Abstract:
Research in catheterization laboratory centers around the questions involving coronary blood flow, myocardial contraction and relaxation, and pressure-flow relationships. These questions can be quantitatively addressed by a number of techniques outlined in this chapter.
Keywords:
coronary blood flow, hemodynamics, myocardial function, LV relaxation, pressure-volume relationships, Doppler coronary flow
Research techniques have been and continue to be of great value for understanding common problems in cardiology. Moreover, many of these techniques, once solely limited to the research arena, have been incorporated into routine diagnostic cardiac catheterization, such as fractional flow reserve (FFR) and intravascular ultrasound (IVUS). This chapter is an overview of commonly used research procedures in the cardiac catheterization laboratory ( Tables 10.1 and 10.2 ).
Objective | Method |
---|---|
I. Ventricular Function | |
| Ventricular P-V relationship (simultaneous LV pressure with LV volume by echocardiogram, contrast angiogram, nuclear angiogram, or impedance catheter) |
Variables derived: end-systolic P-V slope, intercept; contractility (+dP/dt) | |
| Ventricular P-V relationship (as above) |
Variables derived: end-diastolic P-V slope, intercept; relaxation (−dP/dt, τ, K) | |
| |
| |
II. Myocardial Blood Flow (Coronary Vasodilatory Reserve, Effects of Drugs) | |
Indicator dilution; inert gas (xenon, nitrogen); thermodilution | |
Doppler flow velocity | |
Digital radiographic studies | |
III. Endothelial Function | |
| |
| |
| |
IV. Electrical Function (Abnormal Conduction, Excitation) | |
Electrophysiologic studies | |
His bundle | |
Atrial and ventricular refractory periods | |
Conduction abnormalities | |
Inducible ventricular ectopy | |
Bypass tracts |
Left Ventricular Function | Methods |
---|---|
Pressure-Volume Relationships | |
End systole | High-fidelity pressure |
End diastole | LV volume LV gram (cineangiographic, digital) RV gram 2D echocardiogram Impedance catheter |
Wall Stress | |
LV mass | Quantitative ventriculography |
Diastolic function | High-fidelity pressure |
Doppler mitral inflow | |
Ventricular interaction | RV/LV high-fidelity pressures |
Aortic impedance | Aortic flow velocity, high-fidelity pressure |
Coronary Physiology | |
Coronary blood flow, coronary reserve, coronary vasodilation (response to drugs) | Pharmacologic studies with papaverine, adenosine, acetylcholine Physiologic flow responses during interventional procedures, such as angioplasty or hemodynamic studies |
Ischemia Testing | |
Induced tachycardia | Electrophysiologic study |
Isoproterenol, dopamine | Pharmacologic infusion |
Transient coronary occlusion | Coronary angioplasty |
Attitude toward research in the catheterization laboratory
The support staff in the cardiac catheterization laboratory may view research studies as unnecessary, unimportant, or dangerous to the patient. These commonly held misconceptions should be dispelled and the use and safety of the procedure advocated. It is to be emphasized, however, that only skilled physicians, with directed goals and institutional research board approval, should apply these research techniques. Discoveries that are to be made are invaluable in identifying new therapies and advancing the frontiers of treatment for cardiac disease. It is most helpful for nurses and catheterization laboratory physicians to appreciate these aspects and convey a sense of confidence and enthusiasm to the patient in reaching a common goal, that is, to improve the care and outcome of patients with heart disease.
Quantitative coronary and left ventricular angiography
Although visual estimation is universally used during angiography in the clinical setting, significant observer variability is the rule. Quantitative coronary angiography (QCA) and ventriculography are used to help overcome the subjective limitations of angiographic interpretation. Because of time constraints, these methods are typically performed off-line after data acquisition.
Quantitative coronary angiography
QCA can be performed using digital (or hand-held) calipers or, more commonly, computer-generated automated edge detection systems. For exact measurements, image calibration is required from an object with known dimensions, most commonly a contrast-filled coronary catheter. The catheter image is enlarged for measurement of its diameter to generate a calibration factor (millimeters/pixel) that is used to calculate vessel lumen size. QCA software then examines brightness values in the area of interest and uses digital algorithms to calculate vessel diameter from automatic border detection from operator-selected centerlines.
Commonly measured parameters from QCA are minimal lumen diameter (MLD), reference vessel diameter, acute lumen gain (final MLD–baseline MLD) after percutaneous coronary intervention (PCI), late lumen loss (follow-up MLD–final MLD) after PCI, and percent diameter stenosis ( Fig. 10.1 ).

Limitations of QCA, which can lead to data variability, include inconsistencies in image acquisition (e.g., vessel foreshortening, different imaging planes or magnification) and frame selection and differences in vessel tone among measurements. Significant discrepancy in distances from x-ray generator to calibration device (i.e., catheter) and to coronary vessel also leads to underestimation or overestimation of measurements. Precision can be improved with use of intracoronary (IC) vasodilators for maximal vasodilatation, complete contrast filling of the artery, and identical imaging equipment and planes among measurements.
Quantitative ventriculography
Quantitative ventriculography is best performed with biplane imaging using a 60-degree straight left anterior oblique (LAO) projection and a 30-degree right anterior oblique (RAO) projection. End-diastolic and end-systolic frames of a completely opacified ventricle during a normal sinus rhythm beat are examined using the centerline chord method. In this method, chords perpendicular to a centerline in a frame halfway between end-systolic and end-diastolic images are created and then normalized to the end-diastolic perimeter. Regional wall motion is quantified based on the degree of local chord shortening (positive values = hyperkinesis; negative values = hypokinesis) ( Fig. 10.2 ).

Quantitative coronary flow
Doppler coronary flow velocities
Coronary flow reserve (CFR; maximal coronary blood flow/resting coronary blood flow) is a global measure of coronary vasodilator circulatory capacity and is affected by epicardial and microvascular circulatory abnormalities ( Fig. 10.3 ). It was historically measured by coronary sinus (CS) blood flow with the use of a continuous thermodilution technique. Currently, coronary flow is determined from IC arterial flow velocity using 0.014-inch Doppler-tipped sensor guidewires. In the Doppler technique, quantitative measurement of coronary flow is obtained from the use of pulsed sound waves (12 to 15 MHz) and measurement of the returning signal reflecting off moving red blood cells. The Doppler guidewire can also be coupled with a pressure sensor ( Fig. 10.4 ) to measure simultaneous poststenotic coronary pressure and flow.


A pressure-temperature sensor-tipped guidewire also can be used to simultaneously measure FFR (by coronary pressure) and CFR (by coronary thermodilution) with calculation of the index of microvascular resistance (IMR). Measurement of physiologic response of coronary circulation to various drugs, maneuvers, and interventions, as well as assessment of the significance of coronary obstructive lesions before and after revascularization are examples of useful applications ( Box 10.1 ). Measurement of volumetric changes in coronary blood flow can be combined with measurement of myocardial oxygen consumption (arterial and CS blood) to identify whether increases in blood flow are caused by increased myocardial oxygen demand (i.e., metabolic regulation) or pharmacologic changes independent of myocardial demand (e.g., primary artery vasodilation or constriction).
CVR assessment
Syndrome X
Transplant coronary arteriopathy
Collateral flow studies
Coronary flow research studies
Pharmacologic and endothelial function studies
Intraaortic balloon pumping
Coronary physiology of vascular disease
Ischemic test correlation
CVR, Coronary vasodilatory reserve.
a FloWire Doppler Guide Wire (Volcano Corporation, Rancho Cordova, CA)
Doppler methodology and setup
Setting up the Doppler wire system usually takes less than 10 minutes. Timing of the reflected sound waves is used to measure blood flow velocities from moving red blood cells in a sample area that is 5 mm from the tip of the wire (and 2 mm across)—far enough away so that blood velocity is not affected by the wake of the wire. The returning signal is transmitted in real time to the display console. A gray-scale spectral scrolling display shows velocities of all red blood cells within the sample volume. Key parameters are derived from automatically tracked peak blood velocities, making them less sensitive to position ( Fig. 10.5 ).

The Doppler guidewire has a forward-directed ultrasound beam that diverges in a 27-degree arc from the long axis (measured in the −6-dB round-trip points of the ultrasound beam pattern). A pulse repetition frequency of >40 Hz, pulse duration of +0.83 seconds, and sampling delay of 6.5 seconds are standard for clinical use. The system is coupled to a real-time spectrum analyzer, videocassette recorder, and video page printer. The spectrum analyzer uses online fast Fourier transformation to process the Doppler audio signals. Simultaneous electrocardiographic and arterial pressure data are also displayed ( Fig. 10.6 ). In vivo testing has demonstrated excellent correlation of the Doppler guidewire–measured velocity with electromagnetic measurements of flow velocity and volumetric flow.

Proper engagement of the coronary ostium with a guiding catheter is key to assure the administration of the entire specified amount of any drug to be given. Before the Doppler guidewire is placed into an artery, the patient should be given intravenous (IV) heparin (40 to 60 U/kg with target activated coagulation time >200 seconds). After diagnostic angiography or during angioplasty, the Doppler guidewire is passed through a standard angioplasty Y connector attached to a guiding catheter. The guidewire is advanced into the artery and beyond the target location (e.g., stenosis) by a distance equal or greater than 5 to 10 times the arterial diameter (∼2 cm). Avoid placement in any side branches. Obtain distal flow velocity data at rest and during hyperemia ( Figs. 10.7 and 10.8 ).


It is important to note that Doppler coronary velocity measures only relative changes in velocity. Volumetric coronary flow is calculated as velocity (cm/s) multiplied by vessel area (cm 2 ). For measurement of absolute blood flow, the following assumptions must be made:
- 1.
The cross-sectional area of the vessel being studied remains fixed during hyperemia.
- 2.
The vessel lumen is cylindrical with a velocity profile that is not distorted by arterial disease.
- 3.
The angle between the crystal and sample volume remains constant and <30 degrees from the horizontal flow stream.
Coronary flow reserve
Hyperemic measurements are obtained by IC injections of adenosine (30 to 50 mcg in the right coronary artery and 50 to 100 mcg in the left coronary artery). It is important to give doses high enough to induce complete vasodilation. Some protocols use ultra-high doses of adenosine (>200 mcg); however, the incremental benefit of these larger doses is unproven. Guide catheter position (i.e., avoid suboptimal selective engagement into the coronary artery and disengagement with very forceful manual injection) is critical to accuracy.
CFR is computed as the ratio of hyperemic and basal mean flow velocities. A normal CFR is >2.0. Comparisons of CFR and FFR for detection of ischemia based on noninvasive testing as a gold standard are shown in Table 10.3 .
Indication | CFR | iFR | HSRv a | FFR |
---|---|---|---|---|
Ischemia detection | <2.0 | <0.89 | >0.8 | <0.75 |
Deferred angioplasty | >2.0 | <0.89 | — | >0.80 |
Endpoint of angioplasty | >2.0 to 2.5 b | — | — | >0.90 |
Endpoint of stenting | — | — | — | >0.90 |
Measurement of collateral circulation
Coronary collateral flow can be measured with the use of the Doppler-tipped flow wire ( Fig. 10.9 ) and pressure wire. Before placing the wire in the patient, a 0.014-inch pressure wire is set at 0 (atmosphere), calibrated, and advanced through a balloon catheter, and positioned in the vessel of interest. Collateral flow is measured by simultaneous measurement of mean aortic pressure (PAo; mm Hg), coronary occlusion pressure (Poccl; mm Hg), and central venous pressure (CVP; mm Hg). Collateral flow is calculated as (Poccl–CVP)/(PAo–CVP).

Translesional hemodynamics
Although the hemodynamics of coronary flow can be assessed by Doppler flow velocity as described earlier, there are instances in which it is also important to examine translesional pressure at rest and at hyperemia.
Fractional flow reserve
In clinical practice, the ischemic potential of a questionable or intermediate (40% to 70%) stenosis can be determined by FFR. FFR is computed as the ratio of aortic pressure (from the guide catheter) to poststenotic pressure measured from a pressure guidewire placed beyond the stenosis at rest followed by hyperemia (adenosine IV infusion or IC bolus). The technique of FFR for clinical practice is described in detail in Chapter 6 .
For research into coronary hemodynamics, note that FFR can be subdivided into three components describing the flow contributions by the coronary artery, myocardium, and collateral supply. FFR of the coronary artery (FFR cor ) is defined as the maximum coronary artery flow in the presence of a stenosis divided by the theoretic normal maximum flow of the same artery (i.e., the maximum flow in that artery if no stenosis were present). Similarly, FFR of the myocardium (FFR myo ) is defined as maximum myocardial (artery and bed) flow distal to an epicardial stenosis divided by its value if no epicardial stenosis were present. Stated another way, FFR represents that fraction of normal maximum flow that remains despite the presence of an epicardial lesion. Note that at maximal hyperemia, FFR cor is about equal to FFR myo because myocardial bed resistance is minimal. The difference between FFR myo and FFR cor is FFR of the collateral flow.
The following equations are used to calculate the FFR of a coronary artery and its dependent myocardium:
FFRcor=(Pd-Pw)/(Pa-Pw)
FFRmyo=(Pd-Pv)/(Pa-Pv)
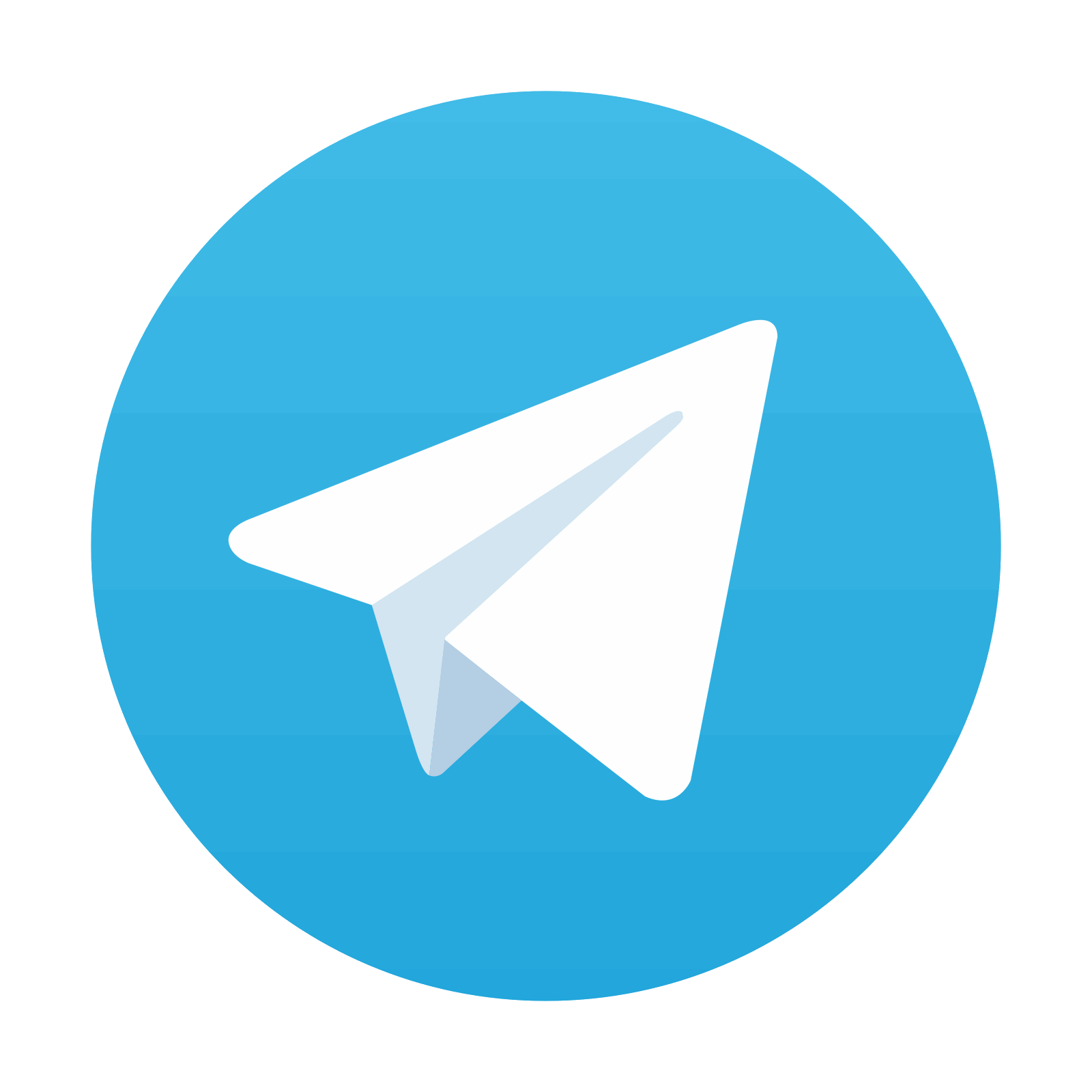
Stay updated, free articles. Join our Telegram channel

Full access? Get Clinical Tree
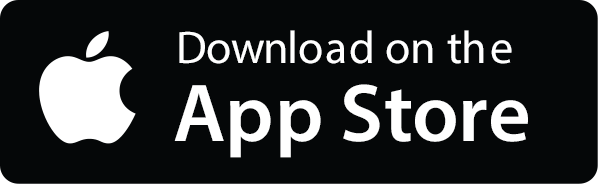
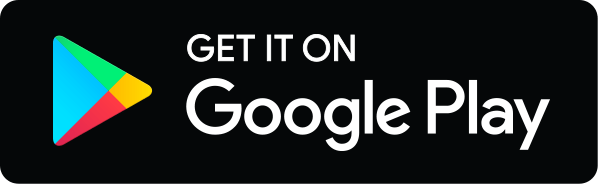
