Chapter 19 Respiration in closed environments and space



The fascination of the human race with exploration has taken humans well beyond the high altitude and underwater environments described in Chapters 17 and 18. Our ability to maintain life in space, the most hostile of environments yet explored, was developed as a result of techniques used to sustain breathing in other seemingly unrelated environments on Earth. All these environments share the problems common to maintaining respiration while separated from the Earth’s atmosphere.
Closed-System Anaesthesia
This may not represent the most dramatic example of closed-environment breathing but it is by far the most common. Careful control of the composition of respired gas is the hallmark of inhalational anaesthesia. The anaesthetist must maintain safe concentrations of oxygen and carbon dioxide in the patient’s lungs, while controlling with great precision the dose of inhaled anaesthetic. It was recognised over 100 years ago that anaesthesia could be prolonged by allowing the patient to rebreathe some of their expired gas, including the anaesthetic vapour.1 Provided oxygen is added and carbon dioxide removed, other gases can be circulated round a breathing system many times, providing beneficial effects such as warm and humid inspired gas. More recently, rebreathing systems have become popular as a method of reducing both the amount of anaesthetic used and pollution of the operating theatre environment.
A totally closed system during anaesthesia means that all expired gases are recirculated to the patient, with oxygen added only to replace that consumed and anaesthetic agent added to replace that absorbed by the patient. In practice, low-flow anaesthesia, in which over half of the patient’s expired gases are recirculated, is much more commonly used.1 In each case, carbon dioxide is absorbed by chemical reaction with combinations of calcium, sodium, potassium or barium hydroxides, resulting in the formation of the respective carbonate and water. The reaction cannot be reversed, and the absorbent must be discarded after use.
Widespread use of closed-circuit anaesthesia is limited by perceived difficulties with maintaining adequate circuit concentrations of gases that the patient is consuming, such as oxygen and anaesthetic agent. However, gas-monitoring systems are now almost universally used with low-flow and closed circuit anaesthesia, allowing accurate control of circuit gas composition.
Accumulation of other Gases in Closed Circuits
Argon is normally present in air at a concentration of 0.93%. Oxygen concentrators effectively remove nitrogen from air, and so concentrate argon in similar proportions to oxygen, resulting in argon concentrations of around 5%. In a study of closed-circuit breathing in volunteers using oxygen from an oxygen concentrator, argon levels in the circuit reached 40% after only 80 minutes.2 Cylinders of medical grade oxygen and hospital supplies from liquid oxygen evaporators contain negligible argon, so the risk of significant accumulation is low.
Methane is produced in the distal colon by anaerobic bacterial fermentation and is mostly excreted directly from the alimentary tract. Some methane is, however, absorbed into the blood, where it has low solubility so is rapidly excreted by the lung, following which it will accumulate in the closed circuit. There is a large variation between subjects in methane production and, therefore, the concentrations seen during closed-circuit anaesthesia. Mean levels in the circle system in healthy patients reached over 900 ppm, well below levels regarded as unacceptable in other closed environments, but sufficient to cause interference with some anaesthetic gas analysers.3
Acetone, ethanol and carbon monoxide all have high blood solubility, so concentrations in the closed circuit remain low, but rebreathing causes accumulation in the blood. Levels achieved are generally low,3 but acetone accumulation may be associated with postoperative nausea.4 Closed-circuit anaesthesia is not recommended in patients with increased excretion of acetone or alcohol, such as uncontrolled diabetes mellitus, recent alcohol ingestion or during prolonged starvation.1
Submarines
Diesel powered. Submarines were used extensively during both world wars, and were powered by diesel engines like surface-based warships. Clearly, the oxygen requirement of the engines precluded them from use during dives and battery-powered engines were used, thus limiting the duration of dives to just a few hours. A more significant limitation to dive duration was atmospheric regulation. No attempt was made to control the internal atmosphere, and, after ventilation at the surface, the submarine dived with only the air contained within. After approximately 12 hours the atmosphere contained 15% oxygen, 5% carbon dioxide and a multitude of odours and contaminants. The need to return to the surface was apparent when the submariners became short of breath and were unable to light their cigarettes due to low levels of oxygen.5
Atmosphere regeneration.5,6 The plentiful supply of sea water and electricity make hydrolysis of water the obvious method for oxygen generation. Sea water must first have all electrolytes removed by a combination of evaporation and de-ionisation. Theoretically, one litre of water can yield 620 litres of oxygen, so, even with less than 100% efficient electrolysis, large volumes of oxygen are easily produced. Submarine atmosphere oxygen concentration is maintained at 21 ± 2%.
Atmospheric contamination during prolonged submarine patrols is well recognised, many hundreds of substances entering the atmosphere, originating from both machinery and crew. These substances include volatile hydrocarbons such as benzene, oil droplets, carbon monoxide, cadmium, and microbial organisms, with varying concentrations in different parts of the submarine. Continuous monitoring of many compounds is now routine, and maximum allowable levels during prolonged patrols are defined.7 Submarine air-conditioning units include catalytic burners that oxidise carbon monoxide, hydrogen and other hydrocarbons to CO2 and water, and charcoal absorbers to absorb any remaining contaminants. The health risks from submarine occupation are therefore believed to be extremely small.5,7,8
Physiological Effects of Prolonged Hypercapnia
Definition of a ‘safe’ level of atmospheric CO2 over long periods has concerned submarine designers for some years. The respiratory response to inhalation of low concentrations of CO2 (< 3%) is similar to that at higher levels (page 69), but compensatory acid–base changes seem to be quite different.
Respiratory changes.9,10 Atmospheric CO2 levels of 1% cause an elevation of inspired Pco2 of 1 kPa (7.5 mmHg), which results in an average increase in minute ventilation of 2–3 l.min−1. However, the degree of hyperventilation is highly variable between subjects, and presumably relates to their central chemoreceptor sensitivity to CO2 (page 69). Measurements of arterial blood gases in submariners show that the elevated minute volume limits the increase in arterial Pco2 to an average of only 0.14 kPa (1 mmHg). After a few days, the increase in ventilation declines, and minute volume returns towards normal, allowing arterial Pco2 to increase further to reflect the inspired Pco2. The time course of the decline in ventilation is too short to result from blood acid–base compensation (see below), and is believed to reflect a small attenuation of the central chemoreceptor response. On return to the surface, ventilation may be temporarily reduced following withdrawal of the CO2 stimulus.
Calcium metabolism.9,11 Elevation of arterial Pco2 causes a respiratory acidosis, which is normally, over the course of one or two days, compensated for by the retention of bicarbonate by the kidney (page 359). The changes in pH seen when breathing less than 3% CO2 appear to be too small to stimulate measurable renal compensation, and pH remains slightly lowered for some time. During this period, CO2 is deposited in bone as calcium carbonate, and urinary and faecal calcium excretion is drastically reduced to facilitate this. Serum calcium levels also decrease, suggesting a shift of extracellular calcium to the intracellular space.11 After about three weeks, when bone stores of CO2 are saturated, renal excretion of calcium and hydrogen ions begins to increase and pH tends to return to normal. Abnormalities of calcium metabolism have been demonstrated with inspired CO2 concentrations as low as 0.5%.
Some other effects of low levels of atmospheric CO2 during space travel are described below (page 307).
Space6,12–14
Space represents the most hostile environment into which humans have sojourned. At 80 km (50 miles) above Earth there is insufficient air to allow aerodynamic control of a vehicle, and at 200 km (125 miles) there is an almost total vacuum. True space begins above 700 km (435 miles), where particles become so scarce that the likelihood of a collision between two atoms becomes negligible. Even under these conditions, there are estimated to be 108 particles (mainly hydrogen) per cubic metre compared with 1025 on the Earth’s surface. Maintenance of a respirable atmosphere in these circumstances is challenging, and both American and Soviet space pioneers lost their lives during the development of suitable technology. Current experience is based on expeditions in close proximity to Earth, involving Earth orbit or travel to the moon. This means that the raw materials for atmosphere regeneration can be repeatedly supplied from Earth.
Atmosphere Composition
A summary of manned space missions and the atmospheres used is shown in Table 19.1. Spacecraft have an almost totally sealed, closed-circuit system of atmospheric control, and early Soviet space vehicles aimed to be completely sealed environments. Their designers had such confidence in the structure that emergency stores of oxygen were considered unnecessary until Soyuz 11 depressurised on re-entry in 1971, tragically killing all three cosmonauts. American Apollo missions leaked approximately 1 kg of gas per day in space, even with a lower atmospheric pressure (Table 19.1).
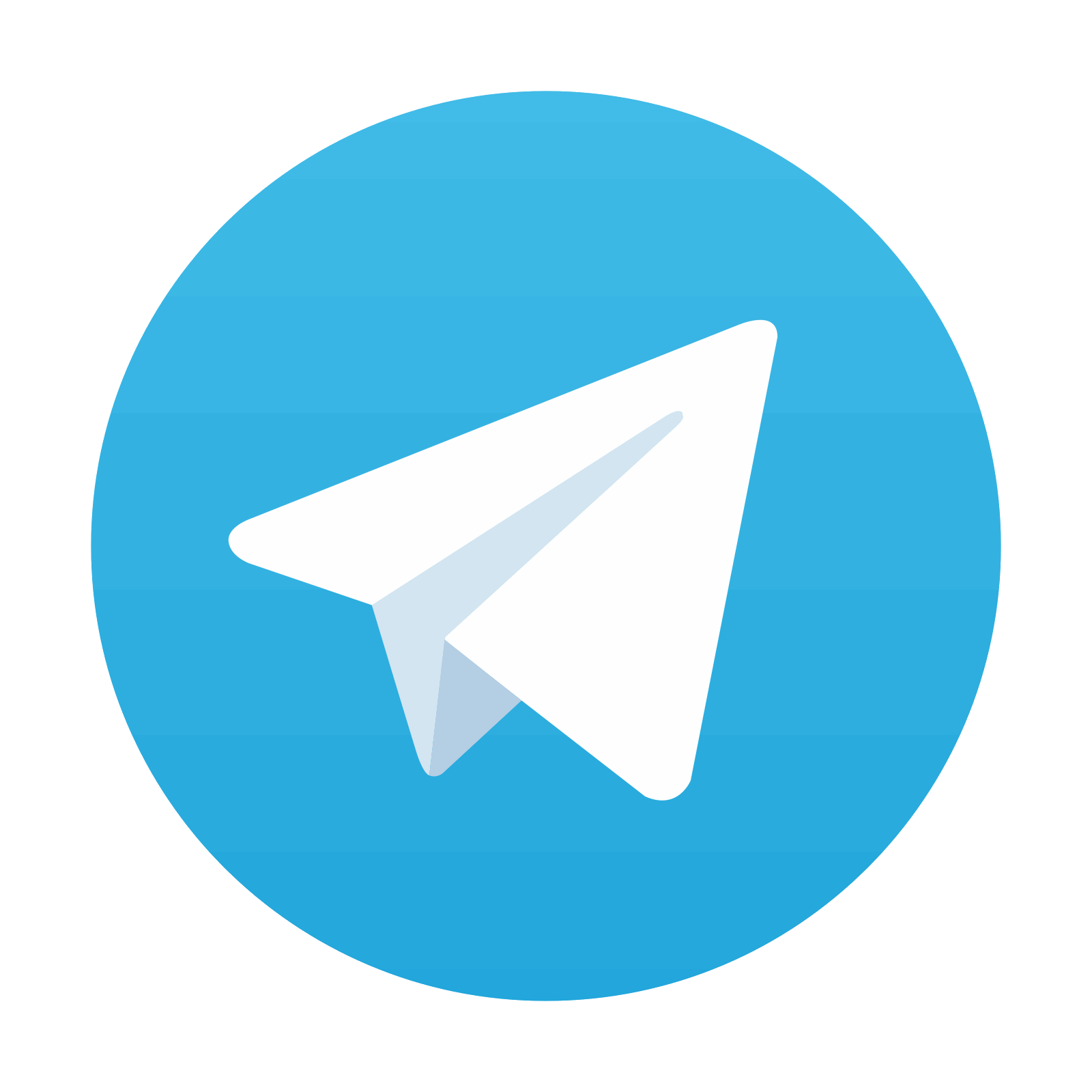
Stay updated, free articles. Join our Telegram channel

Full access? Get Clinical Tree
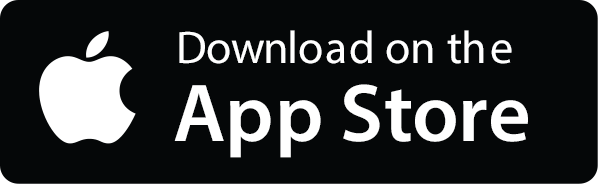
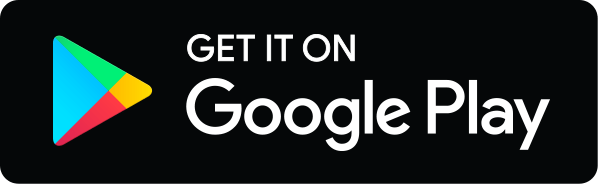