Clinical benefit from any revascularization procedure will be the net gain due to reperfusion of the ischemic organ balanced against the risk of a procedural complication. The same is true for renal artery ischemia caused by atherosclerotic or, less commonly, fibromuscular dysplasia (FMD) lesions (Fig. 52-1). Recent clinical trials have attempted to determine the benefit of revascularization but have been seriously hampered by difficulty in defining a threshold for an ischemia producing renal artery stenosis (RAS).
FIGURE 52-1
A. Selective left renal artery angiography from the right radial access using a 5-Fr, 125-cm-long multipurpose catheter. Note there is a moderately severe proximal stenosis present (arrow). B. Selective right renal artery angiography from the right femoral artery with a 5-Fr internal mammary catheter. Note the appearance of “stacked coins” (arrow) diagnostic of fibromuscular dysplasia (FMD).


Renal ischemia due to an obstruction of the renal artery causes 3 well-described clinical scenarios: (1) renovascular hypertension, (2) ischemic nephropathy, and (3) cardiac destabilization syndromes such as sudden-onset pulmonary edema, decompensated heart failure, and acute coronary syndromes. Helping to identify patients with these clinical manifestations of renal ischemia who also have anatomically suitable lesions for treatment is the purpose of this chapter. The primary method of revascularization for renal artery stenosis is endovascular, not open surgery. Open surgery is rarely performed today and usually accompanies another related open surgical procedure on the abdominal aorta, for example.
The prevalence of renal artery stenosis (RAS) depends on the population examined. In a Medicare population (mean age, 77 years), screening renal ultrasound duplex studies demonstrated greater than 60% RAS in 6.8% of patients.1 There were almost twice as many males (9.1%) as females (5.5%; P = .053), and there were no racial differences (Caucasian, 6.9%; African American, 6.7%) in the prevalence of RAS. Among the general hypertensive population, RAS is the most common (2%-5%) secondary cause of hypertension. An autopsy series of patients older than 50 years found RAS (≥50%) in 27% of patients, and this rate increased to 53% if there was a history of diastolic hypertension >100 mm Hg. Among patients entering dialysis treatment, 10% to 15% have RAS as the cause of end-stage renal disease.2 Approximately 25% of elderly patients with renal insufficiency have unsuspected RAS.
RAS is predominantly due to atherosclerosis in the adult population, with FMD being more common in younger females.3 RAS is more common in patients who have atherosclerosis involving any other vascular bed. In patients undergoing cardiac catheterization for suspected coronary artery disease, the prevalence of RAS ranges from 25% to 30%, whereas peripheral arterial disease or abdominal aortic aneurysm is associated with RAS in 30% to 40% of cases.
Atherosclerotic RAS generally progresses over time and is often associated with loss of renal mass and worsening renal function. Lesion progression in serial angiographic studies ranges from 39% to 49%. RAS lesion progression is directly related to the underlying severity of the stenosis. The more severe the narrowing (≥60%), the more likely it is to progress to occlusion.4 Worsening of “asymptomatic” RAS is associated with a progressive loss of renal function.5 Control of blood pressure with medical therapy does not prevent progression of RAS.6,7 Renal artery occlusion occurred in 16% of the medically treated arm over a 1-year period in a randomized trial.8
Atherosclerotic RAS remains a major cause of end-stage renal disease. In a serial angiographic study of RAS patients compared to normal controls, renal function significantly declined and was related to the severity of the RAS. RAS is estimated to be responsible for renal failure in 10% to 15% of patients entering dialysis programs. In patients starting dialysis secondary to atherosclerotic RAS, the mean survival was 25 months, and the 2-, 5-, and 10-year survival rates were 56%, 18%, and 5%, respectively.
RAS is an independent predictor of adverse cardiovascular events such as myocardial infarction, stroke, and cardiovascular death. Patients with significant RAS are more likely to have renal insufficiency, coronary artery disease, peripheral arterial disease, hypertension, and cerebrovascular disease. In patients identified as having greater than 75% RAS at the time of cardiac catheterization, the 4-year survival was 57% compared to 89% in patients without RAS (P < .001). Patients with greater severity of RAS have worse survival than those with milder or no RAS. Patients with bilateral RAS exhibit a lower 4-year survival (47%) when compared to patients with unilateral RAS (59%; P < .001), independent of prior myocardial revascularization procedures.9
Screening for RAS is appropriate in patients at increased risk for RAS (Table 52-1). Whenever possible, screening tests for RAS should be performed noninvasively using direct imaging tests (Doppler ultrasound, computed tomographic angiography [CTA], or magnetic resonance angiography [MRA]) (Fig. 52-2). Noninvasive imaging has become so sophisticated and accurate that it is seldom necessary to perform catheter-based angiography for the diagnosis of renal artery disease.
|
It may be appropriate to perform screening angiography for RAS at the time of cardiac or peripheral vascular angiography of other vascular beds.10,11 For patients who are candidates for revascularization with risk factors as outlined in Table 52-1 or clinical syndromes suggestive of RAS, aortography is given a Class I indication for screening at the time of angiography performed for other clinical indications. There is good evidence that nonselective, diagnostic, screening renal angiography is safe and is not associated with incremental risk when performed at the time of cardiac catheterization.12
Duplex ultrasonography (DUS) is an excellent noninvasive imaging test to detect RAS but is highly dependent on the skills of the technician performing the test. It is the least expensive of the imaging modalities and provides useful information about the degree of stenosis, the kidney size, and other associated disease processes such as obstruction. The location and degree of stenosis can accurately be determined by duplex ultrasound of the renal artery.
Overall, when compared to angiography, DUS has a sensitivity and specificity of 84% to 98% and 62% to 99%, respectively, when used to diagnose RAS. Renal artery duplex is an excellent test for the follow-up after renal stenting to confirm patency. Following endovascular therapy, a renal artery duplex should be obtained within the first few weeks to establish a baseline, and then at 6 months, 12 months, and yearly thereafter to ensure patency.
One drawback of DUS is that it may not identify RAS in accessory renal arteries (67%) compared to main renal arteries (98%). Therefore, if the patient has hypertension that cannot be adequately controlled with a good regimen, and the DUS fails to demonstrate RAS, another imaging modality may be considered to identify stenosis of an accessory renal artery.
The intrarenal resistance index (RI) is the ratio of the peak systolic to end-diastolic velocity within the renal parenchyma at the level of the cortical blood vessels.13 The RI is a representation of small vessel glomerulosclerosis. There have been conflicting reports regarding the usefulness of RI to predict individual patient response to revascularization. One retrospective study demonstrated that an elevated RI greater than 0.80 predicted a lack of improvement in blood pressure and renal function after revascularization; however, it included balloon angioplasty without stent procedures, a strategy that is now recognized as less optimal compared to stenting (see below).14 A prospective study of renal stenting in 241 patients with an elevated RI (>0.70) showed improvement in blood pressure and renal function after intervention.15 Patients with a higher RI (>0.8) actually benefitted more from revascularization than did those with milder elevations.
CTA uses ionizing radiation and iodinated contrast to produce excellent images of the abdominal vasculature (Fig. 52-3). CTA has a sensitivity and specificity for detecting RAS of 89% to 100% and 82% to 100%, respectively. Excellent 3-dimensional image quality with enhanced resolution can be obtained with multidetector-row CTA technology. The advantages of CTA over MRA include greater spatial resolution, an absence of flow-related phenomena that may overestimate the degree of stenosis, and the capability to visualize calcification and metallic implants such as endovascular stents and stent grafts. CTA is well tolerated with an open gantry, and thus, claustrophobia is not as limiting a factor as it is for MRA. The disadvantages of CTA compared to MRA are exposure to ionizing radiation and the need to administer potentially nephrotoxic iodinated contrast agents.
MRA also provides excellent imaging of the abdominal vasculature and associated anatomic structures (see Fig. 52-2). When compared with angiography, MRA has demonstrated a sensitivity of 91% to 100% and a specificity of 71% to 100%. Contrast-enhanced MRA using gadolinium improves image quality when compared with noncontrast studies and shortens imaging time, thereby eliminating some of the artifact created by gross patient movement. However, MRA does not have the same sensitivity and specificity in patients with FMD and is generally not a good screening test if FMD is suspected.
MRA should not be used in patients with a glomerular filtration rate less than 30 mL/min/1.73 m2 because of the increased likelihood of developing nephrogenic systemic fibrosis. MRA may not be used in patients with metallic (ferromagnetic) implants such as some mechanical heart valves, cerebral aneurysm clips, and electrically activated implants (pacemakers, spinal cord stimulators). At present, MRA is not very helpful in following patients after stent implantation due to imaging artifact produced by the metallic stent.
The traditional “gold” standard for determining the severity of RAS has been invasive angiography. The Achilles heel of renal stenting is the inaccuracy of the angiographic determination of the severity of the ostial renal stenoses. Even with quantitative measurement, angiography may be unable to discriminate between nonobstructive stenoses and clinically significant ones (Fig. 52-4).16 Most would agree that interventionalists are able to identify “critical” stenoses in renal arteries, but for mild to moderately severe lesions, physiologic confirmation is necessary.
FIGURE 52-4
Lack of correlation between visual estimated renal artery stenosis and an objective measure of the hemodynamic gradient (renal fractional flow reserve [RFFR]) across the stenosis. (Reproduced with permission from Subramanian R, White CJ, Rosenfield K, et al. Renal fractional flow reserve: a hemodynamic evaluation of moderate renal artery stenoses. Catheter Cardiovasc Interv. 2005;64:480-486, Figure 4.)

Hemodynamic measurement of the severity of RAS assures that there is a physiologic reason to expect benefit with revascularization. Confirmation of a hemodynamic gradient is evidence of physiologic RAS with renin release and has been documented by De Bruyne and colleagues.17 Hemodynamic parameters of significant RAS (peak systolic gradient >21 mm Hg,18 renal fractional flow reserve [FFR] of ≤0.8,19 and a dopamine-induced mean translesional gradient ≥20 mm Hg20) are associated with clinical improvement after renal stenting in patients with mild to moderate renal artery stenoses.
Renal FFR is measured after induction of maximum hyperemia. This hemodynamic assessment of flow, which is widely used in the coronary circulation, is based on the principle that flow across a conduit artery is proportional to pressure across the vascular bed and inversely proportional to the resistance of the vascular bed. Under conditions of maximum hyperemia, the flow through the conduit artery is maximal, while the resistance of the vascular bed is at a minimum and constant. Any reduction in flow under these conditions is caused by the stenosis and is proportional to the ratio of pressure distal to the stenosis (Pd) and the pressure proximal to the stenosis (Pa). Renal hyperemia can be achieved with papaverine,16,21 dopamine,22 or acetylcholine.23 Translesional pressure gradients are measured, and FFR (Pd/Pa) is calculated using a 0.014-inch pressure guide wire. Renal artery FFR correlates well with other hemodynamic parameters of lesion severity21,24 and, in some series, has been proven to be a better predictor of clinical response. In one study, renal FFR was measured after renal stent placement in 17 patients with refractory hypertension and moderate to severe (50%-90% stenosis) unilateral RAS. Ten patients had normal baseline renal FFR (defined as FFR ≥0.80), whereas an abnormal baseline renal FFR (<0.80) was recorded in 7 patients. At 3 months after intervention, 86% of patients with an abnormal renal FFR experienced improvement in their blood pressure, compared with only 30% of those with normal renal FFR (P = .04). In this small series, baseline systolic, mean, or hyperemic translesional pressure gradients were not different between patients whose blood pressure improved and those in whom it did not.25
Angiographic measurements of renal blood flow by using renal frame counts (RFC) and renal blush grades (RBG) for microvascular flow can differentiate normal patients from patients with FMD.26 RFC is the number of cine frames required for the contrast to reach the smallest visible distal branch in the renal parenchyma. As in Thrombolysis in Myocardial Infarction (TIMI) frame counting, the first frame used for the RFC is the frame in which the contrast first fills the main renal artery. The last frame is when contrast enters the smallest visible branch of the distal renal parenchyma along the axis of the main renal artery. The measurements are done with 30-frames-per-second angiography.
RFC was initially described in patients with FMD of the renal arteries (15 kidneys), who were compared to subjects with normal renal arteries (50 kidneys) and had a significantly higher (prolonged) mean RFC (26.9 vs 20.4; P = .0001).26 Hypertensive patients with renal artery stenoses have also been shown to have decreased renal perfusion as measured by RFC.27 Clinical responders tended to have higher baseline RFCs than nonresponders and had greater improvement in their RFC values following renal stenting. Three-quarters of the hypertensive patients who responded to renal stenting had a baseline RFC ≥25, and if the RFC improved by >4, then 79% were responders to renal stenting.
It has been shown that patients with the highest systolic blood pressures have the greatest decrease in systolic pressure,28 but there is no correlation between blood pressure improvement after renal stent placement and the variables of age, sex, race, severity of stenosis, number of vessels treated, baseline diastolic pressure, or baseline serum creatinine. Two variables, bilateral RAS (odds ratio [OR], 4.6; P = .009) and mean arterial pressure >110 mm Hg (OR, 2.9; P = .003), are associated with improved blood pressure response following renal artery stent placement. Studies comparing the results in elderly (≥75 years) versus younger (<75 years) patients or in females versus males have failed to show any difference in response to renal stent placement.
An analysis of 527 renal stent patients enrolled in 5 modern prospective, multicenter (117 centers) studies demonstrated that systolic blood pressure (SBP) and diastolic blood pressure (DBP) were significantly decreased at 9 months.28 An SBP reduction >10 mm Hg occurred in 61% of patients. A baseline SBP >150 mm Hg was strongly associated with blood pressure response, but other clinical characteristics were not. In hypertensive patients in whom RAS is identified, the best predictor of RAS benefit was a baseline SBP >150 mm Hg.
The current American Heart Association (AHA)/American College of Cardiology (ACC) guideline indications for RAS in hypertensive patients with hemodynamically significant RAS and a viable kidney (linear length >7 cm) include: (1) accelerated hypertension, (2) refractory hypertension (failure of 3 appropriate drugs, 1 of which should be a diuretic29), (3) hypertension with a small kidney, and (4) hypertension with intolerance to medications (Class IIa, Level of Evidence B).30 By convention, a hemodynamically significant lesion requires demonstration of a ≥70% RAS by visual estimation, ≥70% RAS by intravascular ultrasound measurement, or a 50% to 70% RAS with a systolic gradient of ≥20 mm Hg or a mean translesional gradient of ≥10 mm Hg.30
The recently published Cardiovascular Outcomes in Renal Atherosclerotic Lesions (CORAL) trial compared the initial treatment strategy for renovascular hypertension patients; patients received either multifactorial medical therapy (eg, an angiotensin receptor blocking agent, a thiazide-type diuretic, amlodipine, atorvastatin, antiplatelet therapy, and diabetes managed according to clinical practice guidelines) or medical therapy plus renal stenting consistent with the guidelines.31 The CORAL study found that the primary composite end point (death from cardiovascular or renal causes, myocardial infarction, stroke, hospitalization for congestive failure, progressive renal insufficiency, or the need for renal replacement therapy) in patients with RAS (>60% diameter stenosis) and poorly controlled hypertension on at least 2 medications did not differ between groups. The number of blood pressure medications was not different between the groups (medical vs stent: 3.5 ± 1.4 vs 3.3 ± 1.5 medications) at the completion of the trial, and both groups had a similar decrease in SBP (15.6 ± 25.8 mm Hg in the medical therapy group vs 16.6 ± 21.2 mm Hg in the stent group). The CORAL recommendations for an initial trial of multifactorial medical therapy are consistent with the current AHA/ACC guidelines, which require that patients fail medical therapy for renovascular hypertension prior to revascularization.
Treatment of ischemic nephropathy continues to be a source of debate among experts. Opponents of revascularization of RAS patients with renal insufficiency contend that the kidney is supplied with an excess of nutrient blood flow, and therefore, few kidneys will benefit from revascularization.32 This does not explain the 10% of patients beginning dialysis for end-stage renal disease due to renal artery occlusion.
The literature is replete with patient series in which RAS improves renal function,33-37 as well as counterbalancing reports of worsening of renal failure after successful RAS.38-40 However, there are no large randomized studies demonstrating benefit of revascularization over medical therapy alone for improving renal function.
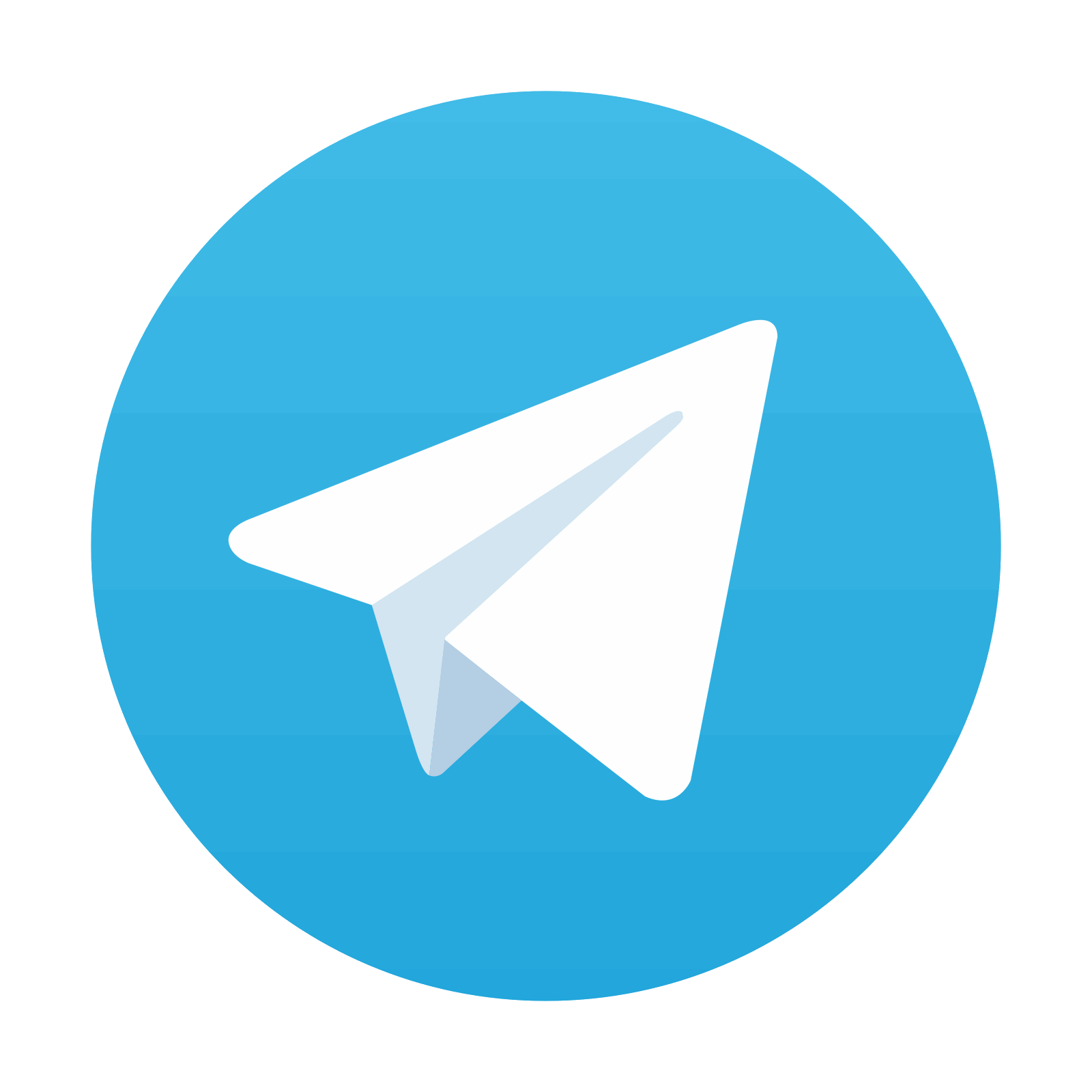
Stay updated, free articles. Join our Telegram channel

Full access? Get Clinical Tree
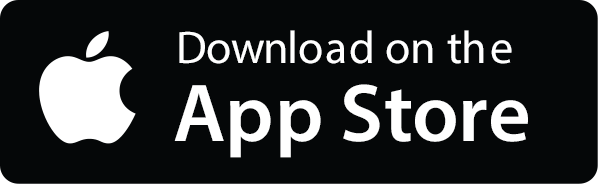
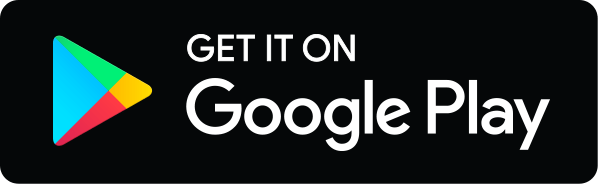