Abstract
Mitral regurgitation (MR) is a common valvular pathology with significant morbidity and mortality implications. Mechanical treatment of this condition is more effective than medical treatment and surgical correction has traditionally been the mechanical method of choice. Following major advances and wide acceptance of percutaneous interventions for coronary artery diseases, the field of valvular heart disease became an attractive target for transcatheter treatment modalities. Significant steps have been achieved in the field of percutaneous treatment of mitral stenosis as well as aortic stenosis, and lately, mitral regurgitation has been the focus of interest for many investigators looking for transcatheter solutions. Percutaneous edge-to-edge techniques and annuloplasty are innovative but have many disadvantages including the inability to reintervene and leaving a foreign body behind, respectively. Since the mitral and tricuspid annuli have dense collagen, a treatment modality targeting that collagen is logical. Observing the thermal effect on collagen, which causes conformational changes and shrinkage, radiofrequency energy was tested to evaluate its effect on the collagen-rich structure that is the mitral valve annulus. The potential of shrinking the mitral annulus by applying direct thermal source could be a promising modality for the treatment of mitral regurgitation with potential open and percutaneous applications. This paper presents an overview of the recent advances in transcatheter treatment of mitral regurgitation focusing on a new treatment modality that aims at reducing the mitral valve annulus diameter through the direct application of thermal energy using a radiofrequency energy probe.
1
Introduction
Mitral regurgitation (MR) is a common valvular abnormality representing the second most frequent single native valve disease in industrialized countries after aortic stenosis . It results from abnormal coaptation of the mitral valve leaflets during systole creating a regurgitant orifice that allows the blood to flow, abnormally, from the left ventricle (LV) to the left atrium (LA). This leads to volume overload on the LV which, in turn, leads to LV remodeling and dysfunction . Historically, rheumatic heart disease accounted for most cases of mitral regurgitation. Currently, the most common etiologies for MR in the developed countries are ischemic and degenerative changes in the mitral valves . The natural history of MR has not been well defined due, in part, to the imprecise assessment of the degree of MR. While patients with mild MR appear to have a good prognosis , those with severe MR have higher morbidity and mortality .
1.1
Anatomy of the Mitral Valve
A detailed description of the anatomy of the mitral valve is beyond the scope of this article and interested readers are referred to an excellent detailed description by Muresian . It is important to recognize that the mitral valve is an important component of the LV, hence the use of the term mitral valvular complex (MVC), which is a finely tuned system of elements acting in a coordinated manner. The MVC includes the mitral valve, the left atrial myocardium, the left ventricular myocardium, the left atrial and left ventricular endocardium, and the aortomitral curtain (the mitral-aortic continuity). The mitral valve per se is composed of the valvular leaflets (including the commissures), the annulus, and the subvalvular apparatus (tendinous cords and the papillary muscles) ( Fig. 1 ).

The leaflets are traditionally subdivided into an anterosuperior and posteroinferior segments separated by two commissural areas. The anterior mitral leaflet is wider and occupies approximately one third of the annular circumference. The attachment of the anterior leaflet to the mitral annulus extends to the aortic annulus through fibrous tissue, providing “fibrous continuity” between the aortic and mitral valve.
On microscopic examination, the leaflets have a fibrous skeleton consistent of dense collageneous core called the fibrosa, covered towards the atrial side by a layer of myxomatous connective tissue called the spongiosa. The annulus does not represent a well-defined anatomical structure and has different anatomical, surgical, and echocardiographic definitions. Anatomically, it is the fibroelastic or collagenous structure that encircles the orifice between the LA and LV at the level of the atrioventricular junction. Surgically, the annulus is identified at the level of the visible transition between the left atrial myocardium and the mitral leaflet. Echocardigraphically, the annulus is identified at the valvular hinge region, with a resultant saddle-shaped form with its highest points at the middle of the anterior leaflet adjacent to the aortic valve and at the middle of the posterior leaflet. Trichrome staining shows that the mitral valve annulus is rich with dense collagen ( Fig. 2 ).

1.2
Treatment of Mitral Regurgitation
Mitral regurgitation is a mechanical problem resulting from malcoaptation of the leaflets and can best be corrected with a mechanical solution . Improving the coaptation of the mitral leaflets is the foundation on which mechanical treatment of MR is predicated, aiming at restoration of valve competence and removing the volume overload and its deleterious consequences.
Mitral valve repair is preferred over mitral valve replacement in nonrheumatic MR because of better outcome , lower operative mortality, and better long-term survival . Destruction of the mitral valve apparatus caused by mitral valve replacement may lead to immediate decrease in LV contractility and increase in afterload . Preserving some or all of the chordal attachments between the papillary muscles and valve leaflets during mitral valve replacement preserves LV function . Horskotte et al. indicated that mitral valve replacement with chordal preservation has favorable impact on mortality and late outcome compared with mitral valve replacement without chordal preservation . Rozich et al. supported the argument that the decrease in ejection fraction after correction of MR is caused by destruction in the mitral valve apparatus rather than the surgery’s effect on the low impedance ejection into the left atrium . In fact, the advantages of mitral valve repair over replacement are no longer debated, and mitral valve repair is the standard of care whenever it is feasible . Repair has been demonstrated to be superior in the setting of combined coronary bypass procedures, reoperations, double-valve procedures, and in elderly patients . Moreover, majority of cases can be done with minimally invasive surgical techniques which has been shown to be safe, with low perioperative morbidity, low rates of recurrent mitral regurgitation, and low rates of re-operation and death at late follow-up . Less invasive approaches for mitral valve repair including transcatheter techniques are being evaluated from bench to clinical trials and have become attractive for cardiologists and cardiac surgeons .
1.3
Trans-catheter treatment of mitral regurgitation
The late 70s of the last century witnessed a revolution in the field of cardiology starting with the introduction of transcatheter interventions. The stage was set by pioneers like Dotter and Judkins ; then, extending on their work, Gruentzig introduced the percutaneous transluminal coronary angioplasty which continued to evolve with novel technology until it gained a remarkable worldwide acceptance. Technical advances in transcatheter procedures, and operator experience led the way to expansion of transcatheter applications to other fields such as pulmonary balloon valvotomy , aortic valvotomy , and mitral valvotomy .
Although still lagging behind percutaneous treatment for aortic or mitral stenoses, percutaneous valve interventions for MR witnessed significant advances over the last few years. Percutaneous treatment for valvular diseases is attractive because it provides a less traumatic alternative avoiding sternotomy and cardiopulmonary bypass, which means lesser complications and shorter hospital stay. These therapeutic modalities are particularly attractive for patient with high surgical risk.
Transcatheter techniques for treatment of MR are, for the most part, percutaneous variations of existing surgical techniques, and some are based on novel concepts in this field. Optimal repair of the mitral valve should remodel the annulus, maintain leaflet mobility, and achieve normal coaptation of the mitral leaflets . It should also avoid injury to adjacent structures such as the aortic valve, the left circumflex artery, the coronary sinus, and the conduction system.
New percutaneous approaches to mitral valve disease have gained significant interest lately, and several review articles addressing these concepts emerged in the last few years . Some of these techniques are still in the pre-clinical investigation phase and some are in the early phase of clinical application. Other than percutaneous mitral valve replacement, which we are not addressing in this paper, most of these novel modalities are percutaneous variations to mitral valve repair. These techniques could be grouped based on the concept of treatment into four groups: Coronary sinus annuloplasty, direct annuloplasty, leaflet repair, and annulus plus left ventricular chamber remodeling.
Coronary sinus annuloplasty is based on the most frequent surgical technique which involves placing a ring to constrict the mitral annulus diameter. The percutaneous approach of this concept relies on the anatomic relationship between the coronary sinus and the annulus and on the fact that the coronary sinus could be easily accessed percutaneously. In this approach, a device is placed in the coronary sinus, which runs parallel to the annulus, to deform the mitral annulus and diminish the annular circumference. Several devices have been used in this approach including the Monarc device (Edwards Lifesciences, Orange, CA, USA) which has been used in clinical settings . This device consists of a self-expanding two stent anchors separated by an extended spring bridging the two elements. The spaces of the spring are kept open by biodegradable material filling these spaces. As the filling material degrades, usually over several weeks, the spring shortens and applies tension on the coronary sinus and the adjacent annulus. Another coronary sinus device used in clinical applications is the Carillon mitral contour system (Cardiac Dimensions, Kirkland, WA, USA), which consists of a nitinol wire-shaping ribbon between proximal and distal anchors . Tension is applied to the wire element between the two anchors to constrain the coronary sinus and reduce the annular circumference. The percutaneous transvenous mitral annuloplasty system (Viacor, Wilmington, MA, USA) is a coronary sinus device that was tested in animals and in open surgery settings. It consists of a multilumen polytetrafluoroethylene catheter in which variable stiffness rods are inserted. The rods compress the midportion of the coronary sinus and diminish the septal to lateral dimension of the mitral annulus . After the optimal number and stiffness of rods have been tested in a temporary diagnostic catheter, a permanent version of the device is implanted. Another approach using the coronary sinus is close to being tested in clinical setting; this is the percutaneous septal-sinus shortening procedure (PS3). In this approach, an anchor is placed in the coronary sinus and a cord traversing the left atrium is attached to an anchor in the fossa ovalis. Tension is applied to the cord to diminish the mitral annulus septal-lateral dimension .
Direct approaches to the mitral annulus are still in infancy. They include the Mitralign device (Mitralign, Tewksbury, MA, USA), which is based on the surgical placation annuloplasty and has been reproduced percutaneously in preclinical models. It uses anchor pledgets placed directly into the mitral annulus from the left ventricular side, and a “drawstring” to cinch the annulus. A catheter is used to access the space on the ventricular side of the posterior mitral leaflet. Clinical studies with the Mitralign device are being planned .
Leaflet repair methods are based on the surgical approach first reported by Alfieri in the early 1990s and involves suturing together the anterior and posterior leaflets at a single point midway between the circumferences of the leaflets resulting in a double orifice mitral valve . The percutaneous version of this approach uses a clip, MitraClip (Evalve, San Francisco, CA, USA), which is delivered to the left atrium via a 24F guide catheter and positioned in the mid left atrial cavity above the mitral orifice. It is then passed into the left ventricle below the mitral leaflets, pulled back to grasp the leaflets, then the clip arms are closed to create a double orifice. This device approach has been successfully used in a Phase I clinical trial in the United States with encouraging results leading to the EVEREST II, an ongoing Phase II Food and Drug Administration approved trial .
The percutaneous annulus and chamber remodeling technology (Coapsys) has been developed in preclinical models and is soon to be applied clinically . It stems from the Coapsys surgical system (Myocor, Maple Grove, MN, USA), which applies the concept of moving the ventricle rather than the annulus to increase leaflet coaptation, by placing pads on either side of the left ventricle with a cord passing through the left ventricular cavity to apply tension to the mitral annulus. This is also referred to as the Septal Lateral Annular Cinching (SLAC).
1.4
The rationale for thermal annular remodeling for treatment for MR
Each of the abovementioned percutaneous techniques has its shortcomings. The utility of the edge to edge technique as a stand alone procedure is debated in the surgical literature. There is concern of suboptimal midterm results for this technique especially in patients with ischemic MR when performed without annuloplasty . Furthermore, the percutaneous approach to annuloplasty involves placement of implantable device, thereby increasing the risk of potential complications, related to the device itself, as well as the anatomic variability of coronary vessels in the case of coronary sinus approach.
One potential area of study is the application of thermal energy, derived from radiofrequency energy (RFE), to improve valve mechanics by modifying the properties of collagen, which is an essential component of the mitral valve annulus. In the next paragraphs, we review the structural properties of collagen, the effect of thermal energy on its properties and the rationale for use of RFE for the treatment of MR.
1.5
Structure and function of collagen
Collagen is the major insoluble fibrous protein in the extracellular matrix and in connective tissues. In fact, it is the single most abundant protein in the animal kingdom, providing the extracellular framework for all multicellular organisms .
Over 26 different types of collagen are identified. The various collagens, and the structures they form, all serve one goal: help tissues withstand stretching. Collagen provides the tensile strength of tendons and ligaments allowing them to stretch without being broken. Also, it provides the structural support of different tissues in the body, and the elasticity of the skin. Types I, II, III, V, and XI are the interstitial or fibrillar collagens and the most abundant in the body. Other collagens form meshworks and may function as anchors in epidermal–dermal junctions, cartilage, and blood vessel wall .
Type I collagen is easy to isolate and was the first to be characterized, but all collagens share the basic structural unit which is the triple helix. The fundamental structural unit of type I collagen is a 300-nm long and 1.5-nm thin protein that consists of three coiled subunits: two α1 (I) chains and one α2 (I). Each chain contains 1050 amino acids wound around one another in a characteristic right-handed triple helix ( Fig. 3 ). All collagens contain three-stranded helical segments of similar structure; the unique properties of each type of collagen of are due mainly to segments that interrupt the triple helix . The triple-helical structure of collagen arises from an abundance of three amino acids: glycine, proline, and hydroxyproline. These amino acids coil themselves in a characteristic repeating sequence of Gly-Pro-X, where X is the hydroxyproline, and make up the collagen triple helix .

The secondary structure of collagen is that of an alpha helix where collagen molecules are held in an extended conformation and stabilized by both intra-molecular and intermolecular forces. These stabilizing forces consist of hydrogen bonds, salt links, and covalent cross links. The highly ordered arrangement of collagen molecules in this extended conformation forms the structural basis and tensile strength of the densely organized collagenous tissue in tendons and ligaments .
1.6
Collagen’s response to thermal energy
Thermally induced conformational alterations of collagen have been well studied for its application in the field of orthopedic therapy . When tendril fibrils are exposed to temperature above 54°C, a steady decrease in signal intensity is demonstrated by second harmonic generation microscopy . This decrease in signal intensity is a sign of collagen denaturation.
Because collagen is abundant in many tissues and organs, including the mitral annulus, the potential for modification of collagen is quite relevant. It has been shown that collagen responds to heat by shrinking . The ability to shrink collagen through thermal or chemical methods has been known since at least 1871 . The degree of shrinkage of the collagen is dependant on the temperature and the duration of heat application. A temperature just below 70°C appears to be the threshold temperature at which the process of shortening and shrinking of collagen begins, while a temperature higher than 80°C leads to denaturation and destruction of the collagen . The effect of time or duration of thermal exposure is demonstrated by the fact that, for a given temperature, there is an initial slow change, followed by an increase in shrinkage rate, then the shrinkage reaches a plateau, giving a sigmoidal shape to the curve of shrinkage versus time . When bovine knee capsule was placed in saline solution at temperatures ranging from 55°C to 75°C, for variable durations, the tissue shrinkage was first noticed at 60°C and reached its plateau, with 50% shrinkage, at temperature above 65°C .
Beyond certain critical level of shrinkage, detrimental material and structural alterations can occur. For example, as tendons shrink, their cross-sectional area increases and tensile strength decreases . With progressive shrinkage, the tissue reaches a point where it elongates beyond the tendon’s normal length, as demonstrated by plotting the stress versus stretch ratio . The ideal level of thermal energy is a one that balances the magnitude of the insult against the possibility of irreversible collagen damage and provides maximal shrinkage with minimal negative effects on the mechanical and tensile strength of the tissue. Microscopically, the changes noted in shrinking collagen consist of conformational change of collagen from the highly ordered crystalline arrangement to random coil contracted state. It is believed that heat causes rupture of molecular cross-links responsible for stabilizing collagen. Transmission electron microscopy shows that heat causes an increase in collagen fibrils’ cross-sectional area. The fibrils maintain the circular shape but they lose their distinct margin edges. Loss of periodical cross striation was also seen using this technology. These ultrastructural changes are thought to be the result of unwinding of the collagen triple helix upon tissue exposure to heat . Both global and local mechanisms were shown to contribute to the denaturation of type I collagen in rat tail tendons .
1.7
Thermal Modification of Collagen and the Rationale for Treatment of MR
Several medical treatments such as laser modification of corneal curvature, orthopedic, and dermatological procedures used the concept of thermal modification of collagen. Lyons et al., showed that laser-assisted capsulorrhaphy was an effective treatment alternative for shoulder instability . Heat-induced collagen shrinkage was used to rejuvenate the skin by tightening loose skin and folds .
Mitral valve repair methods, whether surgical or percutaneous, aim at improving the coaptation of the mitral leaflets either by reducing the circumference of the mitral leaflets annulus or by displacing the posterior annulus anteriorly closer to the anterior leaflet. Since the mitral annulus is rich with dense collagen, it would be logical to target this tissue with treatment modalities affecting collagen properties. As mentioned above, thermal energy could be applied to a specific tissue, in this case the mitral annulus, in the form of RFE hoping to shrink the annulus and to decrease the regurgitant orifice. This potential therapeutic modality was first explored by Heuser et al., in vivo on an ovine model, using the QuantumCor probe (QuantumCor, Lake Forest, CA, USA) . The QuantumCor is a malleable probe that incorporates seven electrodes (∼1.5×2 mm) and conforms to the annulus shape ( Fig. 4 ). The probe could be inserted to the mitral annulus location and RFE is delivered and controlled by a computer to a maximum temperature sensed by adjacent thermocouples.

In this animal experiment, Heuser et al., reported the effect of RFE on 16 healthy Suffolk mix sheep among which six had naturally occurring MR. The sheep were properly prepped and sedated then intubated and hemodynamically and electrocardiographically monitored. Intracardiac echocardiography (ICE) was used to guide the procedure and to assess outcome and was introduced via an intravenous catheter. Through open thoracotomy, and under cardiopulmonary bypass, the mitral annulus was accessed through an incision in the left atrial appendage. The QuantumCor probe was inserted and, under computer control with thermocouples feedback, RFE was delivered providing a temperature of 65°C. The RFE heat was applied to annuli circumferentially starting from the posterior annulus. The entire posterior annulus was treated from commissure to commissure in 1–2-cm segments applying RFE for 50–60 s per treatment site with some overlaps in treatment areas . Dimension changes were computed by analysis of images obtained with a 7.5-MHz Acuson AcuNav diagnostic ICE catheter, used with the Acuson Cypress or Sequoia Echocardiography System (Siemens Medical solutions, Mountain View, CA, USA). Changes in the septal/lateral diameter of the mitral annulus were measured. The presence of MR pre- and posttreatment was assessed by color Doppler flow. Two “sham” or control sheep underwent the same surgical procedure including positioning of the RFE probe on the mitral annulus but without energy application.
Nine sheep were sacrificed for evaluation of acute results and seven were recovered and sent for pasture for 30 days. The nine acute animals were sacrificed and the treated annuli were resected and sent for histopathology analysis. The seven surviving sheep, and the two control sheep, were assigned to be followed for 30-, 60-, 90-, and 180-day interval and histopathology (HP) exam was to be performed at 180 days in order to assess the long-term efficacy and durability of the annular shrinkage following the procedure . Nine animals (including the 2 control) survived until the 90-day point, but 3 from the treatment group did not make it to the 180-day mark. One died from sepsis, another from presumed hypertensive disease and the third from possible complications from anesthesia during ICE; no death was directly associated with the procedure itself. The two control animals were sacrificed at 90 days and sent for necropsy.
The mitral annuli dimensions of the surviving animals were measured at the 30-, 60-, 90-, and 180-day using the same equipments . Histology samples were uniformly taken from the mid-segment at each treatment site so that single treatment results could be evaluated. Overlapping sites were not studied histologically because of the nonuniformity of treatment times and temperature in these areas.
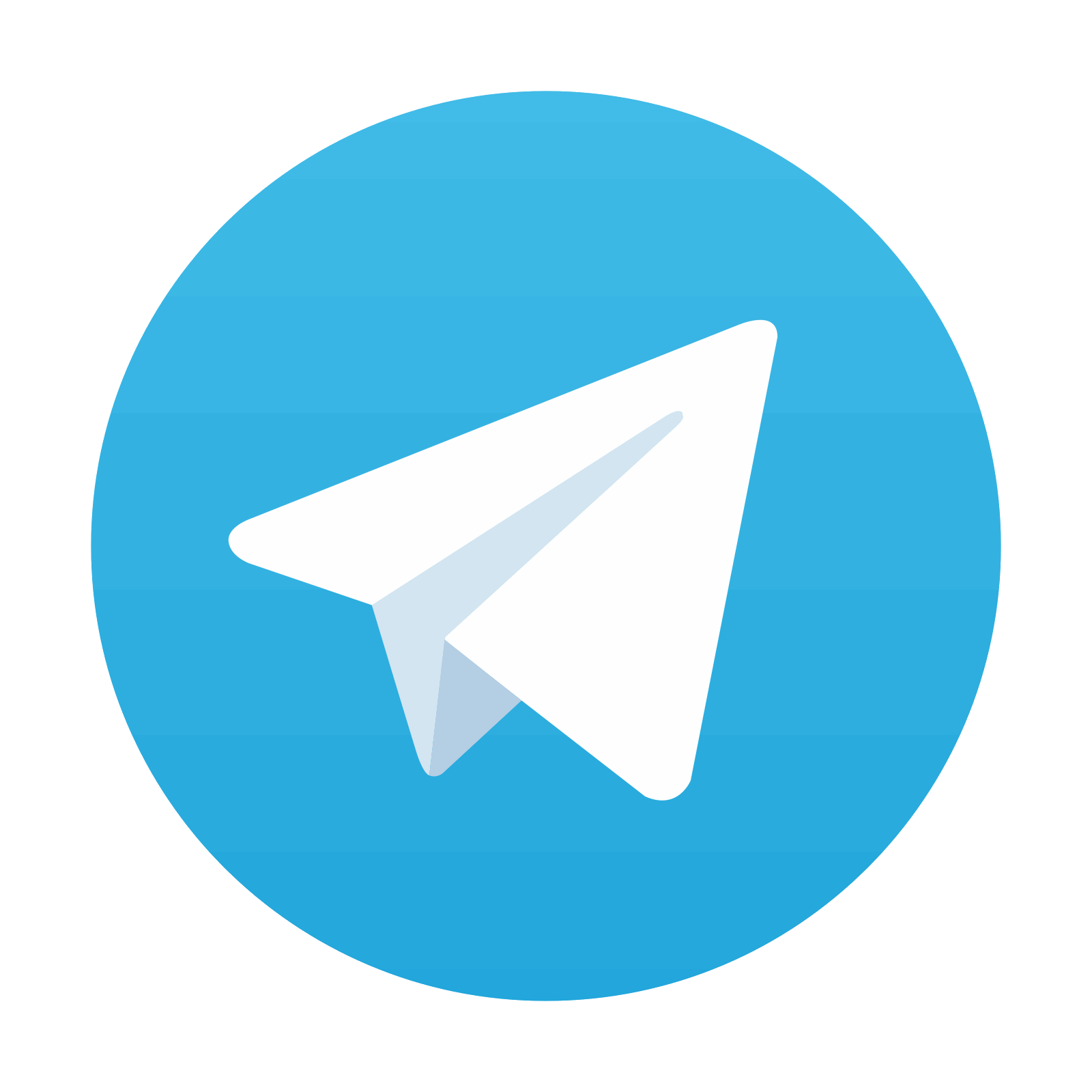
Stay updated, free articles. Join our Telegram channel

Full access? Get Clinical Tree
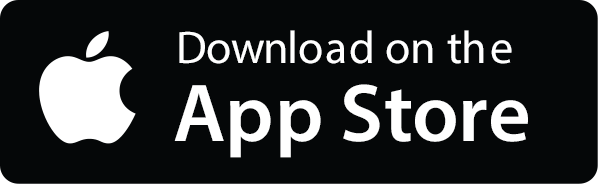
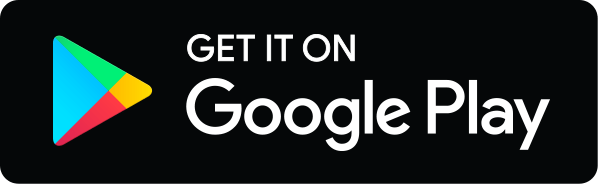