Fig. 11.1
The developing mammalian heart is derived from progenitors that emerge from the primary and secondary heart fields . Scanning electron microscopic images of the developing mouse heart demonstrating the derivatives of the primary and secondary heart fields. Note the primary (blue) and secondary (pink) heart fields at the cardiac crescent (E7.5 to E7.75), heart tube (E8.25), looped heart (E9.5), and four-chambered heart (E10.5). The atria are derivatives of the primary heart field. oft outflow tract, lv left ventricle, a atria, rv right ventricle
The embryonic heart is derived from progenitors that emerge from the primary (posterior) and secondary (anterior) heart fields (◘ Fig. 11.1) [2, 4–6]. The derivatives of the primary heart field include the left ventricle and the atria, whereas the derivatives of the secondary heart field (SHF) include the right ventricle and the right ventricular outflow tract (OFT) [2, 5]. Previous studies by the Buckingham Laboratory showed that the secondary heart progenitors emerged from the splanchnic and pharyngeal mesoderm—ultimately, to contribute to the right ventricle, OFT, and ventricular septum [5]. Fate mapping studies demonstrated that the endocardium in the right ventricle and the OFT was also a derivative of the SHF. A number of transcriptional networks have been shown to be essential for SHF formation and their derivatives, including the LIM homeodomain protein, Islet-1 (Isl1) [4, 5, 7]. Mice lacking Isl1 are nonviable and lack a right ventricle and OFT. Likewise, Foxh1 null mice are nonviable and appear to lack a right ventricle and OFT. Similarly, the bHLH protein, Hand2, is expressed in the secondary heart field derivatives, and global deletion of Hand2 results in the formation of a single ventricle that lacks a distinct right ventricle and OFT . In addition to these networks, Mef2c, Tbx1, and Bop have also been shown to have an important role in the formation of secondary heart field derivatives [5, 7].
These studies are complemented by other transgenic studies using promoter-reporter constructs such as Nkx2-5 , which serves to map cardiac progenitors and the primary and secondary heart field and their derivatives (◘ Fig. 11.2) [2, 7, 8]. Collectively, these studies emphasize the importance of chamber-specific networks that govern progenitors from the secondary heart field and that perturbations of these networks contribute to congenital heart defects. These studies also emphasize the distinct nature of the developing right ventricle as well as the molecular and structural differences between the ventricular chambers.


Fig. 11.2
Nkx2-5 is expressed in cardiac progenitors of the developing heart. Using a transgenic strategy, the Nkx2-5 upstream enhancer (6Kb fragment) was fused to a minimal promoter (Hsp68 or heat shock factor68) and the EYFP reporter to mark the progenitors associated with the cardiac crescent (E7.75), heart tube (E8.5), and looped heart at E9.5
The Right Ventricle
The right ventricle is a thin-walled, crescent-shaped, compliant chamber (◘ Fig. 11.3). Its workload is significantly less as compared to the left ventricle, as the right ventricle pumps blood to the low-resistance and highly compliant pulmonary circulation [9]. In addition, the right ventricle behaves more like a volume pump rather than a pressure pump because of its lower volume-to-surface area ratio. Therefore, the right ventricle cannot handle an acute increase in pressure overload (acute pulmonary embolism). Right ventricular stroke volume has been shown to decrease rather linearly with an acute increase in the afterload. In contrast, persistent pressure overload secondary to chronic pulmonary hypertension (PH) leads to right ventricular hypertrophy and enlargement (◘ Fig. 11.3).


Fig. 11.3
Right ventricle in normal health and pulmonary hypertension. (a) The normal, healthy right ventricle is thin walled and crescent shaped. (b) Pulmonary arterial hypertension (PAH) increases right ventricular afterload , leading to compensatory hypertrophy and, eventually, dilatation. In the presence of pulmonary hypertension, the right ventricle becomes ellipsoidal with a flattened interventricular septum. RV right ventricle, LV left ventricle
Right Heart Failure
Right heart failure resulting from right ventricular dysfunction can be broadly categorized into three different types : increased right ventricular afterload , cardiomyopathy affecting the right ventricular myocardium, and right-sided valvular heart disease. The afterload of the right ventricle consists of a steady component and a pulsatile component. The steady component—pulmonary vascular resistance (PVR)—is the afterload faced by the right ventricle to maintain forward flow, and the pulsatile component—the pulmonary arterial compliance—is the afterload faced by the right ventricle from the pulsatility of the pulmonary artery. Pulmonary vascular resistance accounts for 75–80 % of the total right ventricular afterload , and pulmonary arterial compliance accounts for the remainder of the 20–25 % of the total right ventricular afterload [10].
Pulmonary Hypertension
Pulmonary hypertension , defined as the mean pulmonary artery pressure ≥ 25 mmHg at rest (measured invasively), is the most common cause of right ventricular failure. In the presence of pulmonary hypertension, both the steady and pulsatile afterload of the right ventricle increases. Initially, the right ventricle adapts to the increased total afterload by compensatory hypertrophy. The right ventricle’s compensatory hypertrophy decreases wall stress and increases contractility by the Anrep effect to maintain contractile function (◘ Fig. 11.3). However, over time, the right ventricle dilates due to maladaptive remodeling and eventually fails. The mechanism behind the transition from adaptive, compensatory hypertrophy to maladaptive right ventricular (RV) dilatation is unclear. Right ventricular ischemia due to reduced epicardial blood flow (as a result of reduced perfusion gradient), capillary rarefaction, neurohormonal activation, and metabolic changes with increased anaerobic glycolysis and fatty acid oxidation have been proposed. In 2013, the World Health Organization (WHO) categorized pulmonary hypertension (PH) into five major groups based on pathophysiology and the underlying mechanism (◘ Fig. 11.4).


Fig. 11.4
Fifth World Health Organization classification of pulmonary hypertension. Pulmonary hypertension is classified into five categories based on the pathogenesis and mechanism of origin. BMPR bone morphogenetic protein receptor, ENG endoglin, CAV caveolin, HIV human immunodeficiency virus, PH pulmonary hypertension
Pulmonary Arterial Hypertension: WHO Category I PH
Pulmonary arterial hypertension (PAH) , WHO category I PH, comprises a group of disorders characterized by endothelial cell dysfunction and smooth muscle hypertrophy of the small pulmonary arteries, leading to RV dysfunction, right heart failure, and eventual death [11]. Remodeling of the resistance pulmonary arteries increases pulmonary arterial pressure (PAP) and pulmonary vascular resistance. This remodeling also decreases pulmonary arterial compliance, which collectively increases the afterload of the right ventricle. PAH is defined as mean PAP ≥ 25 mmHg, pulmonary capillary wedge pressure (PCWP) < 15 mmHg, and pulmonary vascular resistance (PVR) ≥ 3 Wood units. PAH can be idiopathic, hereditable, or associated with other conditions such as connective tissue disease, congenital heart disease, portal hypertension, HIV infection, anorexigen exposure, or schistosomiasis. PAH chiefly affects the small, resistance pulmonary arteries, which develop intimal hyperplasia, medial hypertrophy, adventitial proliferation, in situ thrombosis, and inflammation [12]. Capillary-like, angioproliferative vascular channels within the lumina of small muscular arteries, referred to as plexiform lesions, are pathognomonic of PAH [12].
The pathogenesis of PAH likely involves multiple pathways rather than a single mechanism [11]. The three main pathways implicated in the pathogenesis of pulmonary vascular remodeling include the endothelin, nitric oxide, and prostacyclin pathways (◘ Fig. 11.5). Endothelial dysfunction characterized by an imbalance of vasoactive and vasodilator substances does occur in the small pulmonary arteries. Endothelin production increases, while synthesis of prostacyclin and nitric oxide decreases. Endothelin is a potent vasoconstrictor and a smooth muscle mitogen, whereas prostacyclin is a potent vasodilator that inhibits smooth muscle proliferation and has antiplatelet properties [11]. Nitric oxide, a potent vasodilator, inhibits smooth muscle proliferation and platelet activation. Thromboxane, serotonin, and vasoactive intestinal polypeptide have also been shown to be involved in the pathogenesis of PAH.


Fig. 11.5
Three major pathways implicated in the pathogenesis of pulmonary arterial hypertension (PAH). PAH is characterized by increased endothelin and decreased prostacyclin and nitric oxide in the small pulmonary arteries. ET endothelin, NO nitric oxide, sGC soluble guanylate cyclase, PDE phosphodiesterase, PG prostacyclin
PAH also causes increased proliferation and decreased apoptosis of pulmonary artery smooth muscle cells. Various potential mechanisms shown to be involved in smooth muscle proliferation include normoxic activation of two transcription factors—hypoxia inducible factor (HIF)-1 alpha and nuclear factor of activated T cells (NFAT)—as well as decreased expression of the voltage-gated potassium channels Kv1.5 and Kv2.1, increased expression of the antiapoptotic protein survivin, and increased expression of transient receptor potential channels (TRPCs). Finally, the presence of inflammatory infiltrates and activation of pro-inflammatory cytokines suggest that inflammation may play a role in the pathogenesis of PAH (◘ Fig. 11.6) [13].


Fig. 11.6
Signaling pathways proposed in the pathogenesis of pulmonary arterial hypertension. VDCCs voltage-dependent calcium channels, 5–HTP 5-hydroxytryptophan (serotonin), 5–HTR serotonin receptor, ET endothelin, ETR endothelin receptor, PDGF platelet-derived growth factor, BMPRII bone morphogenetic protein receptor, SOC store-operated calcium channels, MAPK mitogen-activated protein kinase, DAG diacylglycerol, GPCR G protein-coupled receptor, IP3 inositol n,5-trisphosphate, PIP2 phosphatidylinositol bisphosphate, PLC phospholipase C, PLCβ PLC-beta, PLCγ PLC gamma, PKC protein kinase C, RO receptor-operated calcium channel, RTK receptor tyrosine kinase, co–Smad common-mediator Smad, R–Smad receptor-activated Smad signaling pathway
Mutations in genes involved in the transforming growth factor beta (TGF-ß) pathway, bone morphogenetic protein receptor (BMPR2), activin-like kinase, and endoglin have been identified in patients with hereditable PAH [14]. Mutations in caveolin-1 and KCNK3 genes have also been identified in patients with hereditable PAH [14].
The incidence of PAH is about 2–7 cases per million; prevalence is 10–26 cases per million. Idiopathic or hereditable PAH is the most common cause of PAH, accounting for nearly 50 % of patients with PAH. Of the associated causes of PAH, connective tissue disease (mainly scleroderma) is the most common etiology [15–17]. The mean age of patients with idiopathic or hereditable PAH is 45–65 years. It predominantly affects females, with a 3:1 ratio. PAH remains a fatal disease with a relatively high mortality. In the contemporary PAH registries, 1-, 3-, and 5-year survival rates were ~85 %, 69 %, and 61 %, respectively [18–22].
Clinical presentation is marked by exertional shortness of breath —the most common presenting symptom of PAH. Other symptoms include fatigue, chest discomfort, syncope, lower extremity swelling, abdominal distension, and weight gain. On physical examination, patients can have elevated jugular venous distension (JVD) with a prominent “a” wave, a palpable right ventricular heave, a loud second heart sound, a right-sided S4, and a holosystolic murmur secondary to tricuspid regurgitation. When right heart failure occurs, patients can have a prominent “v” wave in the JVD, a right-sided S3 gallop rhythm, hepatomegaly, ascites, and lower extremity edema.
Imaging studies are used to diagnose PAH. A chest X-ray can reveal a prominent central pulmonary artery, decreased peripheral pulmonary vascular markings, and reduced retrosternal space due to right ventricular hypertrophy [13]. EKGs may show right atrial enlargement, right ventricular hypertrophy, and right axis deviation. A transthoracic echocardiogram is the screening test of choice [13], and typical findings include elevated systolic pulmonary artery pressures estimated by Doppler imaging, right ventricular enlargement and hypertrophy, flattened (D-shaped) interventricular septum, reduced right ventricular systolic function, right atrial enlargement, and right-to-left shunt. Doppler estimates of PAP can be inaccurate and should not be used to make a definitive diagnosis of PH.
Patients should be screened for human immunodeficiency virus (HIV) infection, connective tissue disease, and portal hypertension. Pulmonary function tests, overnight polysomnography, ventilation-perfusion (V/Q) scan, and computed tomography (CT) pulmonary angiogram should be performed to exclude other causes of pulmonary hypertension. Right heart catheterization is the gold standard test for diagnosing PAH [13]. It helps to confirm the diagnosis, assess severity, and test for acute vasodilator response. PAH is defined as mean PAP greater than 25 mmHg at rest with a PCWP ≤ 15 mmHg and a PVR > 3 Wood units. During diagnostic right heart catheterization, acute vasodilator challenge is usually performed to identify a subset of patients with a positive response who will respond to calcium channel blocker therapy. Cardiac magnetic resonance imaging (CMRI ) is gaining importance in the diagnostic evaluation of PH, especially to assess the functional status of the right ventricle. It provides an accurate and reproducible assessment of the right ventricular volumes, mass, and contractile function [23].
Treating patients with PAH includes general supportive measures and PAH-specific vasodilator therapy . PAH-specific therapies have been shown to improve functional capacity, quality of life, and hemodynamics, and reduce hospitalizations. PAH survival has improved significantly in the last two decades, but the exact reason for that improvement is unclear. Epoprostenol is the only drug shown to improve survival. None of the other 12 available PAH-specific therapies have been shown to improve survival.
Supportive treatment measures for PAH include supplemental nasal oxygen, diuretics for right heart failure, and long-term anticoagulation using warfarin , with a target INR of 1.5–2.5 [24, 25]. Long-term anticoagulation is recommended for patients with PAH, especially idiopathic PAH [13]. Digoxin has been shown to improve right ventricular contractility in PAH. Calcium channel blockers are indicated in patients with idiopathic PAH who have a positive response during acute vasodilator testing at the time of diagnostic right heart catheterization. Only 5–10 % of patients with idiopathic PAH will have a positive vasodilator response [15], but those who do will have a sustained reduction in pulmonary artery pressures and a better survival when treated with long-term oral calcium channel blocker therapy [26].
PAH-specific vasodilator therap ies target the nitric oxide pathway, the endothelin pathway, and the prostacyclin pathway. Phosphodiesterase 5A inhibitors (PDE5A-inhibitors) increase cyclic guanosine monophosphate, which has vasodilatory, antiproliferative, and proapoptotic effects. Soluble guanylyl cyclase stimulators increase cyclic guanosine monophosphate by stimulating the enzyme-soluble guanylyl cyclase independent of nitric oxide. Sildenafil and tadalafil are two PDE5A-inhibitors approved for patients with PAH. Both drugs reduce mean PAP, increase cardiac output, and decrease PVR associated with an increase in 6-min walk distance and improvement in functional class both with short-term and long-term therapy [27–29]. Riociguat is a soluble guanylate cyclase stimulator that increases 6-min walk distance and time to clinical worsening [30].
Bosentan, sitaxsentan, ambrisentan, and macitentan are the endothelin receptor antagonists available for treatment of PAH. Bosentan and macitentan are dual endothelin receptor antagonists that block both the endothelin A and B receptors, whereas ambrisentan and sitaxsentan are selective endothelin A receptor antagonists. All four endothelin receptor antagonists have been shown to increase 6-min walk distance, improve functional class, and increase the time to clinical worsening [31, 32]. Unlike other endothelin receptor antagonists, in a long-term, event-driven trial, macitentan was shown to reduce morbidity and mortality [33].
Parenteral prostacyclin therapy is the treatment of choice for PAH patients with WHO functional class (FC) IV symptoms. Epoprostenol requires continuous intravenous infusion because of its very short half-life of only 3–5 min, and it is unstable at room temperature. Treprostinil has a longer half-life, and it is stable at room temperature; thus, it can be administered either by subcutaneous or intravenous routes. Epoprostenol and treprostinil improve exercise capacity, hemodynamics, and quality of life, but epoprostenol is the only therapy that has been shown to improve survival in a randomized and double-blind controlled trial [34–36]. Treprostinil can also be administered by inhalation [37]. Treprostinil diolamine is a sustained release oral formulation of treprostinil and is approved as a first-line therapy in PAH patients who are not receiving any background PAH-specific therapy [38].
Lung transplantation is a potential therapeutic option for patients with PAH who do not respond to pulmonary vasodilator therapy. There is no consensus on single-lung transplant vs. double-lung transplant. In addition, patients with severe right ventricular failure may need combined heart-lung transplantation [13].
Left Heart Disease: Pulmonary Venous Hypertension (PVH) —WHO Category II PH
Pulmonary venous hypertension is defined as mean pulmonary arterial pressure ≥ 25 mmHg in the presence of pulmonary artery wedge pressure (PAWP) >15 mmHg [39]. Pulmonary venous hypertension can be associated with left ventricular systolic dysfunction, diastolic dysfunction, and left-sided valvular heart disease. Pulmonary hypertension due to left heart disease is more prevalent than other WHO categories of pulmonary hypertension [40].
Elevated left ventricular filling pressure leading to reactive pulmonary arterial vasoconstriction and pulmonary vascular remodeling results in pulmonary hypertension in left heart failure [41]. An increase in left-sided filling pressure leads to a passive increase in pulmonary artery pressure to maintain forward flow. During this initial stage, there is no structural or physiological abnormality in the precapillary resistance arterioles. Thus, transpulmonary gradient, pulmonary vascular resistance, and diastolic pulmonary artery pressure gradient remain within normal limits. This is referred to as isolated postcapillary pulmonary venous hypertension [42]. In this chapter, we will refer to this state as “postcapillary PH.” However, prolonged elevation in left-sided filling pressure causes pulmonary arterial vasoconstriction and intrinsic pulmonary arteriolar remodeling [43, 44]. This results in a disproportionate increase in pulmonary artery pressure, elevated pulmonary vascular resistance, and diastolic pressure gradient [42, 45]. The Fifth World Symposium on Pulmonary Hypertension classified pulmonary hypertension from left heart disease based on a diastolic pressure gradient as isolated postcapillary (<7 mmHg) or combined postcapillary and precapillary (≥7 mmHg) PH [46].
The prevalence of pulmonary hypertension and right heart failure is based on the underlying etiology of left heart disease (left ventricular systolic dysfunction, diastolic dysfunction, or valvular heart disease) and on the method used to diagnose PH (echocardiography or right heart catheterization). Pulmonary hypertension is more common in left ventricular diastolic dysfunction (18–83 %) compared to left ventricular systolic dysfunction (35–47.5 %) [47–55]. The prevalence of right heart failure due to pulmonary hypertension from left heart disease is not well reported. Recent data suggest that right heart failure occurs in about 30 % of patients with pulmonary hypertension due to left heart disease [50, 56, 57]. PH also commonly complicates both mitral stenosis and regurgitation [58–60]. PH is common in isolated aortic stenosis but less common in aortic regurgitation [61–64].
The presence of pulmonary hypertension and right heart failure is associated with increased mortality in patients with left heart disease regardless of the underlying etiology. Using echocardiography , the elevated (estimated) systolic pulmonary artery pressure in patients with left ventricular systolic dysfunction is associated with a higher risk of mortality, cardiac transplantation, left ventricular assist device implantation, or heart failure hospitalization [53, 54, 65]. Similar trends have been shown in patients with left ventricular diastolic dysfunction [47, 66]. The presence of right heart failure has incremental prognostic value over pulmonary hypertension for adverse outcomes [56, 65, 67–69]. The presence of pulmonary hypertension also predicts poor outcomes in patients with mitral and aortic valve disease [70–78].
Pathophysiology of PVH and Right Heart Failure
Elevated left-sided filling pressure due to restrictive physiology and functional mitral regurgitation is the major mechanism for the development of PH and subsequent right heart failure in patients with left heart disease [48, 79, 80]. Other risk factors include: polymorphism in the promoter region of the serotonin transporter gene, older age, female gender, left atrial enlargement, atrial fibrillation, and decreased pulmonary artery compliance [53, 81, 82]. Risk factors for developing right heart failure include: male gender, atrial fibrillation, the presence of coronary artery disease, lower systemic arterial pressures, and lower left ventricular ejection fraction [56].
The treatment of pulmonary hypertension and right heart failure secondary to left heart disease is mainly based on treating the underlying left heart disease process. The role of PAH-specific therapies in patients with pulmonary hypertension due to left heart disease is unclear and is currently not recommended. In addition to the lack of efficacy data, these drugs pose safety concerns as they have been associated with increased mortality in patients with left ventricular systolic dysfunction. Theoretically, these therapies could cause pulmonary edema by increasing pulmonary blood flow in the presence of elevated left-sided filling pressures [83–85]. Of all the various PAH-specific therapies, phosphodiesterase-5-inhibitors (PDE5-inhibitors) have been studied the most in PH-HFpEF (heart failure with preserved ejection fraction) [86, 87], but the results from these trials are mixed. Thus, none of the currently approved PAH-specific therapies, including PDE-5 inhibitors, have been approved for pulmonary hypertension and right heart failure in patients with left heart disease .
Cor Pulmonale : WHO Category III PH and Right Heart Failure
Cor pulmonale is defined as the combination of right ventricular hypertrophy and/or failure with pulmonary hypertension that results from lung disease, impaired ventilation, or environmental hypoxia [88]. Chronic obstructive pulmonary disease (COPD) is the most common cause of cor pulmonale. The restrictive lung diseases associated with cor pulmonale include: idiopathic pulmonary fibrosis, sarcoidosis, and connective tissue-related interstitial lung disease [88]. Other conditions associated with cor pulmonale include: obstructive sleep apnea, obesity hypoventilation syndrome, and hypoxic high-altitude sickness [88].
Cor pulmonale accounts for 10–30 % of all heart failure admissions in the United States. The estimated prevalence of cor pulmonale in patients with chronic respiratory disease varies widely across different studies based on the definition of PH, the diagnostic modality used to categorize PH, and the severity of the underlying lung disease. Dyspnea is the most common symptom of cor pulmonale, but clinical recognition is difficult as most patients with chronic lung disease often have dyspnea. The presence of other symptoms such as chest pain, lightheadedness, syncope, and worsening dyspnea may indicate the presence of cor pulmonale. Accurate diagnosis of cor pulmonale requires right heart catheterization (RHC) because Doppler-based estimation of PAP can be inaccurate, particularly in patients with chronic lung disease [89]. Importantly, the development of cor pulmonale in patients with COPD and other lung diseases is associated with increased mortality [90].
The first line of treatment for cor pulmonale is long-term oxygen therapy. Chronic alveolar hypoxia is the major determinant of pulmonary vascular remodeling, elevated pulmonary artery pressure, and increased pulmonary vascular resistance in patients with chronic lung disease [91]. Long-term oxygen therapy decreases chronic alveolar hypoxia and, ultimately, decreases pulmonary vasoconstriction and right ventricular afterload , leading to increased right ventricular contractility and increased cardiac output. The role of pulmonary vasodilators in cor pulmonale is unclear. In theory, worsening hypoxia with pulmonary vasodilator therapy is a concern due to increased ventilation perfusion mismatch [88]. Given the lack of efficacy and the theoretical risk of hypoxia, PAH-specific vasodilator therapies are currently not approved for the treatment of cor pulmonale . Digoxin can be used to increase right ventricular contractility, but the efficacy of digoxin in cor pulmonale has not been studied systematically. Diuretic therapy provides symptomatic benefit by decreasing intravascular volume and right ventricular preload. Lung transplantation is the definitive treatment for patients who are less than 65 years of age and are not inotrope dependent. Right ventricular function improves after single- or double-lung transplantation [88].
Pulmonary Thromboembolic Disease
Acute pulmonary embolism can cause acute right ventricular failure and cardiogenic shock [92]. The right ventricle is more sensitive to pressure overload than volume overload; hence, an acute increase in pulmonary artery pressure from an acute massive or sub-massive pulmonary embolism leads to reduced right ventricular contractility and right heart failure. Thrombolytic therapy, in addition to systemic anticoagulation, is recommended in patients that present with acute pulmonary embolism and severe hypotension defined as systolic blood pressure less than 90 mmHg [92]. Over time, about 5 % of patients with acute deep venous thrombosis and/or pulmonary embolism develop chronic thromboembolic disease, which can lead to chronic right heart failure due to increased right ventricular afterload [93]. Pulmonary endarterectomy is the treatment of choice for patients who have operable chronic thromboembolic disease (proximal disease) [93]. For those with distal, inoperable, chronic thromboembolic disease, riociguat, a soluble guanylyl cyclase stimulator, has been shown to improve exercise capacity and reduce clinical worsening and right heart failure-related hospitalization [94]. Percutaneous pulmonary balloon angioplasty is an alternative option for patients with inoperable chronic thromboembolic disease [93].
Right Myocardial Ventricular Infarction
Acute right ventricular myocardial infarction (MI) can lead to right heart failure. It usually occurs in the setting of inferior wall myocardial infarction, as both the inferior wall of the left ventricle and the right ventricle are supplied by the right coronary artery. Isolated right ventricular MI is extremely rare [95, 96]. The incidence of right ventricular myocardial infarction varies from 10 to 50 % in patients with inferior wall myocardial infarction. The classic clinical triad of right ventricular myocardial infarction includes hypotension, elevated jugular venous distension, and clear lung fields. Electrocardiogram reveals ST-segment elevation in the right-sided chest leads (V3R and V4R). The main line of treatment for right ventricular infarction is timely revascularization of the right ventricle, either percutaneously or surgically, as indicated [95, 96]. Cardiogenic shock due to acute right ventricular infarct responds to intravenous fluid administration, as these patients are highly preload dependent. Diuretics should be avoided. In general, regardless of revascularization, acute right ventricular myocardial infarction does not lead to chronic right heart failure unless it’s accompanied by significant pulmonary vascular disease [95, 96].
Arrhythmogenic Right Ventricular Dysplasia
Arrhythmogenic right ventricular dysplasia (ARVD ) is a hereditary progressive cardiomyopathy characterized by fibrofatty replacement of the myocardium leading to ventricular arrhythmias and progressive right heart failure [97]. While ARVD predominantly affects the right ventricle, the left ventricle can also be involved, especially in the subepicardial surface. The exact pathogenesis of ARVD is unclear, but mutations in desmosomal proteins (desmoplakin and plakoglobin) have been associated with ARVD. The prevalence of ARVD varies from 1:2500 to 1:5000, and it predominantly affects males between the second and fifth decades [97].
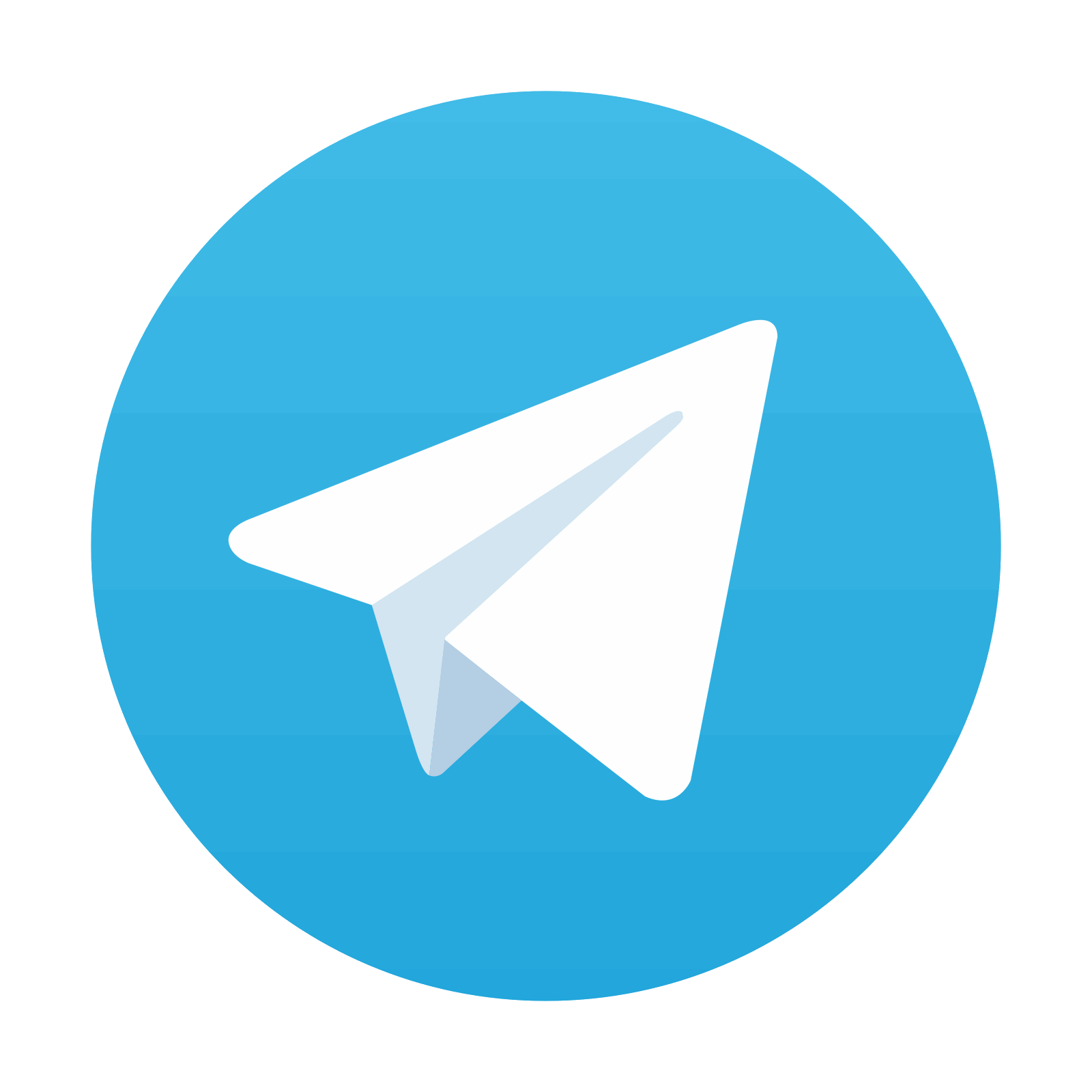
Stay updated, free articles. Join our Telegram channel

Full access? Get Clinical Tree
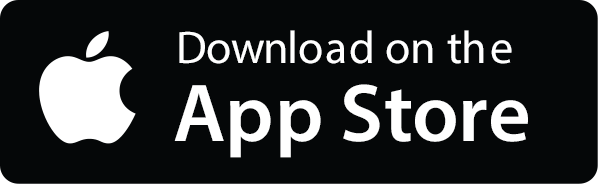
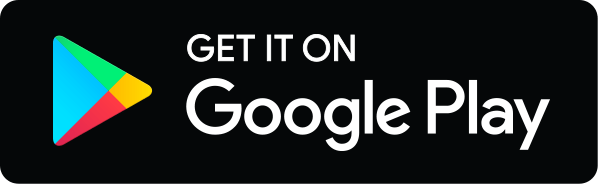