with a higher RV/LV ratio (1.26 ± 0.79 vs 0.83 ± 0.12, P < .05), lower 3D RVEF (37 ± 6% vs 44 ± 7%, P < .005), and lower MFW RVLS (−13.4 ± 4.1% vs −15.6 ± 4.4%, P < .005). Global RV wall strain was not different in patients with McConnell’s sign compared with those without that sign (−18.1 ± 4.8% vs −18.9 ± 4.5%, P = .073).
Correlations with Pulmonary Hypertension and Outcome Results
Mean baseline RVSP was 53 ± 14 mm Hg, mean pulmonary acceleration time was 64.2 ± 11.7 msec, and mean PVR was 3.9 ± 1.8 Wood units ( Table 2 ). Seventy-one percent of patients ( n = 47) had mild to moderate PAH and 29% ( n = 19) had severe PAH at the onset of the acute episode. Most patients with PE had mild or minimal TR, and no patient with severe TR was observed at the time of PE diagnosis. RVSP decreased from 53 ± 14 to 47 ± 12 mm Hg at 30-day follow-up and to 31 ± 7 mm Hg at 6-month follow-up ( P < .005). Forty-three percent of patients ( n = 29) had normalization of RVSP, 21 patients with moderate PAH and four patients with severe PAH. Twenty-four percent of patients ( n = 16) remained in New York Heart Association class ≥ II. Sixty-eight percent of these patients ( n = 11) had residual PAH.
Figure 3 analyzes MFW RVLS, 3D RVEF, and RVSP in normal subjects, patients with acute PE at the time of diagnosis, and on 30-day and 6-month follow-up echocardiography. The figure depicts earlier reversal of MFW RVLS values at 30-day follow-up and longer reversal of 3D RVEF and RVSP values at 6-month follow-up.
By multivariate analysis, 3D RVEF ( P = .001) and MFW RVLS ( P = .002) were independently associated with adverse outcomes (independently of clinical characteristics, laboratory data, and comorbidities) in patients with PE ( Table 3 ). By receiver operating characteristic curve analysis, we compared the ability of various echocardiographic parameters to predict adverse clinical outcomes ( Table 4 , Figure 4 ). Cutoff points for RV FAC, RV/LV ratio, RVSP, MFW RVLS, and 3D RVEF of 37%, 1.05, 43 mm Hg, −12%, and 40% had sensitivity of 73%, 74%, 79%, 84%, and 88% and specificity of 64%, 69%, 68%, 74%, and 79%, respectively, in predicting adverse events after 6-month follow-up. On the basis of the data presented in Table 4 and Figure 4 , we selected RVSP, MFW RVLS, and 3D RVEF for assessing prognostic value. RVSP > 43 mm Hg was present in 14 of 18 patients (78%) who experienced adverse events and in 19 of 48 patients (40%) who did not experience adverse events ( P = .019). MFW RVLS > −12% occurred in 15 of 18 patients (83%) with adverse events and in 14 of 48 (29%) with no adverse events ( P = .002), and 3D RVEF < 40% occurred in 16 of 18 (89%) and 11 of 48 (23%) patients, respectively ( P = .001). The combination of all three parameters was seen in 94% of patients with adverse clinical events and 23% of patients with no adverse events ( P < .001). Values of RVSP, MFW RVLS, and 3D RVEF in patients with adverse events and without adverse events are plotted in Figure 5 (RVSP, 55 ± 13 vs 37 ± 7 mm Hg, P < .05; MFW-RVLS −7.5 ± 4.1% vs −13.9 ± 4.2%, P < .01; 3D RVEF, 34 ± 6% vs 47 ± 7%, P < .01).
Variable | HR | 95% CI | P |
---|---|---|---|
Univariate analysis | |||
Age | 1.99 | 1.54–2.91 | .005 |
SBP | 1.92 | 1.77–2.71 | .025 |
Diabetes mellitus | 1.16 | 1.01–1.76 | .014 |
Sepsis | 1.21 | 1.08–1.83 | .019 |
Renal insufficiency | 1.93 | 1.67–2.82 | .037 |
Troponin I | 2.07 | 1.15–3.24 | <.001 |
RVSP | 2.19 | 2.17–3.46 | .002 |
RV/LV ratio | 1.22 | 1.08–1.74 | .004 |
TAPSE | 1.74 | 1.53–2.86 | <.05 |
RV FAC | 1.98 | 1.63–2.95 | <.01 |
MFW RVLS | 2.27 | 1.49–3.92 | <.0005 |
3D RVEF | 4.32 | 2.43–8.09 | <.0001 |
Multivariate analysis | |||
Troponin I | 1.93 | 0.91–2.79 | .008 |
RVSP | 2.14 | 1.12–2.91 | .007 |
MFW RVLS | 2.95 | 1.31–3.23 | .002 |
3D RVEF | 3.51 | 1.66–4.51 | .001 |
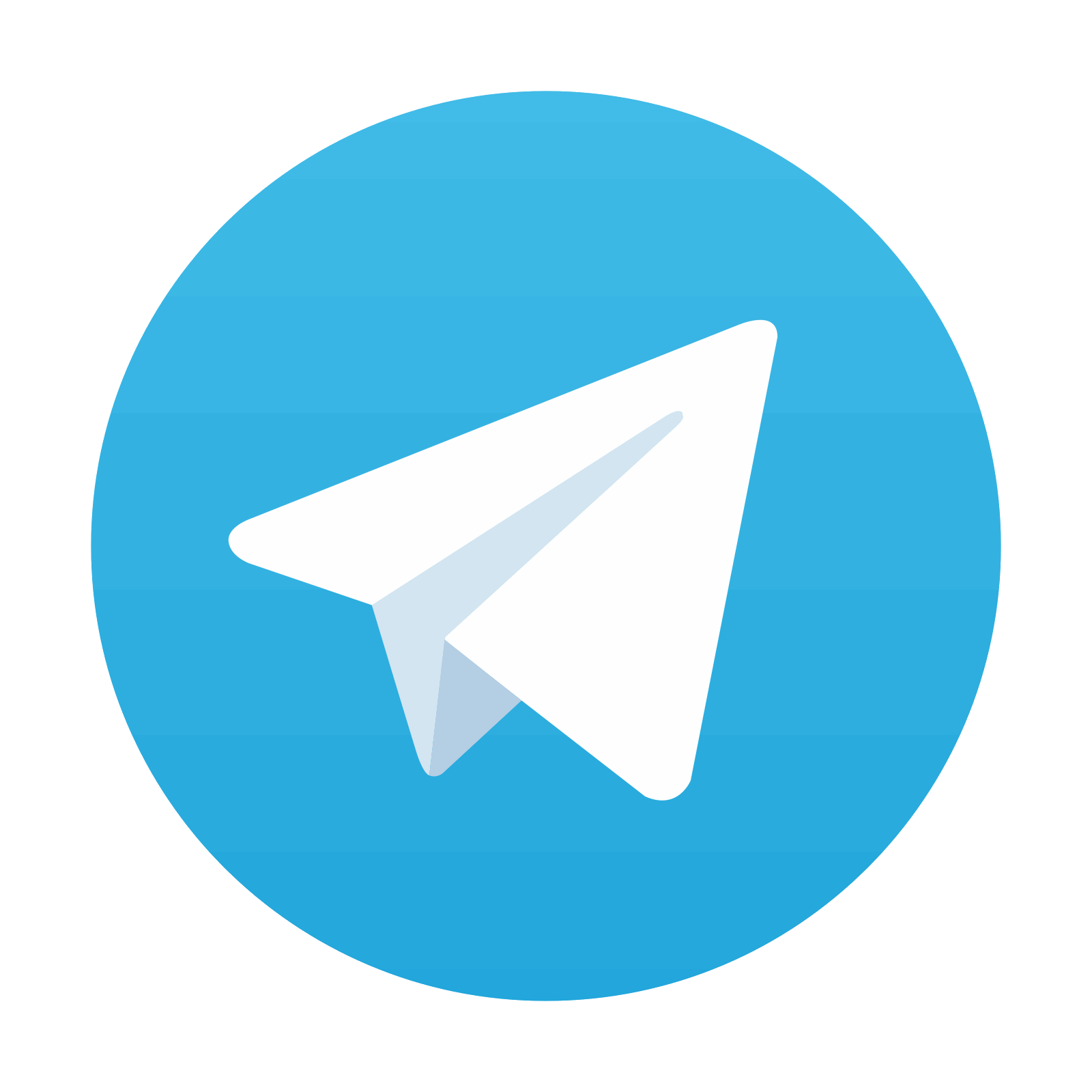
Stay updated, free articles. Join our Telegram channel

Full access? Get Clinical Tree
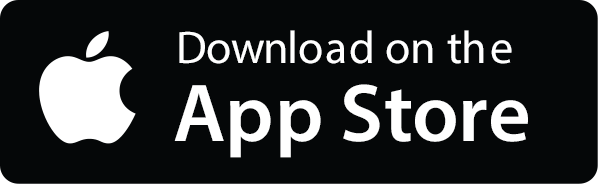
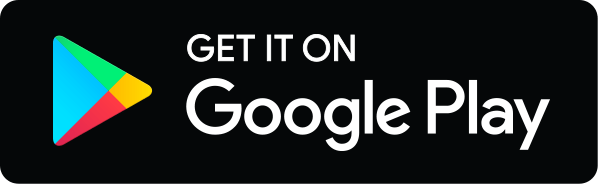
