Fig. 7.1
Acute effect of pulmonary artery banding (PAB) in an infant with LV-DCM. (a) TEE (intraoperative transesophageal ECHO) shown is the four-chamber view in an infant with an extreme dilated left ventricle (LVEDd left ventricular enddiastolic diameter) and its compression of right ventricle (RV) before surgical PAB; (b) TTE (transthoracic ECHO) short axis view shows the PAB and the pulmonary valve (PV); (c) TEE (intraoperative) depicts the acute repositioning of interventricular septum (IVS) immediate after banding based on the RV pressure increase, LV volume, and preload reduction as well as improved filling of the pressure loaded RV. Not shown is the pressure gradient reduction across the PFO caused by the PAB-induced left ventricular preload reduction
Best summarized is the novel PAB strategy by an editorial comment of LL Bailey [36]; “done with care, in properly selected candidates, rPAB appears to shift the ventricular septum leftward, thereby reducing left ventricular (LV) end-diastolic volume and causing the LV to fall within the Frank-Starling curve, where LV reverse remodeling may occur and LV ejection fraction increases; this might reduce LV filling dynamics and end-diastolic pressure and restore ventricular electromechanical synchrony.” In addition, the use of rPAB seems to be especially attractive in very young infants, whose potential for myocyte recovery and repopulation seems greatest [37–39]. The neonatal myocardium is known to contain three times the number of progenitor (proliferating) cells as found in the hearts of 2-year-olds and those who are older. Based on our own research, pressure overload is an additional factor, which leads to an increase of resident cardiac stem cells. Kuehn and coworkers [40] showed that the percentage of cardiomyocytes undergoing mitosis and cytokinesis was highest in infants, decreasing to low levels by 20 years, and that, between the first year and year 20 of life, the number of cardiomyocytes in the left ventricle increased 3.4-fold. They concluded, “that children and adolescents may be able to regenerate myocardium, and that abnormal cardiomyocyte proliferation may be involved in myocardial diseases that affect this cell population. These diseases might be treatable through stimulation of cardiomyocyte proliferation.” Regarding PAB in ccTGA, the adaptive ability of the sub-pulmonary ventricle is better the younger the patient’s age [33]. Independent of a sub-aortic left or right ventricle, the risk for surgical banding is lower, the younger the patient’s age, and it is highly effective in generating right ventricular adaptation to PAB without the need for re-banding. The patient should also have the chance to grow in the PAB. Acute right ventricular adaption to rPAB occurs within 3–15 days, comparable to those patients where a sub-pulmonary left ventricle is retrained [33, 34]. Regarding some of the basic research results of PAB and its effects on the right ventricle (see Chap. 13). In animal models with a non-diseased left ventricle the time course of right ventricular hypertrophy development after PAB showed an exponential rise in the RV mass during the first 3 weeks after PAB, and thereafter the RV mass reached a plateau [41]. Published research data obtained in animal studies confirm that PAB induced RV hypertrophy, which is accompanied by a slightly (almost 10–20 %) hypertrophic reaction of an unaffected healthy left ventricle [42]. Additionally, there appears to be a syncytial relationship between cardiomyocytes, fibroblasts, and the cardiac matrix. A hypertrophy-fibrosis-angiogenesis program that initially allows a compensated response of the RV to pressure overload and stretch seems to be essential for the adaptive mechanisms of the heart to pressure overload. In this context, Takeda et al. [43] have provided evidence that in particular fibroblasts are essential for the adaptive response of the heart to such pressure overload. Data from Bogaard et al. [44] have confirmed that the hypertrophic RV after chronic PAB is characterized by an increase in the size of the myocardiocytes, a cell growth-directed gene expression pattern and a maintained capillary network. During hypertrophic growth, enhanced protein synthesis leads to an increase in the individual size of the myocardiocytes, whereas de-compensation is associated with proteolysis, a switch from cell growth to cell death and replacement fibrosis.
However, in the clinical setting of reversible PAB in LV-DCM, clinical improvement corresponded with an increase of the LV-EF a decrease of LVEDD and BNP-blood levels (Video 7.2). In addition to mechanical interactions between the right and left ventricle, we hypothesize that there are also biological signal interactions between the right ventricle that undergoes hypertrophy and the improving LV [45].
The reversibility of the PAB is based on the specific surgical technique, which allows percutaneous de-banding by a balloon dilation procedure with or without residual pressure gradient [12]. Nevertheless, multicenter investigations are yet to show whether the concept of rPAB leading to LV recovery can be expanded to older children and whether rPAB diminishes the need for mechanical circulatory assist devices and heart transplantation in the management of pediatric heart failure. Such studies are necessary in order to assess long-term risks and benefits of the reported surgical approach and to elucidate the mechanistic interactions between the hypertrophying right and dilated and dysfunctional left ventricle in patients with LV-DCM. Bailey stated [36] “PAB in LV-DCM might have the potential of a game changer if the approach is proven and based on a percutaneous procedure” [36].
The Right Ventricle in the Context of Restrictive Cardiomyopathy and Borderline Left Ventricle: Heart Failure with Preserved Ejection Fraction
In contrast to DCM, RCM or a BLV is primarily associated with a diastolic dysfunctional circulation [46, 47].
Heart failure with preserved left ventricular ejection fraction is attributed to “abnormalities of diastolic function” [48]. Diastolic dysfunction with preserved left ventricular ejection fraction is relatively rare but already present in a newborn, child, or young adult, often associated with congenital or acquired myocardial fibrosis (RCM) or a structural “borderline left ventricle” with or without additional endocardial fibroelastosis [49, 50].
The entity of a too small ventricle as well as structural left heart diseases like pulmonary vein stenosis, total anomalous venous return, mitral or aortic valve diseases and aortic arch obstruction as a single disease or as a multiple structural abnormalities disease (Shone complex), have the potential to be associated with an out-of-proportion pulmonary hypertension and subsequent right heart failure [51].
The Right Ventricle in Context of Borderline Left Ventricle
Borderline and hypoplastic left heart structures are defined by z-score values more than −2 SD deviating from the norm (Video 7.3). From the pathophysiological point of view this means that the filling of the ventricle is limited, the capacity of the end-diastolic volume at rest or during exercise is diminished and the end-systolic volume is minimized [51]. Therefore, the ventricular compliance is reduced by its inability to relax, but the systolic function. The therapeutic strategies are limited and any surgical approach challenging. Trials to resect part of the endocardial fibroelastosis have been performed with success in some cases [52].
One therapeutic option in newborns with BLV might be a “Hybrid approach” which consists of bilateral pulmonary banding, duct stenting and, if necessary, interatrial stenting [53, 54], which gives the right ventricle the chance to work in concert with the diminutive left ventricle. The hybrid approach allows the delay of the final decision of generating a bi- or uni-ventricular circulation later, which depends on the further development of the affected left ventricle (Fig. 7.2a, b). In infancy, patients with left heart induced “out-of-proportion” pulmonary hypertension and right ventricular failure, might be helped by duct stenting, not only as a life saving approach, but even as an option for recovery and delayed surgical correction. Therefore, careful structural assessment of patients with right ventricular failure based on pulmonary vascular suprasystemic pressures is necessary in order to consider additional therapeutic strategies like stenting of a still, even minimal patent ductus arteriosus to achieve a pulmonary-to-aortic (Potts-shunt-like) communication (Fig. 7.3, Video 7.4a, b). Considering such experience in newborns and infants, “hybrid strategies” might have even survival potential in adolescent patients. Before heart–lung transplantation is considered, which is associated with high initial mortality and a limited long-term survival [55], a diastolic dysfunctional structurally left heart might be supported by creation of such a Potts-like shunt to establish a parallel circulation in part. The timing of a “Hybrid approach” in a BLV or complex structural left heart disease with dysfunctional global circulation is difficult. “Out-of-proportion” pulmonary hypertension and subsequent right ventricular failure which necessitates the listing for heart–lung transplantation might be such a criterion favoring a “hybrid approach” consisting of percutaneous atrioseptostomy, interatrial stenting, or device implantation for a definitive interatrial fenestration combined with a surgical [12, 56] or transcatheter [57] performed Potts-like shunt (Video 7.4). However, whether such a strategy will indeed improve survival with improved quality of life needs to be examined in multicenter studies. From a pathophysiology point of view left-to-right shunt at the atrial level together with a pulmonary-systemic right-to-left shunt leads to an unloading of the compromised right ventricle and an economized ventricular function by allowing both ventricles to work in a more synchronized fashion. The right-to-left shunt across the Potts-shunt offers a pop-off valve function for a suprasystemic pulmonary artery circulation, RV pressure unloading together with an improved systemic blood flow (see Chap. 5). It becomes is clear that the left ventricular preload has to be balanced by the right-to-left shunt through a restrictive Potts-shunt combined with a restricted atrial left-to-right shunt, such that systemic blood flow improves despite a reduced transpulmonary blood flow to the left atrium. Both, diminishing ventricular pressure load together with a beneficial ventricular interaction improve systemic oxygen delivery and consecutively the clinical functional class. The change of the cardiac pathophysiology into that of an Eisenmenger physiology at the arterial level does not only preserve highly oxygenated coronary and cerebral blood flow but avoids extreme oxygen desaturation of the lower body via the atrial left-to-right shunt (Chap. 5). However, creation of a Potts-shunt has been described in children with suprasystemic idiopathic pulmonary arterial hypertension (IPAH), as an alternative to lung transplantation [56], and as an alternative to an interatrial communication with right–left shunt provoking a global body cyanosis (see Chap. 5). This surgical procedure requires the construction of an anastomosis between the left pulmonary artery and the descending aorta allowing right-to-left shunting and leading to a decompression of the failing RV without provoking oxygen desaturation in the upper part of the body. In one of our functional class IV patients, a repertoire of surgical-interventional procedures became necessary to treat life-threatening hemoptysis associated with suprasystemic pulmonary hypertension ([13], see Chap. 5).



Fig. 7.2
(a) MRI 4-chamber view of newborn with borderline left ventricle (LV), the right ventricle (RV) is apex forming, postnatally the patient has a duct-dependent systemic blood flow guaranteed by the RV function and persistent pulmonary hypertension; (b) Duct stenting and bilateral pulmonary banding allows survival without prostaglandin infusion, protection of the distal pulmonary vasculature by flow and pressure reducing and gives the chance for further LV developing by preserving sufficient preload and the decision for later bi- or univentricular repair (Cartoon is a courtesy by Hakan Akintürk)

Fig. 7.3
Angiography in lateral 90° view demonstrates a right-to-left shunt through a stented (4 × 12 mm Coronary stent) duct in an infant, who was referred after resuscitation due to suprasystemic pulmonary hypertension and right ventricular failure
Considering the current interventional-surgical Hybrid techniques, we want to hypothesized, that Eisenmenger patients due to intracardiac right–left shunts with full body cyanosis (which includes the coronary as well as cerebral perfusion) might benefit from an intracardiac repair together with a performed reverse Potts-shunt; depending on the systolic and diastolic pulmonary-to-systemic pressure ratio, a valved conduit might be favored as a Potts-shunt (Fig. 7.4).


Fig. 7.4
Cartoon of a valved Conduit between left pulmonary artery (LPA) and descending aorta (DAO) as an additional option to repair intraoperative shunt with “fixed” pulmonary vascular resistance. Hypothesis: change of total body cyanotic type I Eisenmenger-syndrome to a lower body “harlekin” Eisenmenger type II physiology by contemporary avoiding of suprasystemic pulmonary hypertension in case of an inappropriate intracardiac shunt (VSD) closure and established Potts-shunt. The valve should avoid diastolic left–right shunt
The Right Ventricle in Context of a Restrictive Cardiomyopathy
RCM is a rare form of childhood cardiomyopathy, accounting for 2.5 to 5 % of all of the idiopathic cardiomyopathies [58]. Histologic abnormalities are often present, depending on the etiology of the RCM. Endomyocardial biopsy findings include myocyte hypertrophy, myofibrillar disarray, and interstitial fibrosis. RCM is characterized by impaired diastolic function with relatively preserved systolic function and right ventricular wall thickness [59]. Normal systolic function and equalization of increased ventricular end-diastolic pressures lead to abrupt premature cessation of ventricular filling in early diastole. Therefore, ventricular filling is limited to early diastole. The decreased compliance of the ventricle induces atrial dilation and raises the pulmonary vascular resistance [60]. Therefore, PAPs are usually elevated. A few small number case series have been published documenting the clinical course after the diagnosis of childhood RCM [61, 62]; all have reported an accelerated deterioration in comparison with that seen in adults. However, the natural history of the disease is not predictable and a rise of the pulmonary vascular resistance and clinical deterioration unfolded at different rates. No consistent risk factors predictive for progression have been identified [58]. The natural history of RCM varies and is at least partially dependent on the etiology, if any is identified. The mortality of children with idiopathic RCM is high, particularly in the group not undergoing heart transplantation. Rates have been reported to be as high as 63 % within 3 years of diagnosis and 75 % within 6 years of diagnosis [59]. The actuarial survival range is 44–50 % at 1–2 years after presentation. The survival decreases to 29–39 % at 3–5 years after presentation [62]. Children with RCM are at a higher risk for the development of pulmonary hypertension than children with dilated or hypertrophic cardiomyopathy. Patients with RCM and a relatively well-preserved clinical functional status remain stable despite already excessive systolic PAPs. Therefore, risk stratification and the decision to list a patient for heart transplantation is problematic. Invasive hemodynamic assessment is helpful for decision making. As mentioned above, we recommend the use of the TPG (the difference of diastolic pulmonary blood pressure and pulmonary capillary wedge pressure, left atrial and/or left ventricular enddiastolic pressure) as an additional valuable parameter for decision making of the timing of heart vs. heart–lung transplantation. Based on our institutional experience, a non-proofed cut-off value in children might be a difference (PAPd-PCWP/LAP) of less than 15 mmHg. Currently, the therapy for idiopathic RCM is limited to symptomatic treatment and does not improve outcome. However, many of the adult patients but even children are not sufficiently treated by targeted medication; most receive only diuretics [58]. Considering that controlled studies of medical treatment of children and young adults with RCM and subsequent pulmonary hypertension are missing, we favor pathophysiology and pharmacology-based medical treatments. We hypothesize that sympathetic overdrive, renin-angiotensin, endothelin- and aldosterone as circulating as well as cardiac tissue specific agonists or antagonists might be responsible for the progression of a genetically anchored myocardial fibrosis. We have treated several patients, on single case and compassionate basis without a control group. Based on our anecdotal experience, a cocktail of specific antagonists (β-AR, AT-II, ETI, and aldosterone) starting each drug in very low, but slowly increasing dosages to avoid hypotension continues to be our first therapeutic goal. We avoid as always-possible diuretics in chronic treatment of DCM, and in particular of RCM; preserving a sufficient blood pressure not at least for introducing the abovementioned antagonists in sufficient dosages but even not to activate the neurohumoral axis further. We are convinced, that such more cardiac targeted drug treatment may also induce a degree of cardiac re-remodeling. Certainly, controlled studies are needed to support our anecdotal experience. Currently, surgical options in children with RCM are limited to heart transplantation [59, 63]. However, in pure left-sided RCM “interventional, transcatheter or even surgical-interventional hybrid” strategies can be an additional option in selected cases before cardiac transplantations is considered. In patients with isolated left ventricular RCM or patients with left ventricular diastolic dysfunction there may be a benefit from a defined interatrial communication (PFO-like) Atrioseptostomy (Video 7.5a, b) with or without implantation of a fenestrated atrial septum defect occluder or a diastolic dysfunctional device (Video 7.6) should allow a defined, restrictive left-to-right shunt to reduce an “out-of-proportion” left atrial pressure. The global hemodynamic function should improve; the secondary pulmonary hypertension decrease and atrial fibrillation might be avoided by a reduced left atrium pressure and consecutive enlargement (see Video 7.4). Taken together, the need of an increased demand in left ventricular preload has to be considered and therefore preserved. Additionally, and even based on our experience in BLV, the presence of an isolated left-sided RCM with suprasystemic pulmonary hypertension and already started right heart failure might benefit from a hybrid like approach consisting of an interventional performed PFO-like interatrial communication together with a surgically created reversed Potts-shunt; this in particular in patients in whom a heart–lung transplantation is the only alternative.
In general, special attention must be paid to a pre-transplant pulmonary hypertension; it needs to be differentiate between a genuine out-of-proportion pulmonary hypertension by considering the diastolic PAP (see above), a fixed and/or may be still responsive PH. In doubt, a left ventricular assist device combined with specific PH -in particular continuous prostanoid infusion – has to be used to differentiate between a still reactive and fixed pulmonary vasculature. Considering our own and the heart transplant results of others [62], children and young adults suffering RCM might be benefit from immediate and elective ECMO use after cardiac transplantation to prevent post-transplant graft failure. Such a prophylactic strategy might be effective to bridge the vulnerable period immediate after the cardiopulmonary bypass and inflammation-induced endothelial stunning in particular of the pulmonary circulation. In addition, if pulmonary hypertension persists, creation of a small ASD in the donor heart may be of benefit to decompress the right heart in case of a postoperative pulmonary hypertensive crisis. Susceptibility of the host pulmonary circulation together with the 3-to-5 days lasting (endothelial, endocardial, myocardial) stunning period, are risk factors for right ventricular failure, as the unprepared donor heart reacts to the acute afterload increase. Such unloading and an adaptation period for the lung circulation by ECMO appear to be effective in younger patients [63]. However, there remains an increased risk of death from right heart failure after orthotopic cardiac transplantation, sometimes for weeks and months. By utilizing modern pharmacological PH treatment, acute right heart failure can be avoided and a better outcome of heart instead heart–lung transplantation might be achieved.
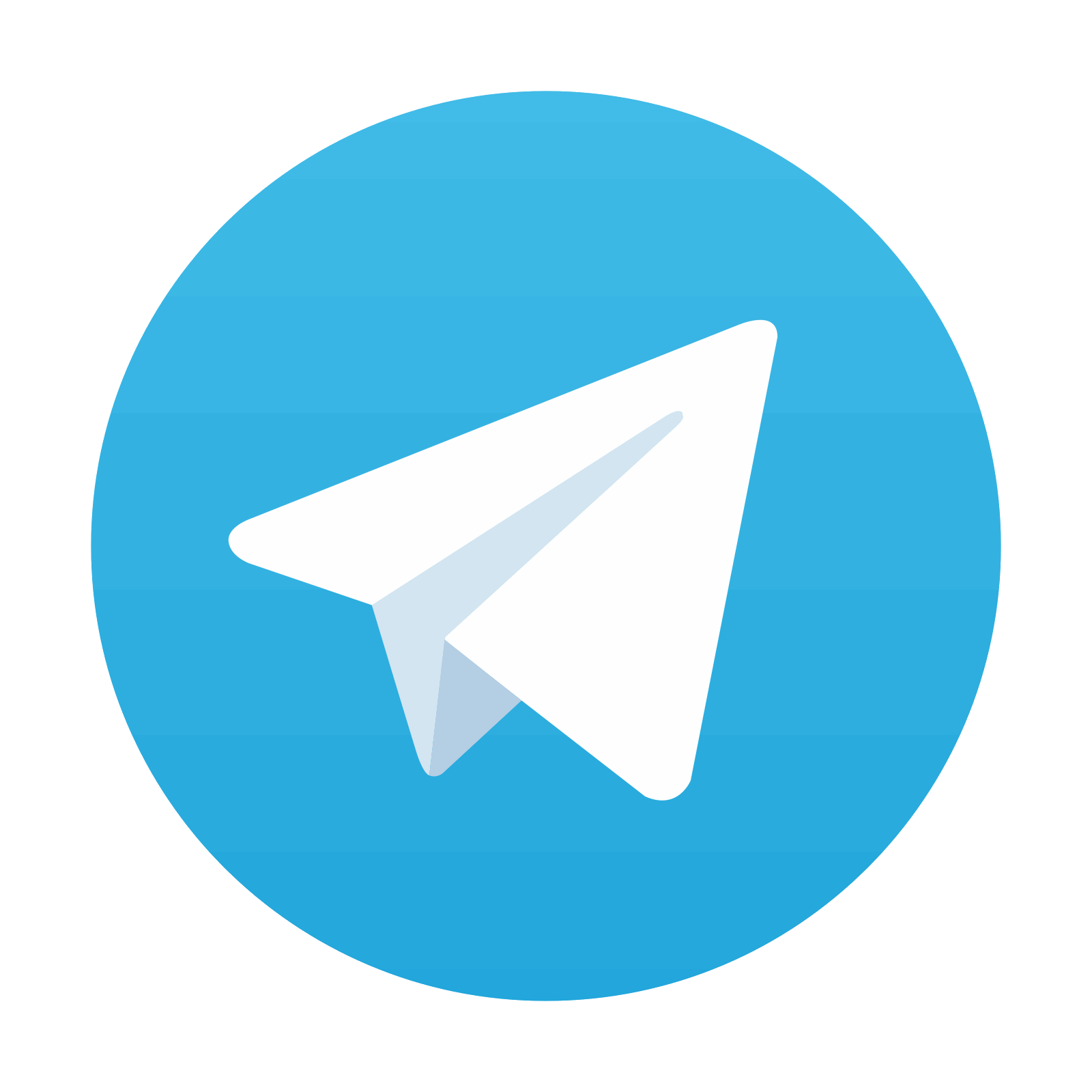
Stay updated, free articles. Join our Telegram channel

Full access? Get Clinical Tree
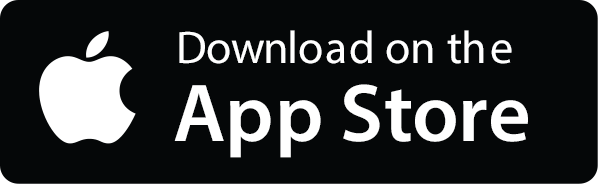
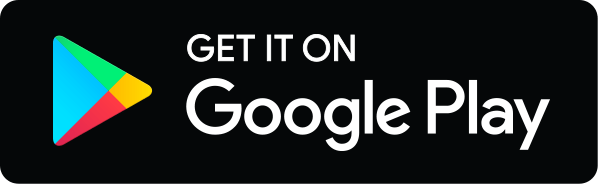