Fig. 13.1
Percutaneous renal denervation procedure. Graphic of catheter tip in distal renal artery. Reproduced with permission from Ardian Inc [19].
13.1 Functional Anatomy of the Renal Nerves
The efferent renal sympathetic nerves modulate renal function through the release of local neurotransmitters. Although immunofluorescent and immunocytochemical methods have identified a large number of substances in renal nerve terminals, only neuropeptide Y and norepinephrine are thought to act as true neurotransmitters. These two substances are released in response to renal nerve activation and bind to specific postjunctional receptors in the kidney. Direct stimulation of the renal sympathetic nerves results in frequency-dependent renal vasoconstriction. Sympathetic activation causes greater constriction of afferent arterioles to the glomerulus than efferent arterioles, causing a drop in glomerular pressure and glomerular filtration rate (GFR). In addition, sympathetic activation reduces blood flow to both the renal cortex and medulla. The renal vasoconstriction induced by sympathetic efferent stimulation results from norepinephrine acting predominantly on α1-adrenergic receptors and neuropeptide Y acting on Y1 receptors [5].
Since changes in renal sympathetic tone may influence RBF and GFR, which in turn influence sodium and water excretion, it is difficult to determine whether there is any direct effect of renal nerve activity on the tubular handling of salt and water. In animal models of sodium overload, blockade of renal α-adrenergic receptors or renal denervation increases urinary sodium excretion in the absence of changes in renal haemodynamic parameters. These findings suggest that there exists a level of efferent renal sympathetic nerve activity that can directly affect renal tubular sodium and water reabsorption independent of changes in GFR, RBF or the intrarenal distribution of blood flow. When the renal sympathetic nerves are directly stimulated at a frequency that is subthreshold for renal vasoconstriction, there is a reversible decrease in urinary sodium excretion without a change in renal perfusion pressure, GFR, RBF or the intrarenal distribution of blood flow (Table 13.1) [6].
Table 13.1
Changes in renal function at different frequencies of renal nerve stimulation
Frequency of RNS, Hz | Renin release | Na + excretion | GFR | RBF |
---|---|---|---|---|
0.25 | NC | NC | NC | NC |
0.50 | ↑ | NC | NC | NC |
1.00 | ↑ | ↓ | NC | NC |
2.00 | ↑ | ↓ | ↓ | ↓ |
This direct antinatriuretic effect of sympathetic activation appears to be mediated by α1-adrenergic receptors.
In addition to neural influences on blood flow and tubular secretion of sodium, the renal sympathetic nerves also stimulate the release of renin from juxtaglomerular cells, which is thought to be meditated by activation of β1-adrenergic receptors with a subsequent increase in cAMP. This mechanism of renin release is independent from but complementary to the release of renin mediated by the vascular baroreceptor mechanism in the afferent arterioles of the juxtaglomerular apparatus and the tubular macula densa mechanism that responds to the sodium load in the distal tubule. Moreover renal sympathetic tone appears to modulate the expression of renin mRNA in the kidney.
The efferent sympathetic activity mediates changes in renal function, but the kidney has also an extensive network of afferent unmyelinated fibres that transmit important sensory information to the central nervous system (CNS). Afferent fibres from the kidney have been shown to travel along with the sympathetic nerves at the level of the kidney and then enter the dorsal roots and project to neurons at both spinal and supraspinal levels. Most of the brainstem regions involved in cardiovascular control including the hypothalamus receive inputs from the renal afferents. Renal afferents are thought to carry information to the CNS from renal chemoreceptors that respond to changes in the composition of the interstitial fluid environment and mechanoreceptors that monitor hydrostatic pressure changes within the kidneys [7]. Mechanoreceptors are located both in the renal cortex and in the renal pelvis, whereas chemoreceptive nerve endings are found primarily in the submucosal layers of the renal pelvis. Direct electrical stimulation of the renal afferent nerves in animals may produce both sympathoinhibitory and sympathoexcitatory reflexes, which reflect the diverse functional nature of various populations of renal receptors [8].
Whether activation of afferent renal nerves contributes to the regulation of arterial pressure and sodium balance has been long overlooked. In normotensive rats, activating renal mechanosensory nerves decreases efferent renal sympathetic nerve activity (ERSNA) and increases urinary sodium excretion, suggesting an inhibitory renorenal reflex. There is an interaction between efferent and afferent renal nerves, whereby increases in ERSNA increase afferent renal nerve activity (ARNA), leading to decreases in ERSNA by activation of the renorenal reflexes to maintain low ERSNA to minimise sodium retention. Increased renal ANG II reduces the responsiveness of the renal sensory nerves in physiological and pathophysiological conditions, including hypertension, congestive heart failure and ischemia-induced acute renal failure. Impairment of inhibitory renorenal reflexes in these pathological states would contribute to the hypertension and sodium retention. When the inhibitory renorenal reflexes are suppressed, excitatory reflexes may prevail. Renal denervation is associated with a fall in muscle sympathetic nerve activity, suggesting that there may be increased renal afferent activity prior to ablation that causes a reflex increase in global sympathetic tone [9].
Although removal of both renal sympathetic and afferent renal sensory nerves most likely contributes to the overall renal and haemodynamic effects initially, additional mechanisms may be involved in long-term effects since sympathetic and sensory nerves may reinnervate renal tissue in a similar time-dependent fashion following renal denervation [10].
Much less is known about the normal function of the renal afferent nerves than the efferent nerves, but there is evidence that renal afferents play a role in the reflex increase in sympathetic tone that occurs in hypertension. Presumably, the renal afferents also mediate a reflex increase in sympathetic tone in CHF, but this has not yet been confirmed in humans or experimental animals.
The increase in sympathetic activity may start as a compensatory response to ventricular dysfunction and reduced cardiac output, but may become part of a pathological positive feedback cycle with the progression of CHF. Although there is a global increase in sympathetic tone in CHF that is due to CNS integration of all afferent input, sympathetic outflow does not increase equally to all organs. Some vascular beds such as the renal vasculature receive greater sympathetic activation than others in the presence of CHF. A disproportionate increase in renal sympathetic activity results in increased renal vascular resistance compared with other systemic vascular beds, and this results in increased plasma renin activity as well as sodium and water retention. The disproportionate increase in renal sympathetic activity may be an important mechanism that reduces GFR and prevents a compensatory natriuresis during the progression of CHF [11].
13.2 Interventions that Reduce Renal Sympathetic Activation in CHF
Although bilateral renal denervation has little effect on kidney function in normal animals, several studies in animal models of heart failure have established the beneficial effects of renal denervation. In a rat model of heart failure induced by left anterior descending artery ligation, increased sodium retention was abolished by surgical renal denervation. Surgical renal denervation in dogs with an arteriovenous fistula and compensated high-output heart failure improved the postprandial natriuresis in response to a sodium load (125 mEq). The therapeutic value of renal denervation was demonstrated in two other rat models of heart failure induced by coronary ligation. Surgical renal denervation was performed prior to coronary ligation and resulted in reduced ventricular filling pressures and improved ventricular function after ligation compared to nondenervated control animals. Renal denervation was also shown to restore the diuresis and natriuresis in response to exogenously administered atrial natriuretic peptide in rats with heart failure induced by coronary ligation. In a rabbit model of pacing-induced heart failure, there was a decrease in RBF, an increase in renal vascular resistance, an increase in the expression of angiotensin II type 1 receptors in renal cortical vessels and a decrease in the expression of angiotensin II type 2 receptors. All of these changes were prevented by renal denervation prior to the induction of heart failure. Taken together, the results of these animal studies suggest that renal denervation may be particularly useful in the treatment of CHF [12].
Although there is little information on the effects of renal denervation in CHF patients, there is indirect evidence for the beneficial effects of interventions that reduce renal sympathetic activation. The observation of a natriuresis in response to acute intrarenal α-adrenergic blockade in CHF patients supports a therapeutic role for reducing the effects of increased renal sympathetic nerve activity on sodium and water retention. Renin release from the kidneys is influenced by β1-adrenergic receptors, and the administration of β-blockers without intrinsic sympathomimetic activity has been shown to reduce plasma renin activity in CHF patients. Furthermore, the beneficial effects of angiotensin-converting enzyme inhibitors and angiotensin II receptor blockers in CHF patients may be mediated in part by reducing or attenuating the negative effects of renal sympathetic activation [13].
Even the efficacy of loop diuretics may be influenced by renal sympathetic activity. A recent study showed that total urine volume and sodium excretion were considerably greater when CHF patients received the same dose of furosemide after lying down for 90 min, as opposed to being active (sitting or walking on a treadmill). Plasma norepinephrine and renin were higher with the upright posture, and these results suggest that the reduced response to furosemide was due to an increase in renal sympathetic tone. These findings suggest that renal denervation may enhance the efficacy of exogenous diuretics administered to CHF patients [14].
13.3 Afferent Renal Nerve Activation in CHF
The afferent nerves in the kidney may also play a role in CHF. The precise population of afferent nerves that are activated and the mechanisms responsible for their activation are unknown. However, it is likely that increased renal afferent nerve signals travel to the CNS and induce a reflex increase in renal sympathetic tone (renorenal reflex), thereby causing renal vasoconstriction, renin secretion and sodium and water retention. Moreover, increased renal afferent nerve signals that travel to the CNS may also cause a reflex increase in sympathetic tone to other organs that have a dense sympathetic innervation, such as the heart and the peripheral vasculature. The CNS in this case serves as the central component of the reflex arc. It is possible that a pathological positive feedback cycle at the level of the kidney may exist, whereby an increase in renal efferent sympathetic tone leads to an increase in renal afferent nerve activity, which further increases efferent sympathetic outflow from the CNS [15].
Although several substances (nitric oxide, calcitonin gene-related peptide, substance P, H+, adenosine) have been postulated to stimulate chemosensitive renal afferents under various conditions, adenosine is of particular interest, since it is readily released by renal proximal tubular cells directly into the tubular fluid during increased metabolic activity. Furthermore, intrarenal adenosine has been shown to be elevated in patients with CHF, and it may activate renal afferents resulting in a reflex increase in sympathetic tone.
Although studies of renal afferent activity in animals or patients with CHF are limited, there is evidence of renal afferent activation in other types of kidney dysfunction. In humans with end-stage renal disease, nephrectomy in patients with or without kidney transplantation resulted in a reduction of muscle sympathetic nerve activity and total body norepinephrine spillover, confirming the role of renal afferents in mediating a reflex increase in sympathetic tone [16].
13.3.1 Renal Denervation Technique
Renal sympathetic denervation in the management of hypertension has previously been explored in man via surgical nephrectomy and even radical surgical sympathectomy. Surgical renal denervation has been shown to be an effective means of reducing sympathetic outflow to the kidneys, augmenting natriuresis and diuresis, and reducing renin release, without adversely affecting other functions of the kidney such as GFR and RBF. However, these early surgical approaches (e.g. splanchnicectomy) were complicated by severe orthostatic hypotension, impotence and incontinence [17, 18].
A minimally invasive, catheter-based approach to directly target sympathetic nerves adjacent to the renal artery has therefore been developed in an attempt to overcome the above surgery-related problems (Fig.13.2).
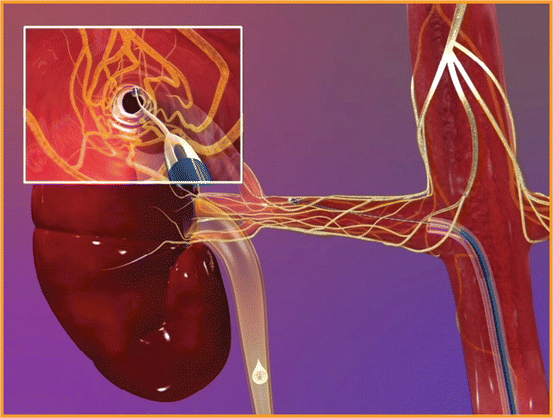
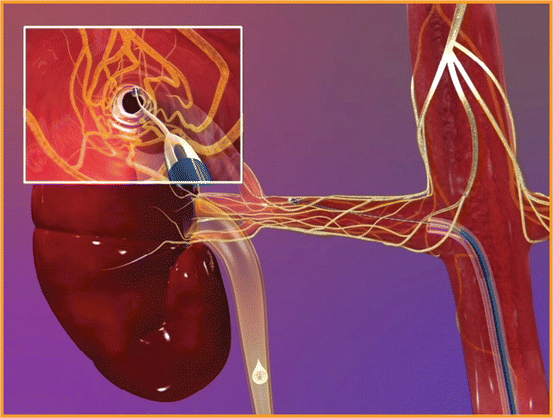
Fig. 13.2
Reflex axe of the effects of sympathetic tone activation in chronic heart failure on the kidneys, heart and peripheral vasculature (Modified with permission from Sobotka et al. [20])
The renal denervation procedure itself involves femoral or radial artery catheterization, with the tip of the catheter being placed in the distal renal artery. The principle is based on the delivery of high-frequency energy applied to the endothelial lining. This procedure is repeated 4–5 times in the individual renal artery and then the same energy is applied to the contralateral renal artery, which interrupts sympathetic nerves located in the adventitia of the renal arteries, whilst the vessel is cooled by the high intraluminal blood flow [21, 22].
Renal imaging should be undertaken to exclude atherosclerotic renal artery disease prior to catheterization and a contrast renal angiogram performed at the time of catheterization (but pre-procedure) to ensure again that no major renovascular disease was present as well as to exclude dual renal arteries and other anatomical abnormalities.
Peri-procedural problems at the time of radiofrequency (RF) energy application include loin pain. This is usually treated with prophylactic use of intravenous analgesia. Potential longer-term complications such as vessel thrombosis have been mitigated with the prophylactic use of aspirin and clopidogrel. Evaluation of development of catheter-related complications to the treated vessel was performed using various angiographic techniques, including computed tomography and magnetic resonance angiography. Very few peri-procedural complications have been reported in the wide experience in resistant hypertensive patients so far such as vessel thrombosis, kidney embolisation or renal artery dissection upon placement of the catheter for RF energy delivery. Sporadic clinical evidences of renal artery stenosis in the follow-up period have been reported.
A key issue with this RF energy denervation approach is whether anatomical and/or functional regrowth of renal sympathetic nerves occurs. Studies in rats subjected to renal surgical denervation have shown that functional reinnervation of the renal vasculature begins to occur between 14 and 24 days after denervation, with complete return of neural function by 8 weeks. It is likely that some efferent sympathetic reinnervation occurs in patients after catheter-based renal denervation, although the time course of this response is unknown. Furthermore, it is difficult to clinically evaluate the amount of reinnervation, since there are no simple diagnostic tests that can evaluate the functional innervation of the kidneys. In contrast to efferent nerves, the afferent nerves may have less capacity to regenerate. Thus, the removal of renal afferent activity with renal denervation and the subsequent effects on central sympathetic outflow may be sustained [19].
It is unknown whether it is disruption of the afferent or the efferent traffic that is the more important element of the treatment, but the effect is a substantial reduction in blood pressure. The first procedure has been performed in patients with resistant hypertension. In patients with uncontrolled hypertension, despite 4.7 ± 1.5 antihypertensive drugs, with average baseline blood pressure levels of 177/110 mmHg, there was a reduction of blood pressure by up to 27/17 mmHg at 12 months. These reductions were sustained over 2 years [23, 24].
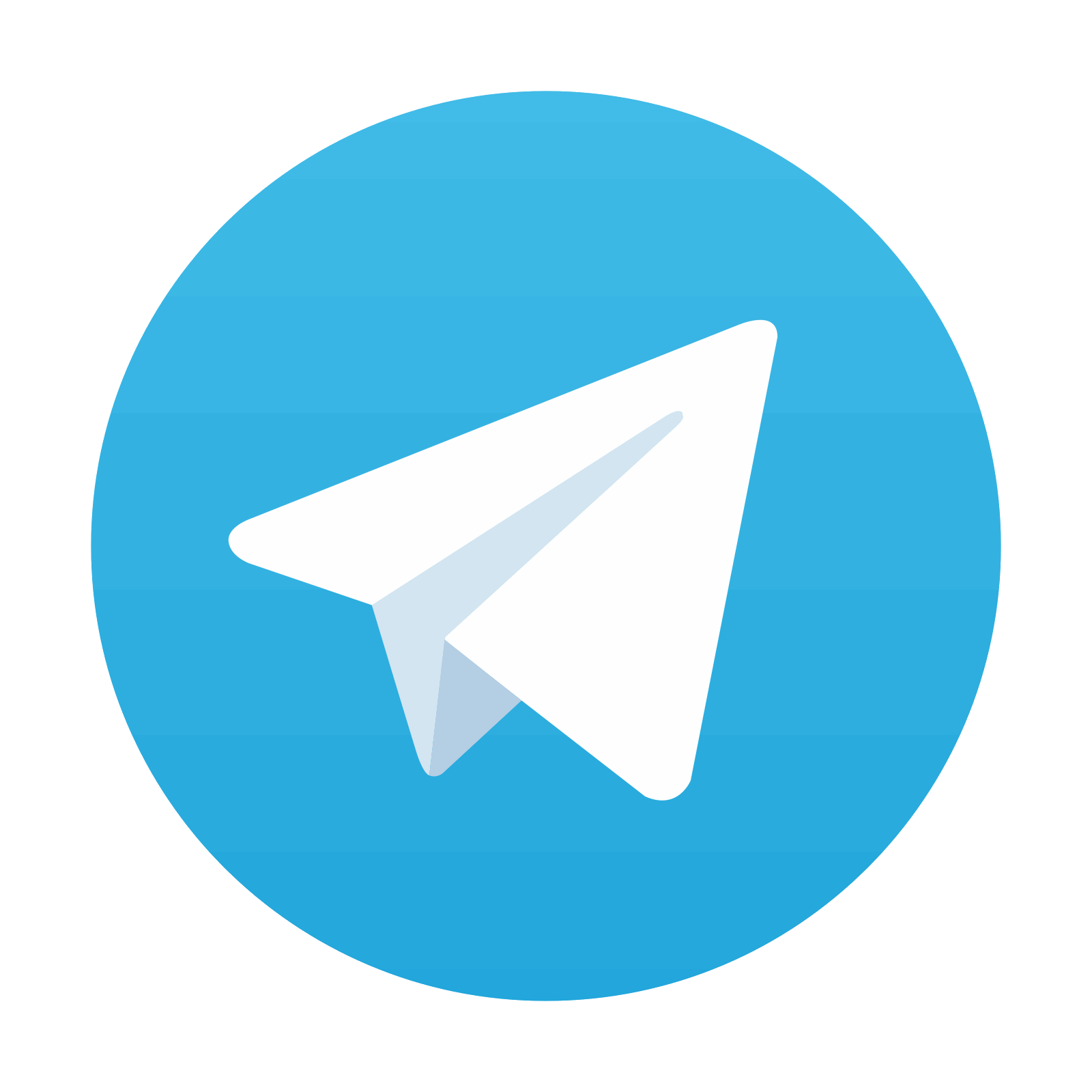
Stay updated, free articles. Join our Telegram channel

Full access? Get Clinical Tree
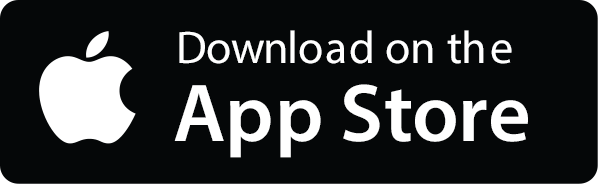
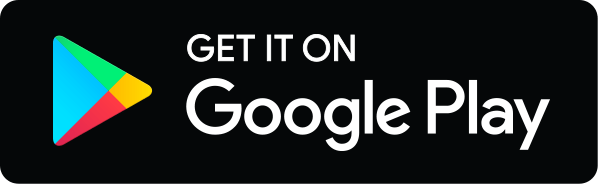